REDOX FACTS Reductionoxidation redox couple pair of molecules
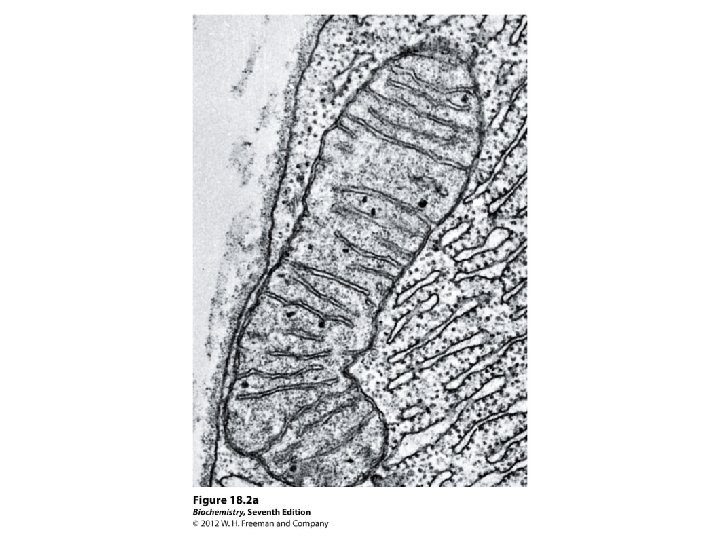
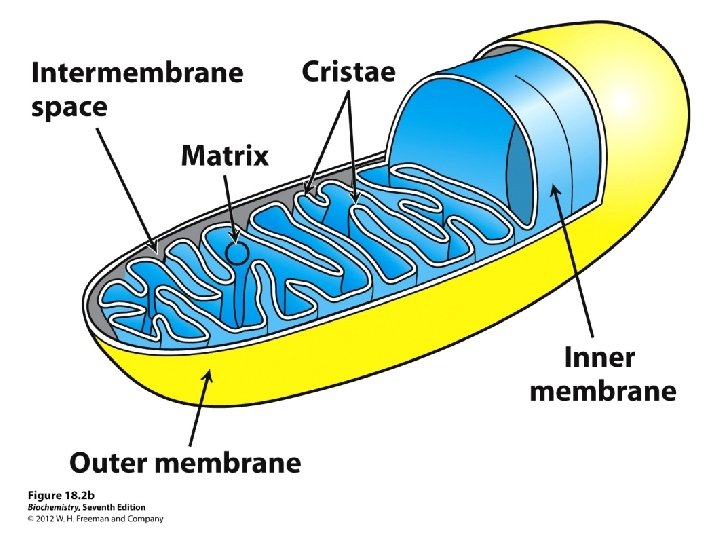
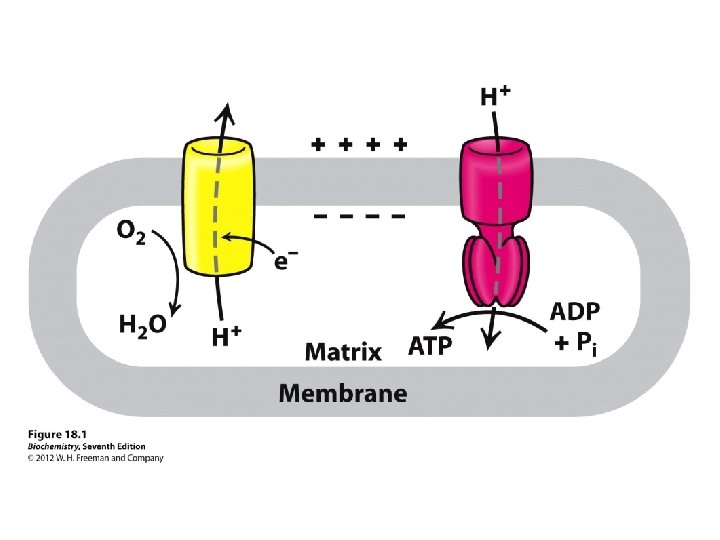
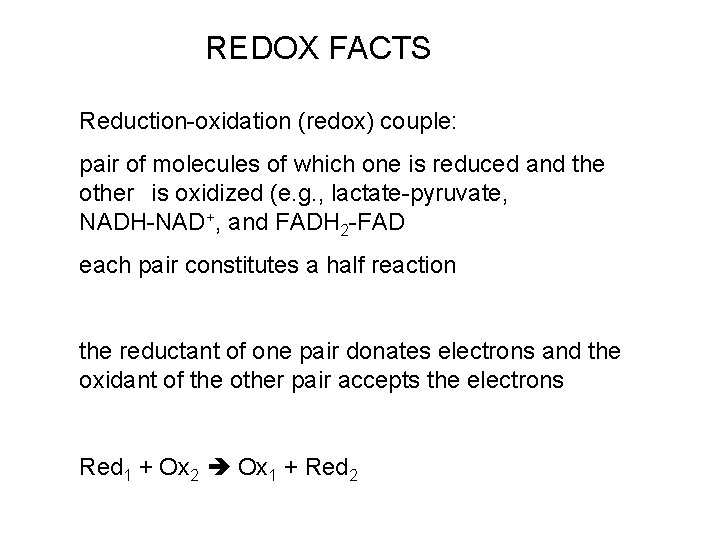
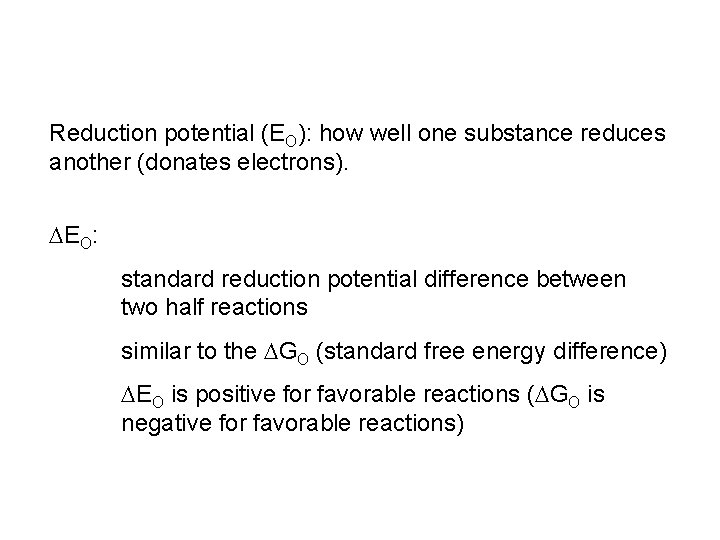
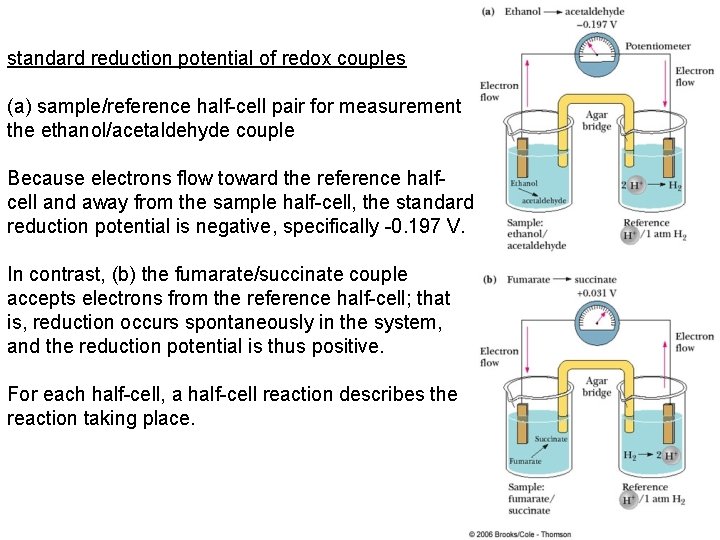
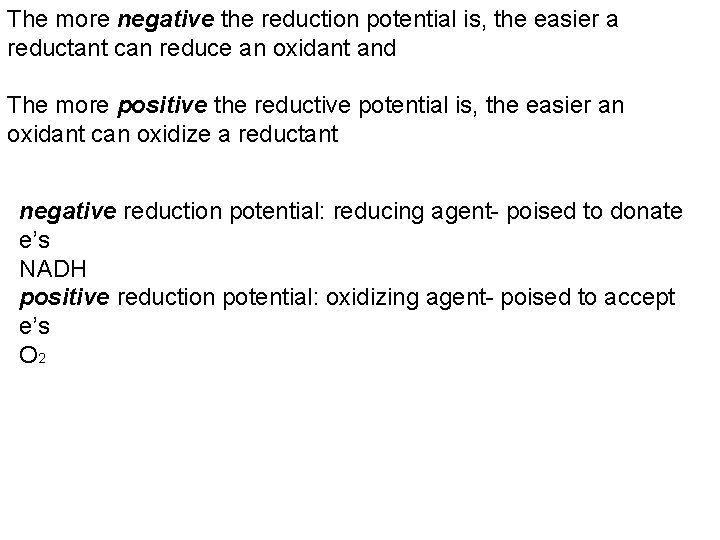
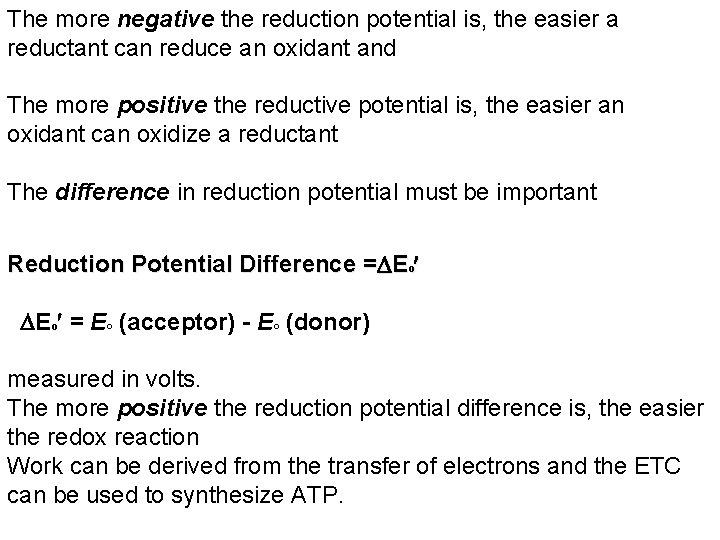
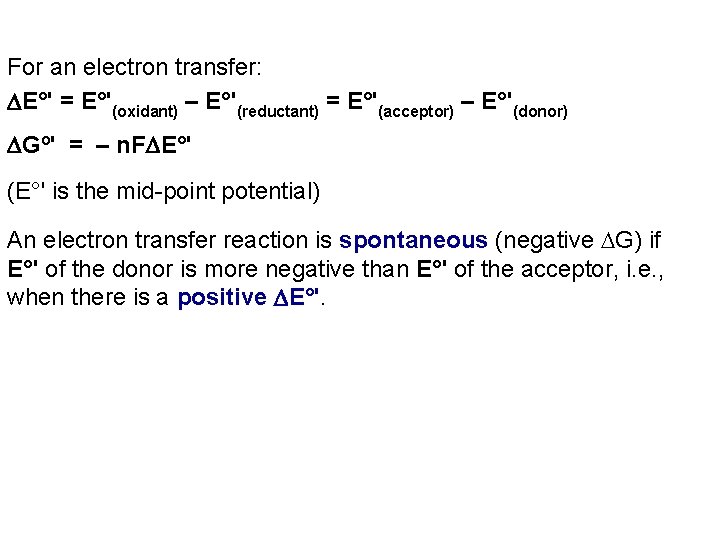
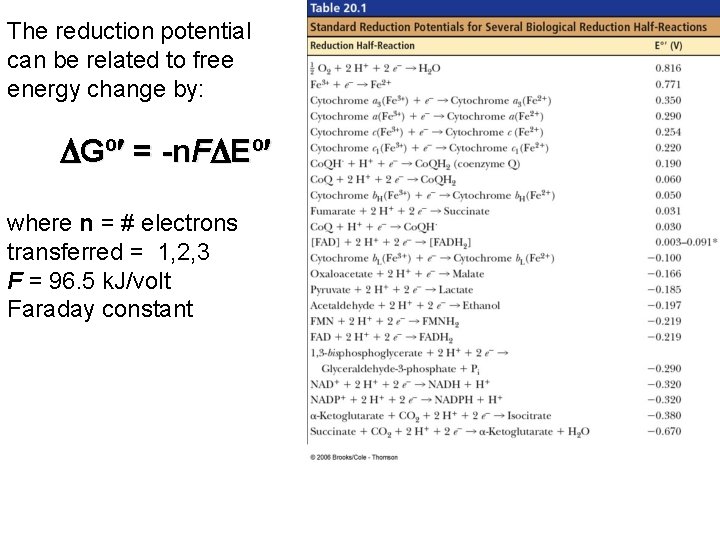
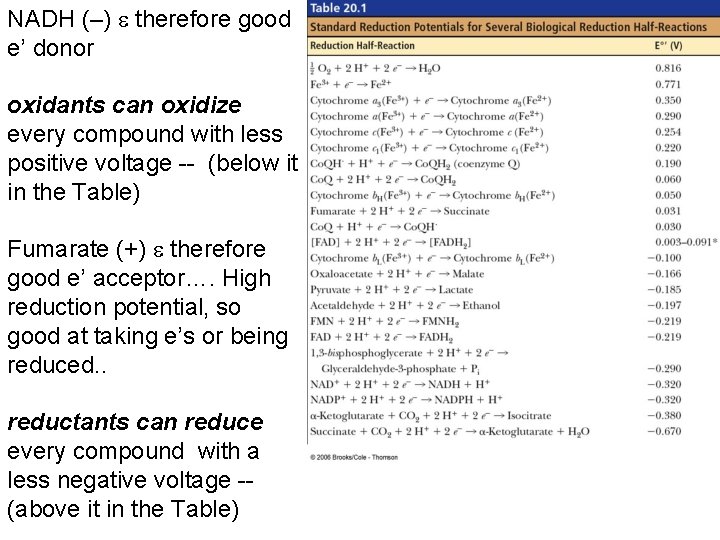
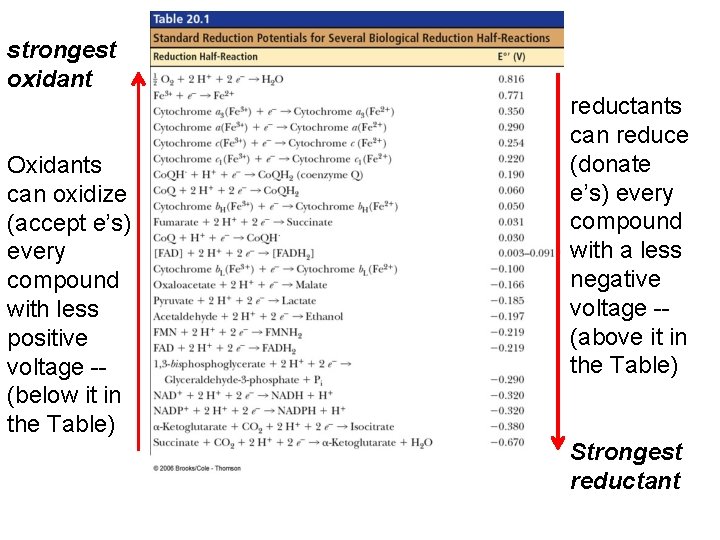
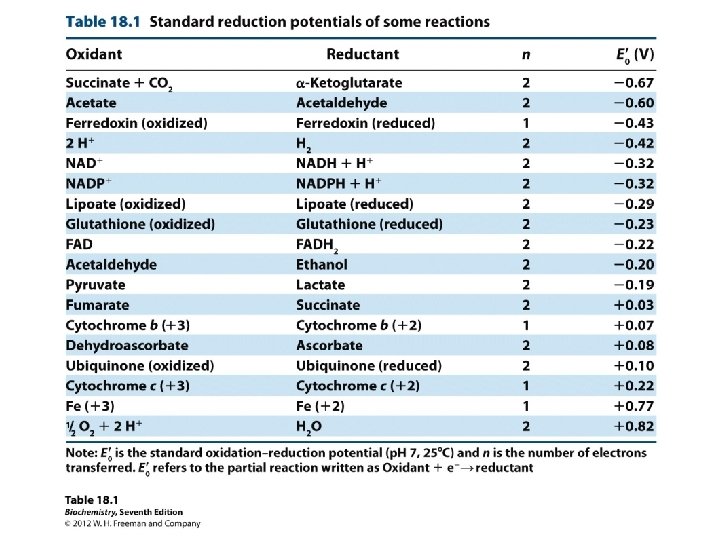
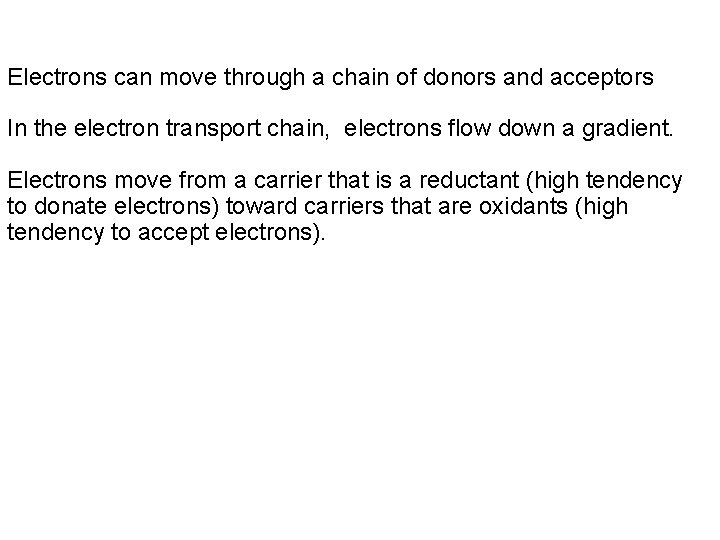
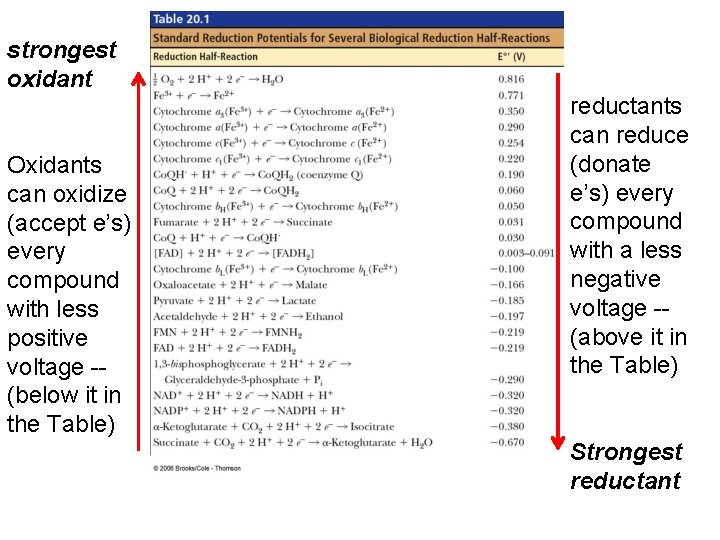
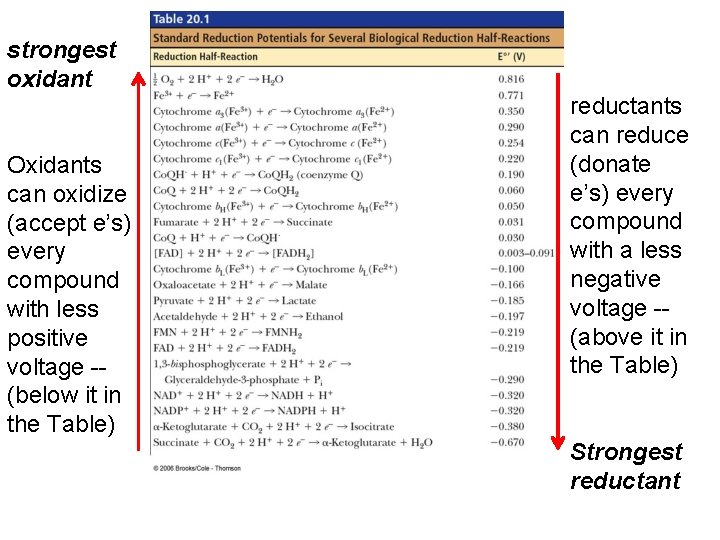
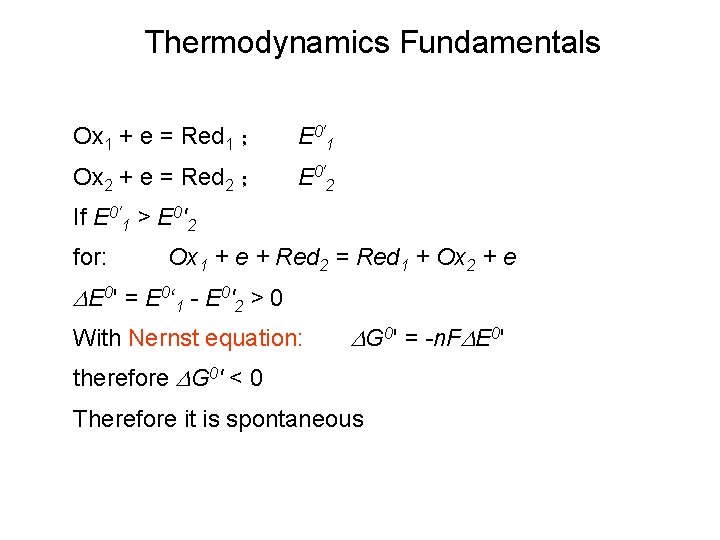
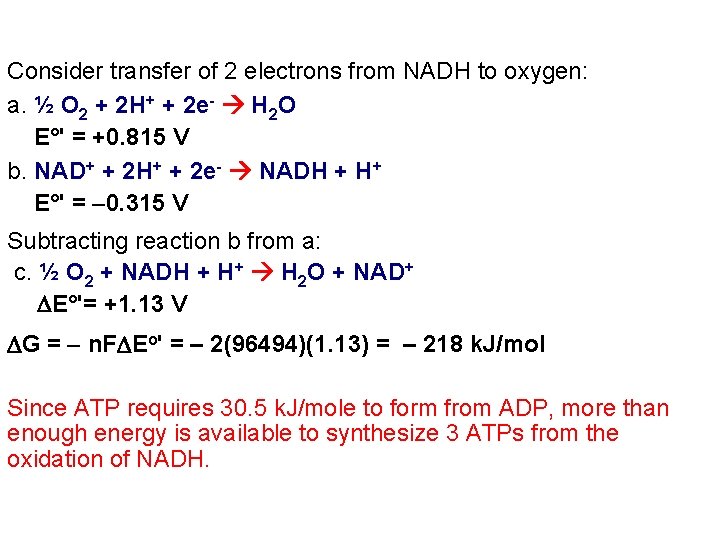
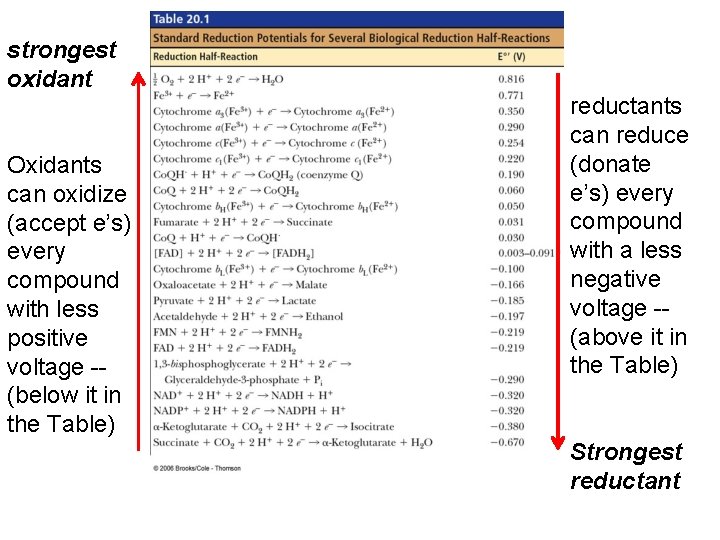
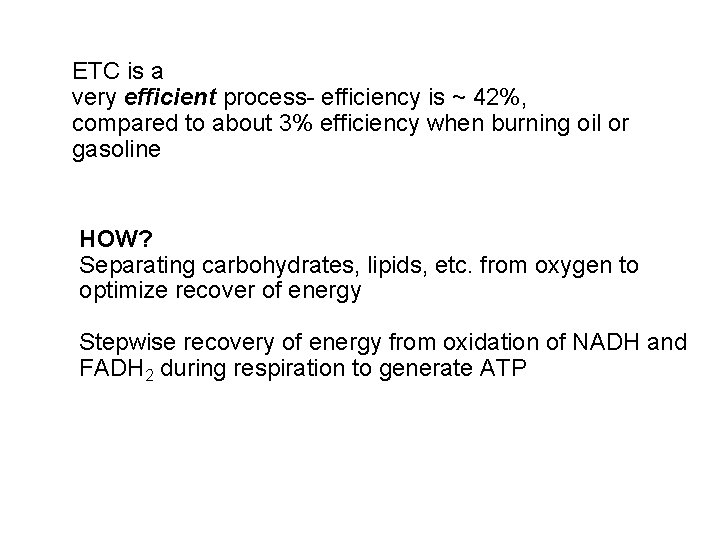
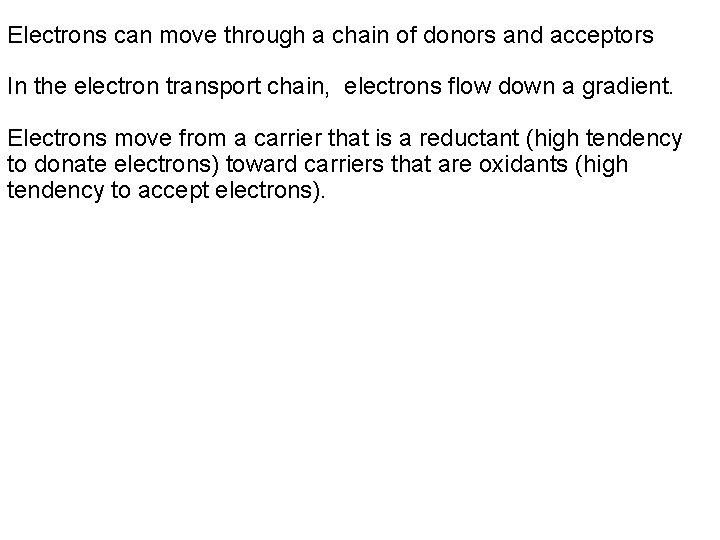
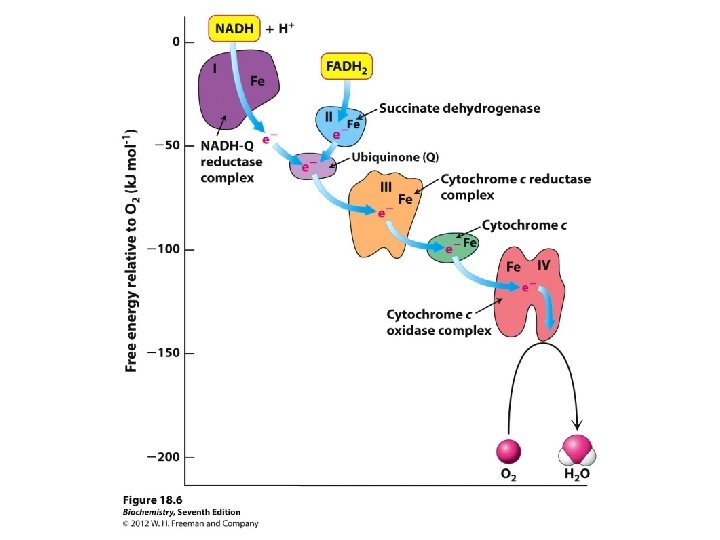
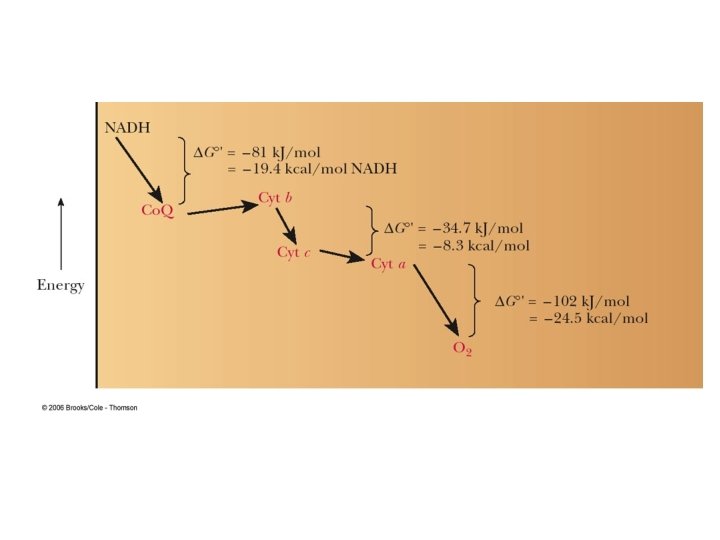
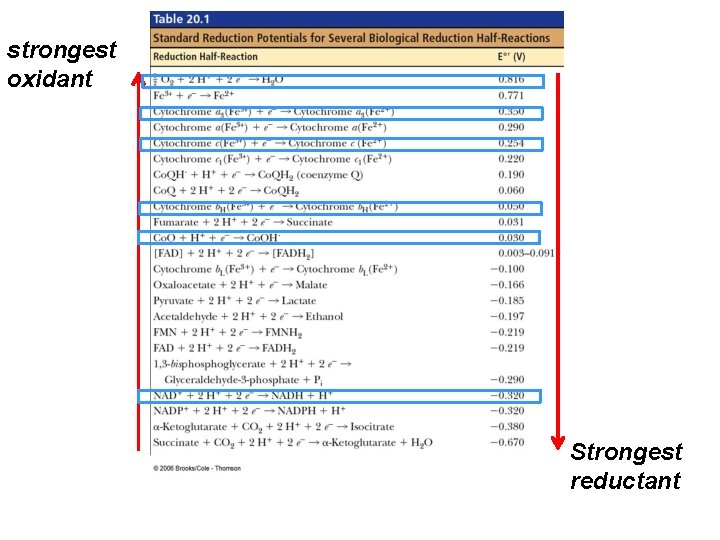
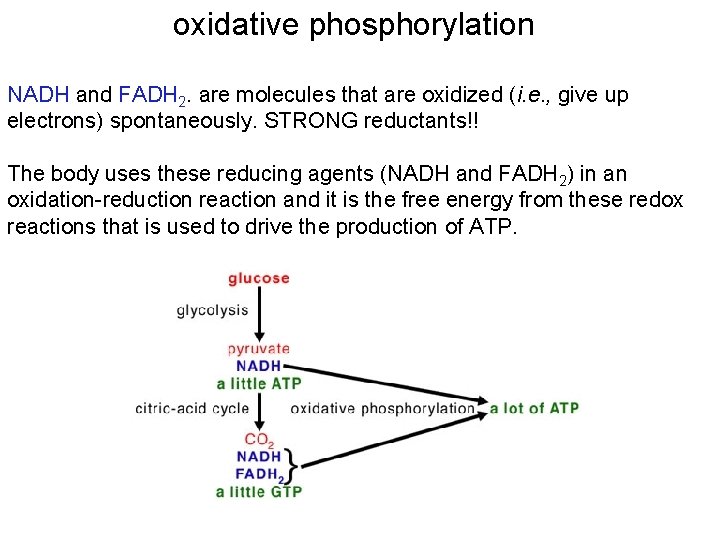
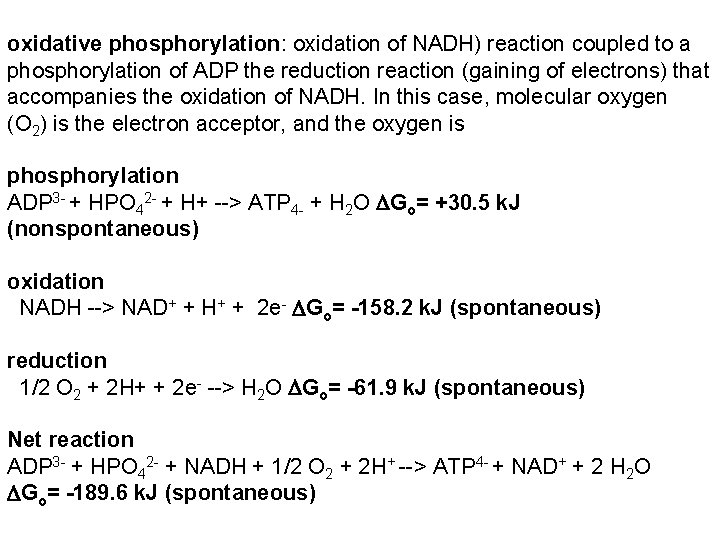
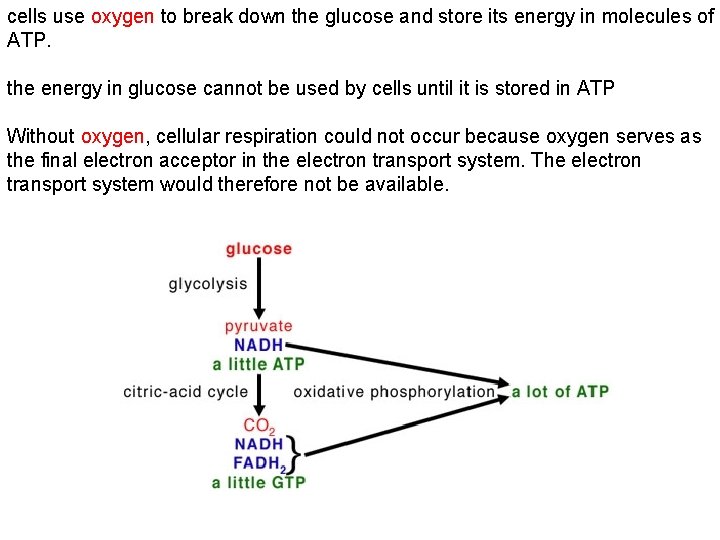
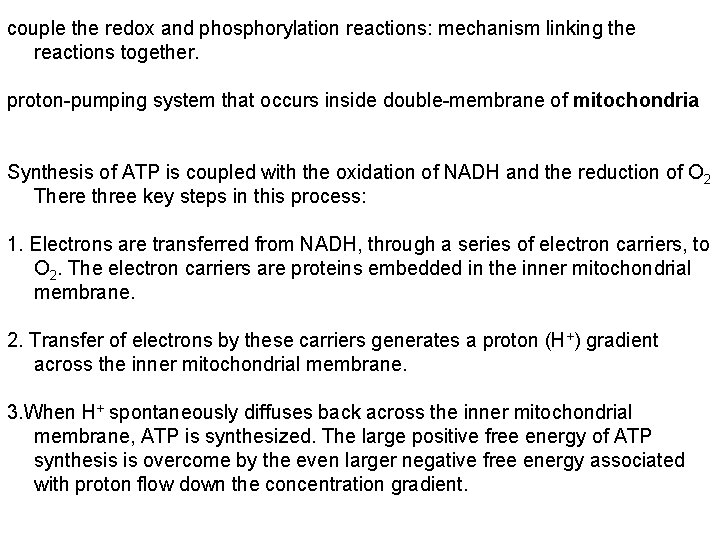
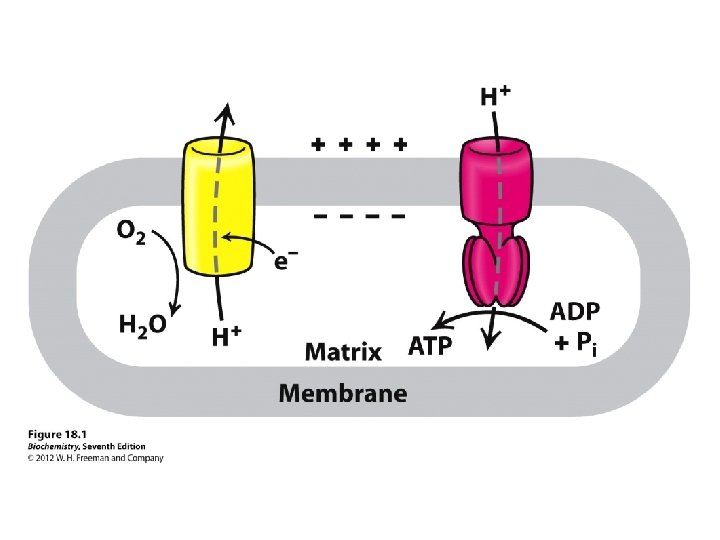
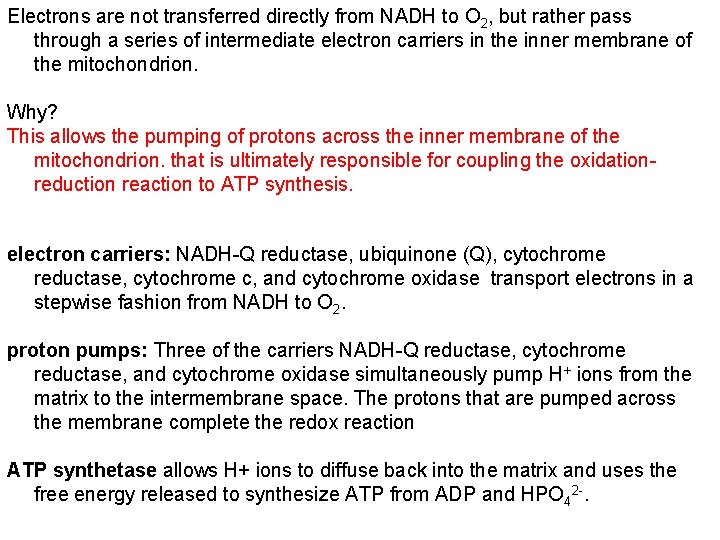
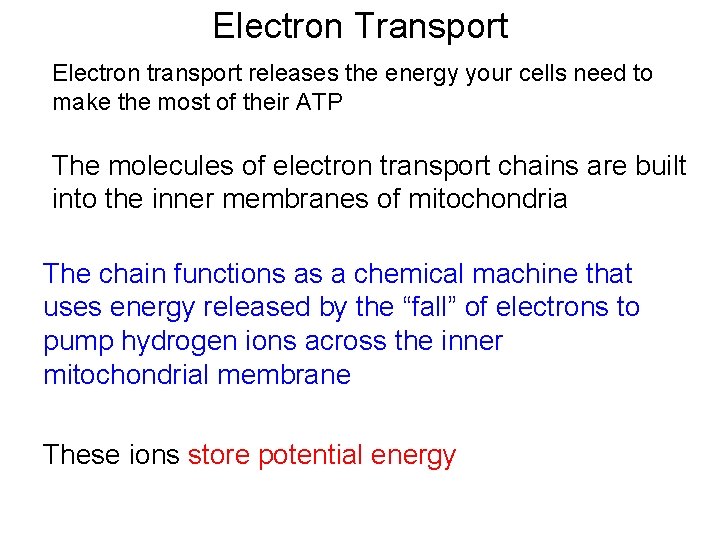
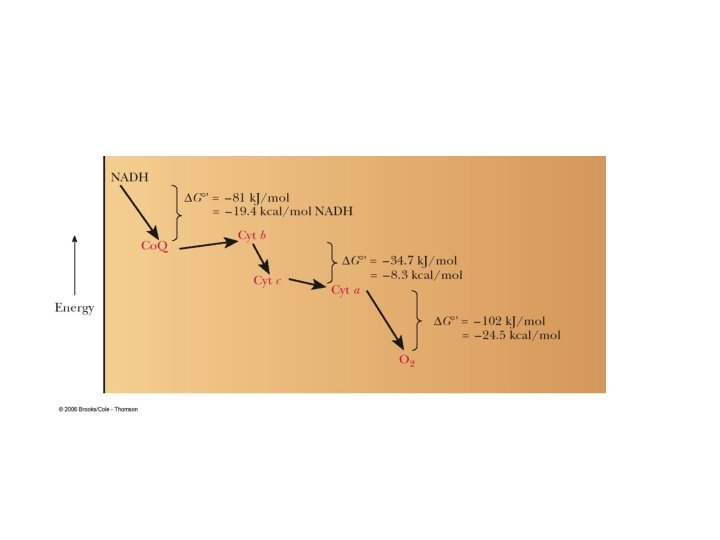
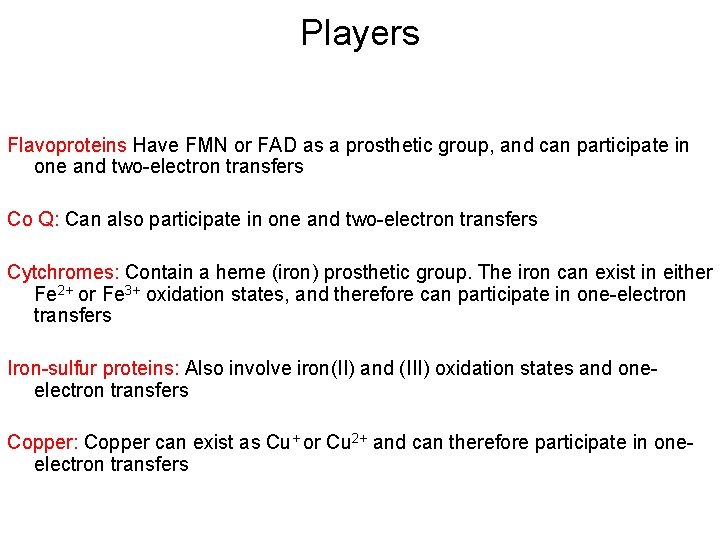
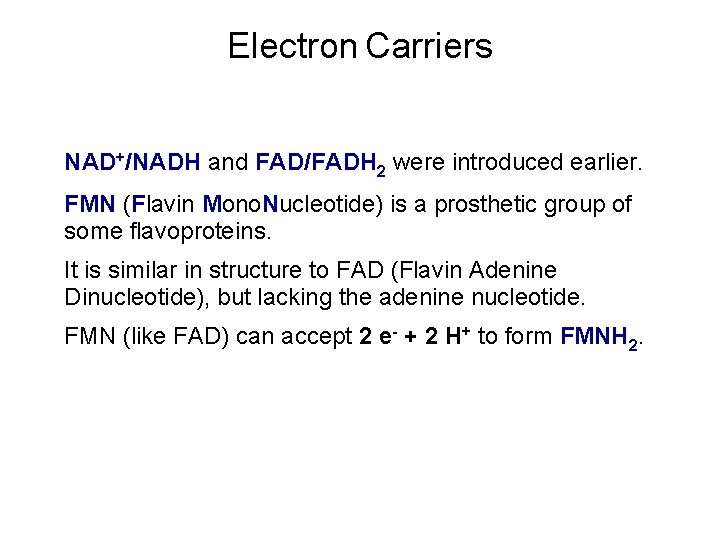
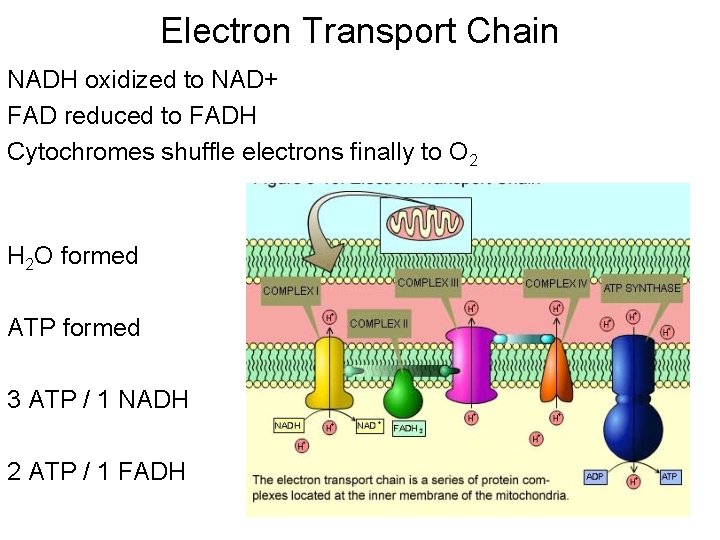
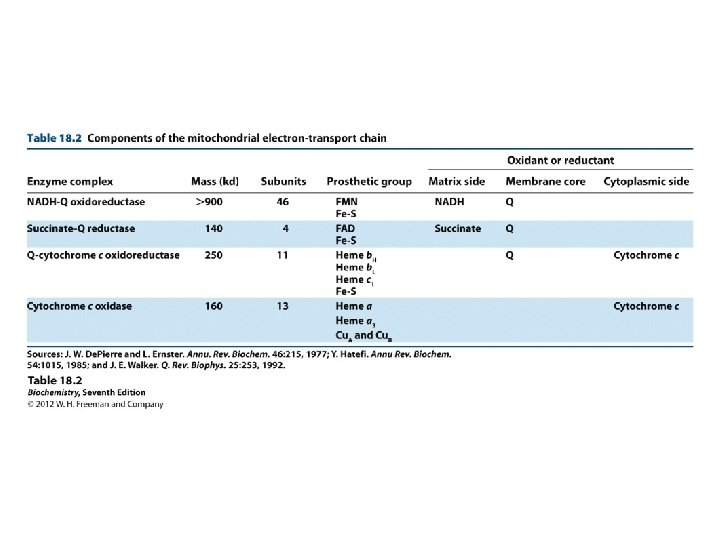
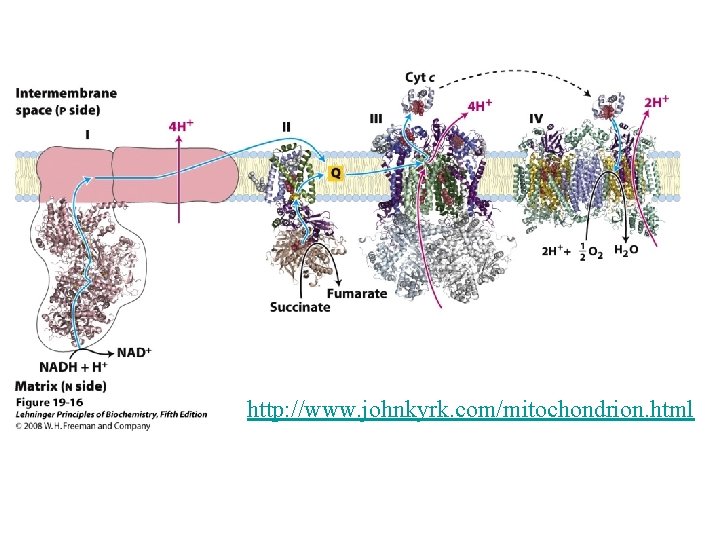
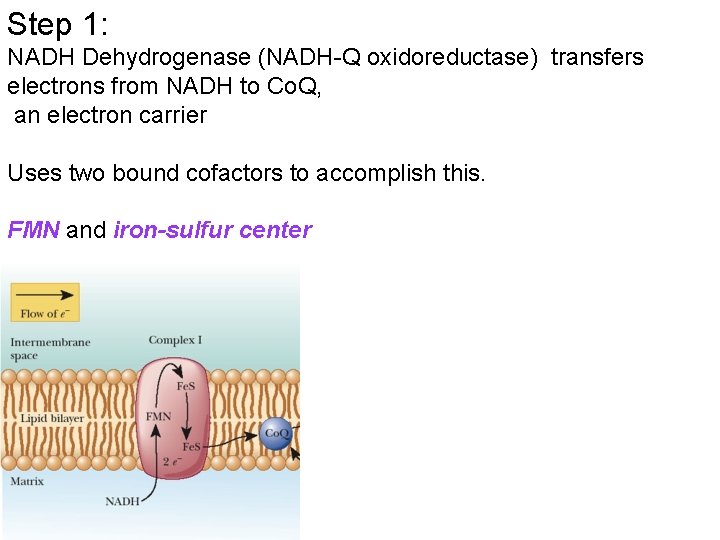
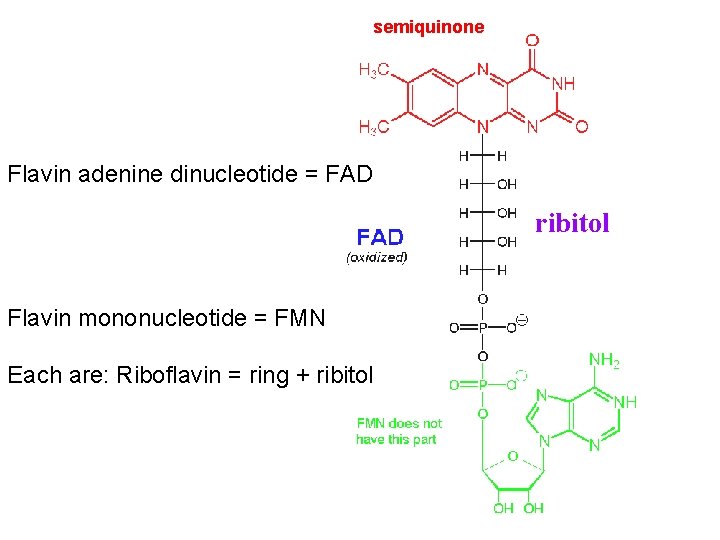
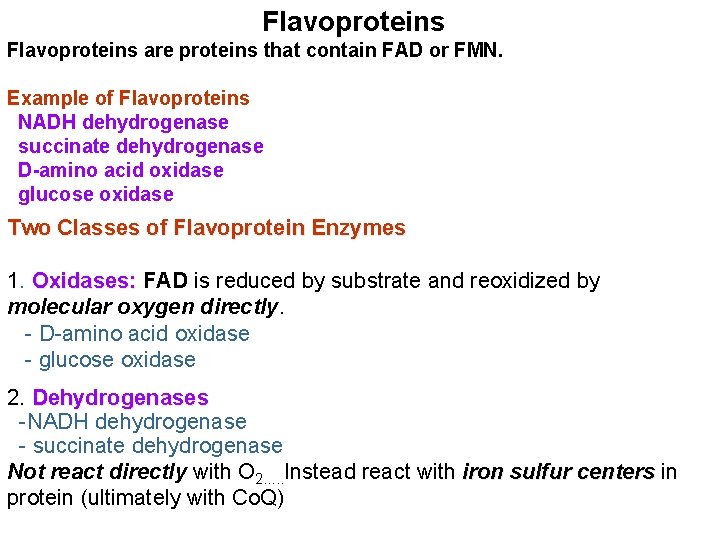
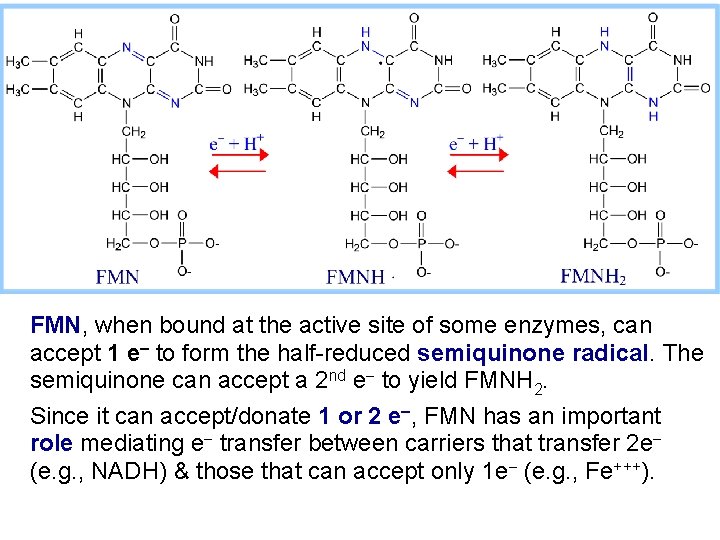
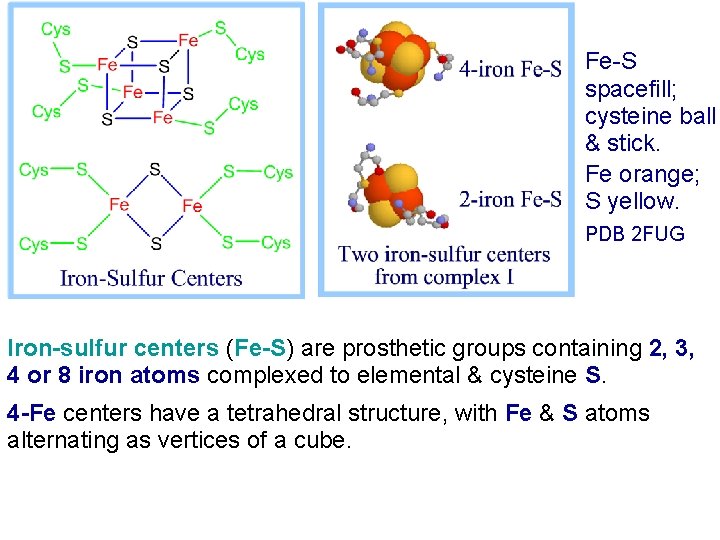
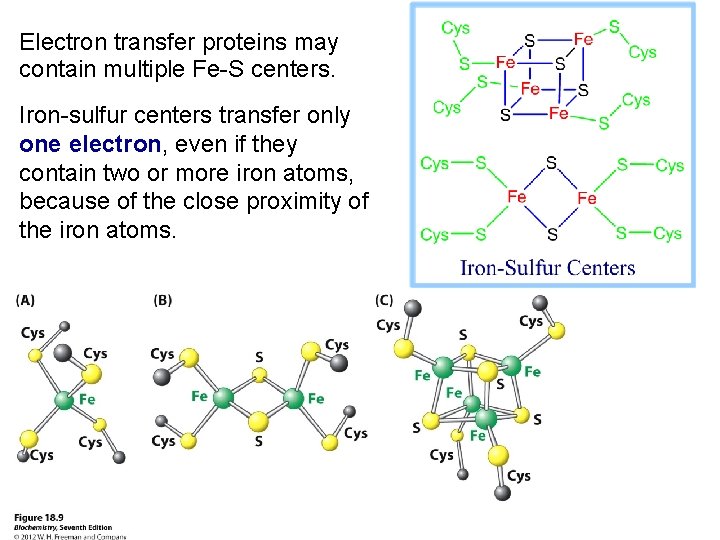
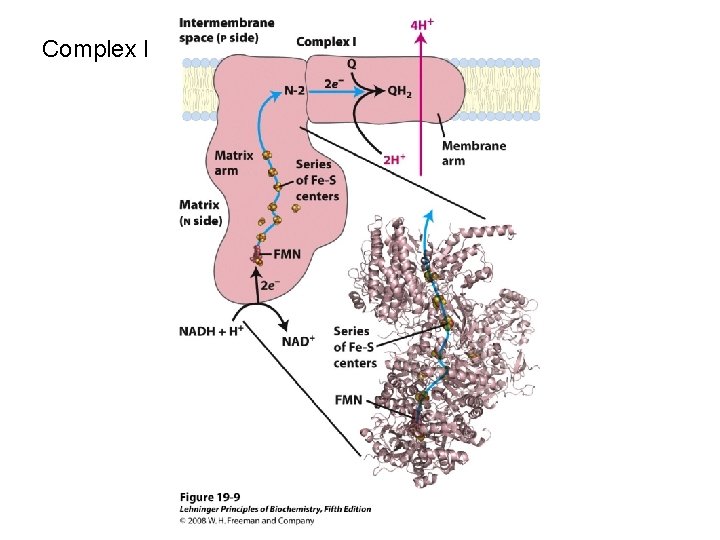
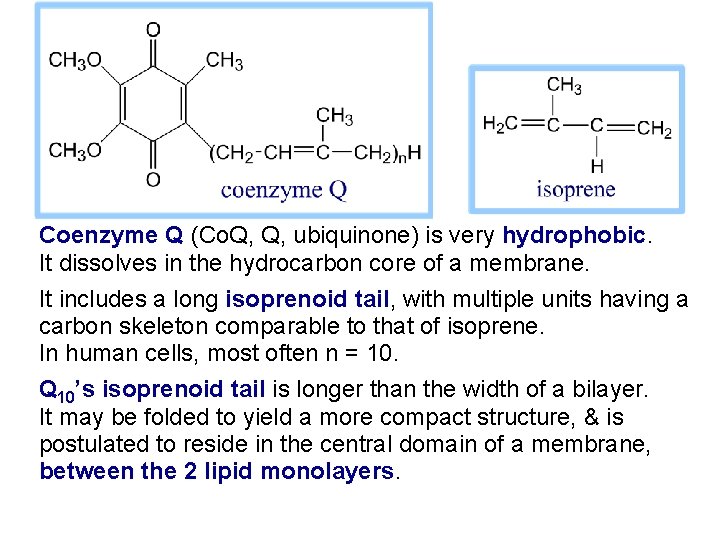
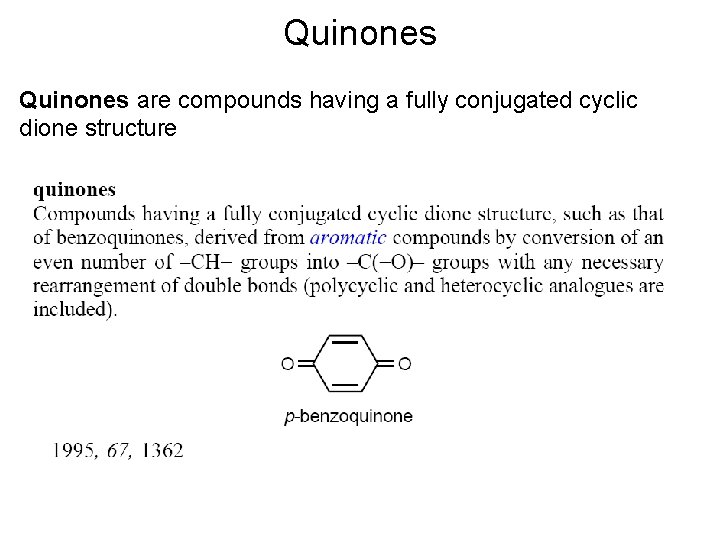
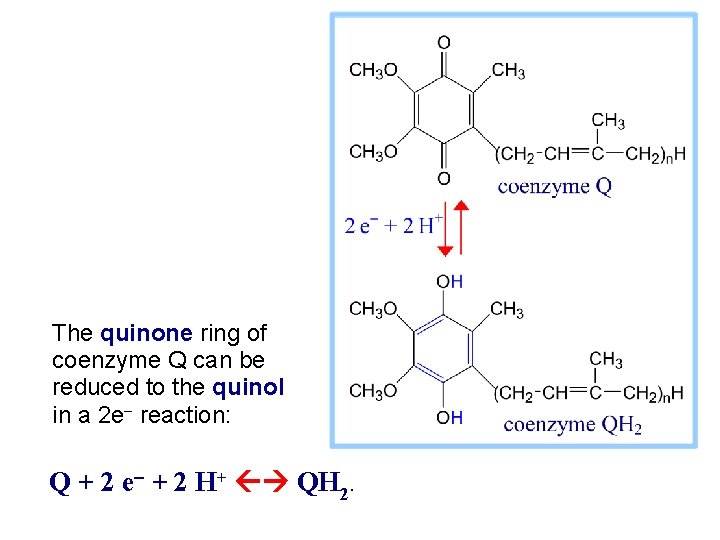
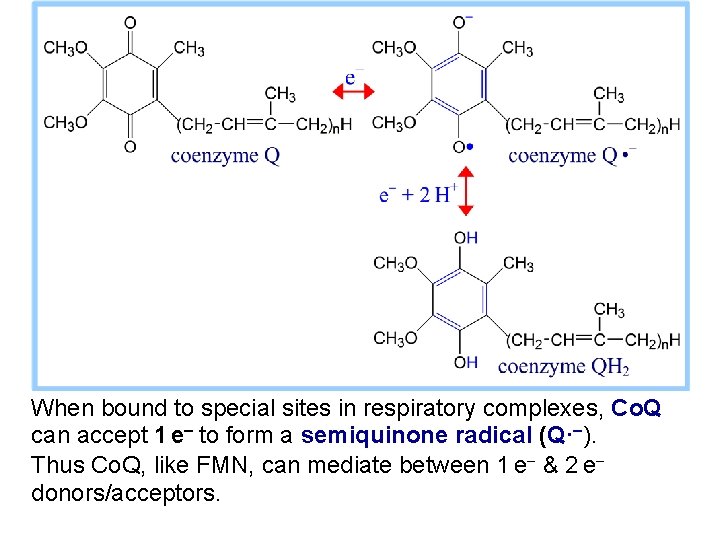
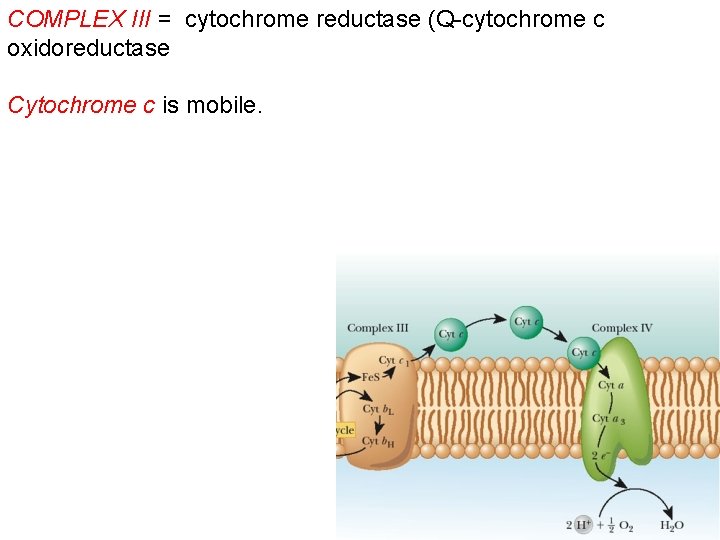
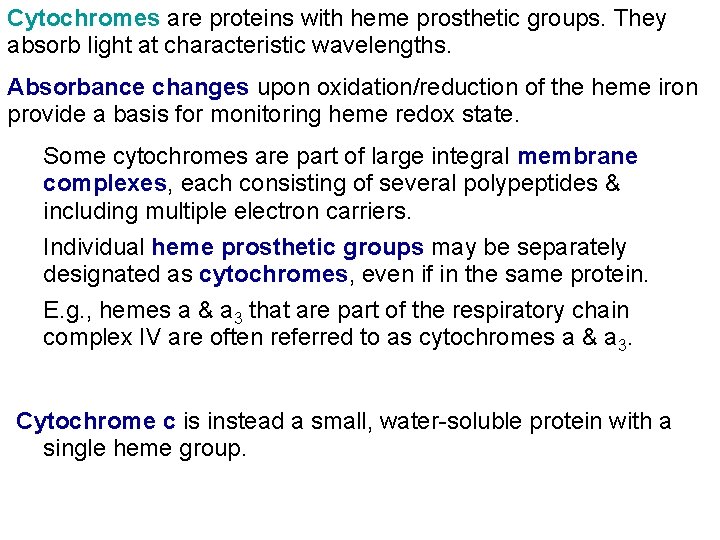
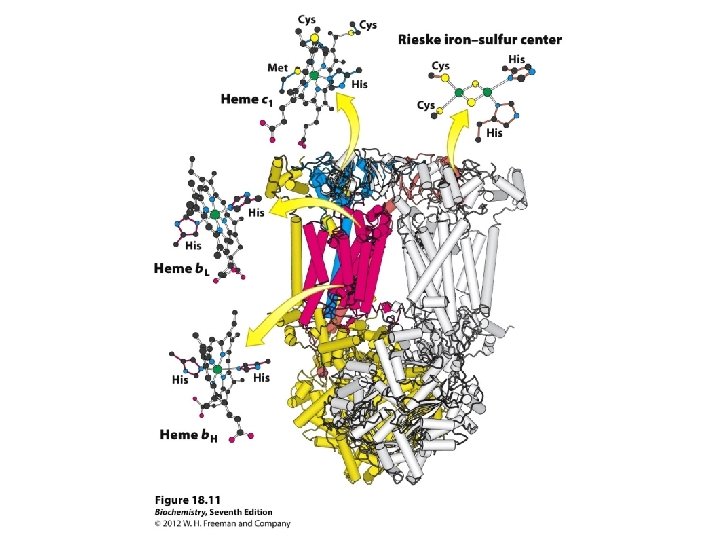
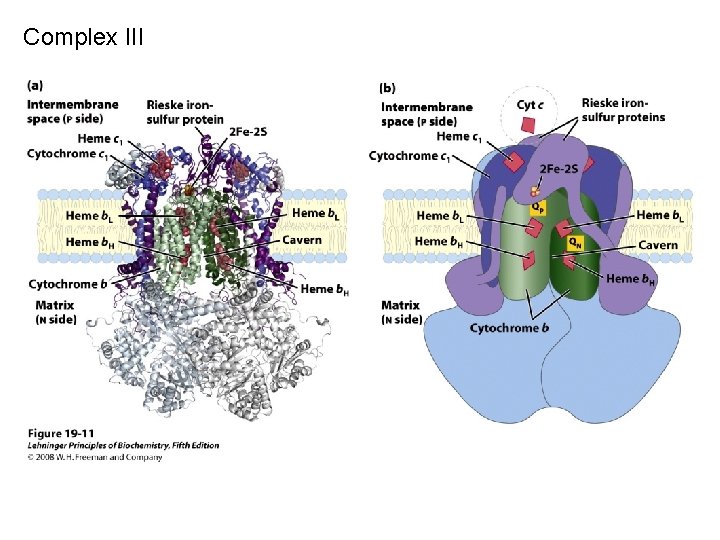
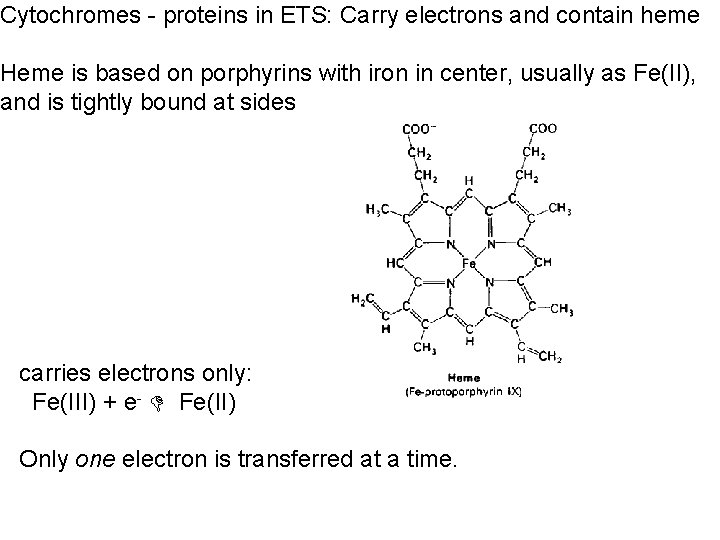
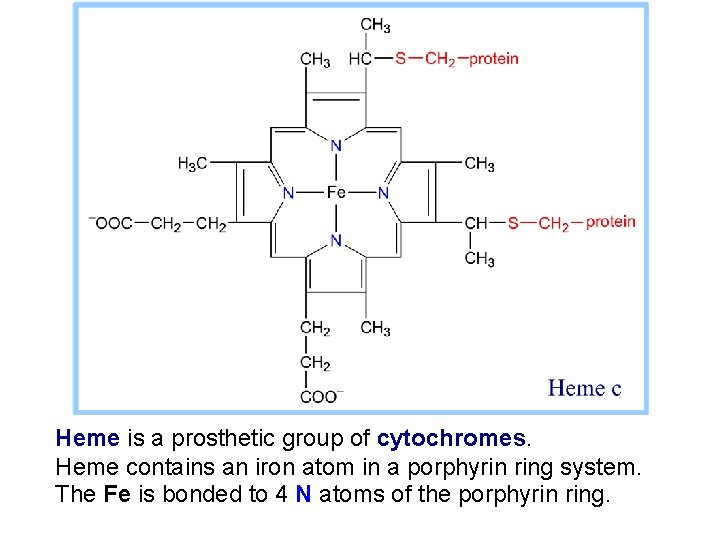
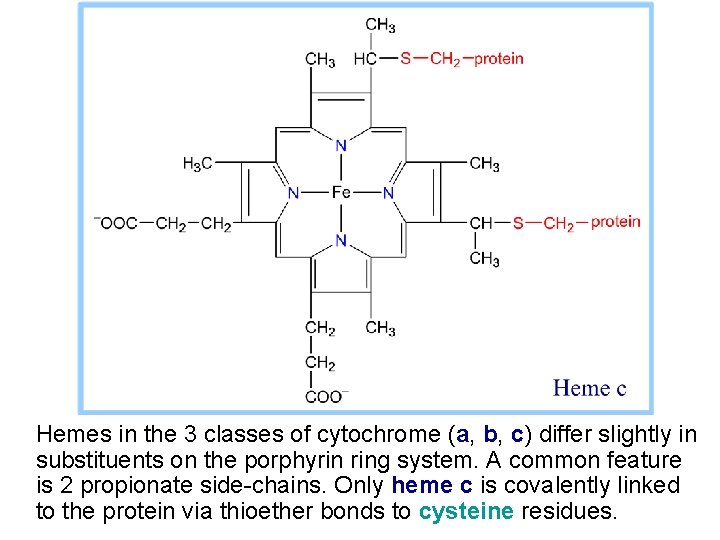
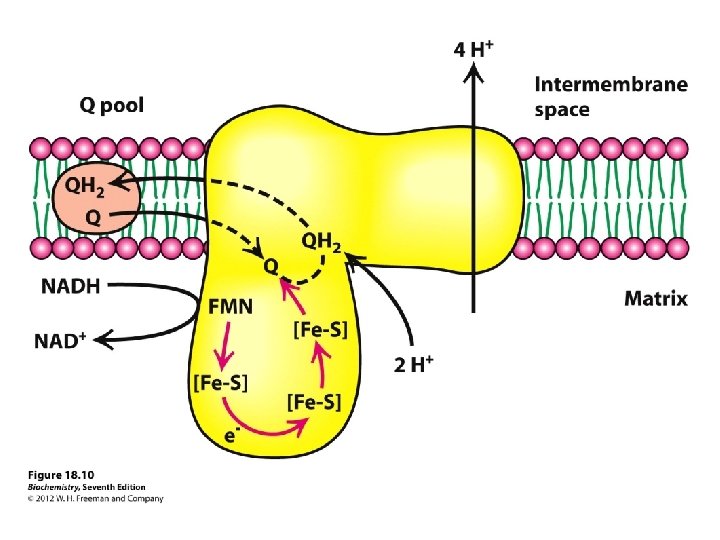
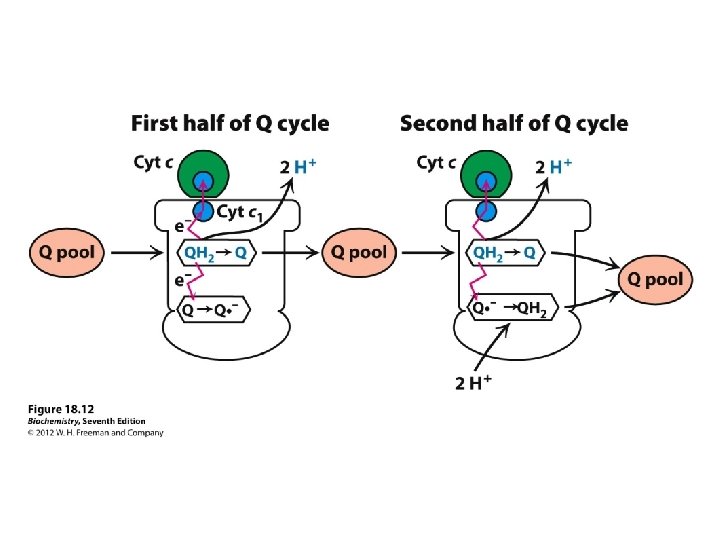
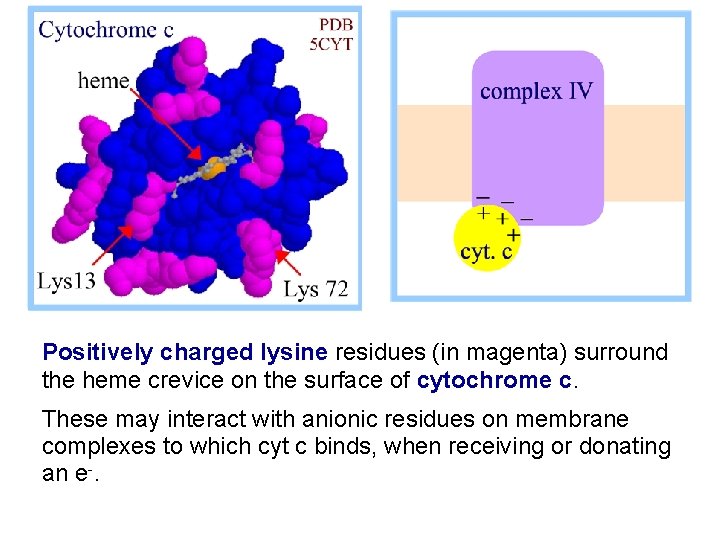
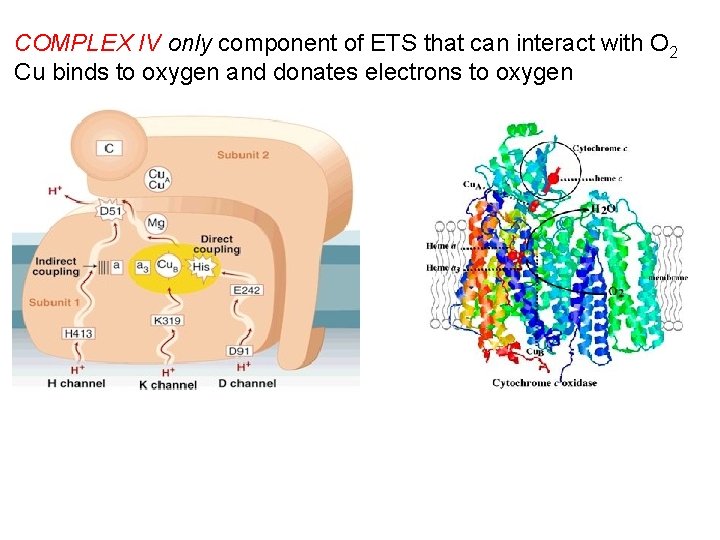
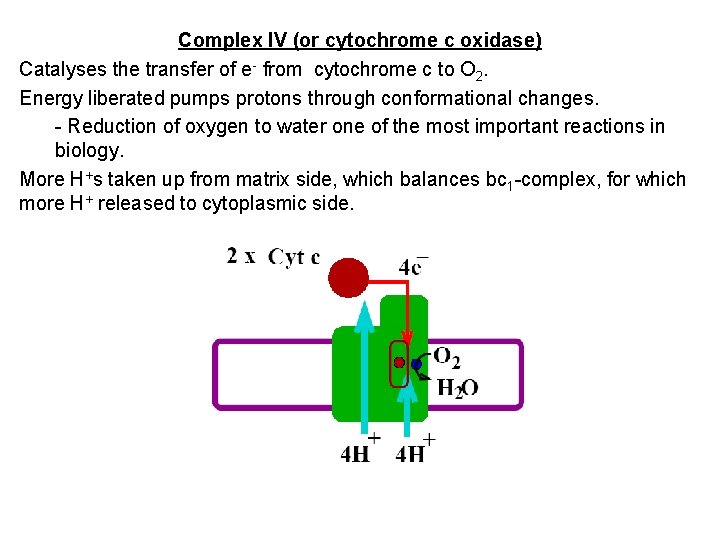
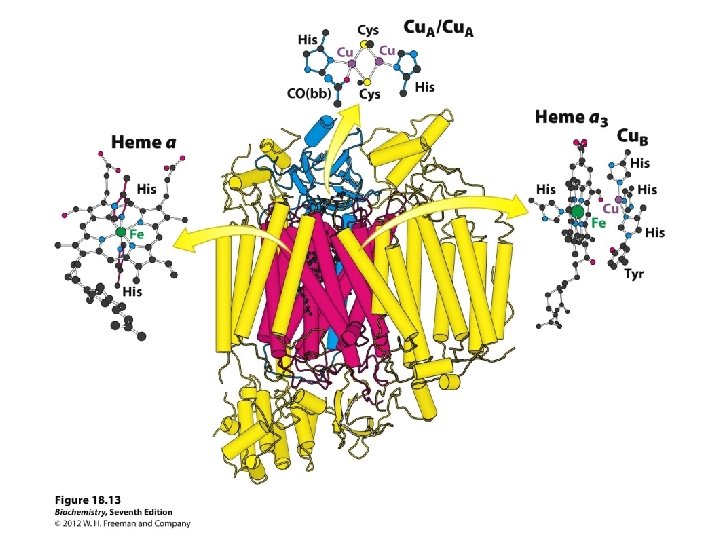
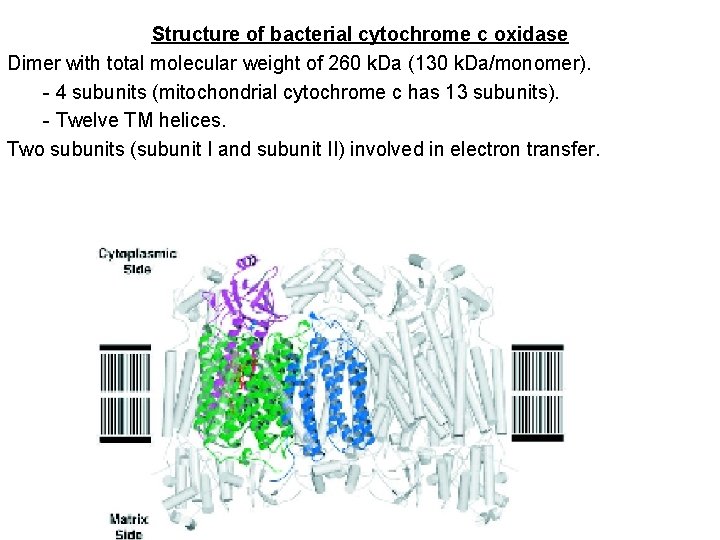
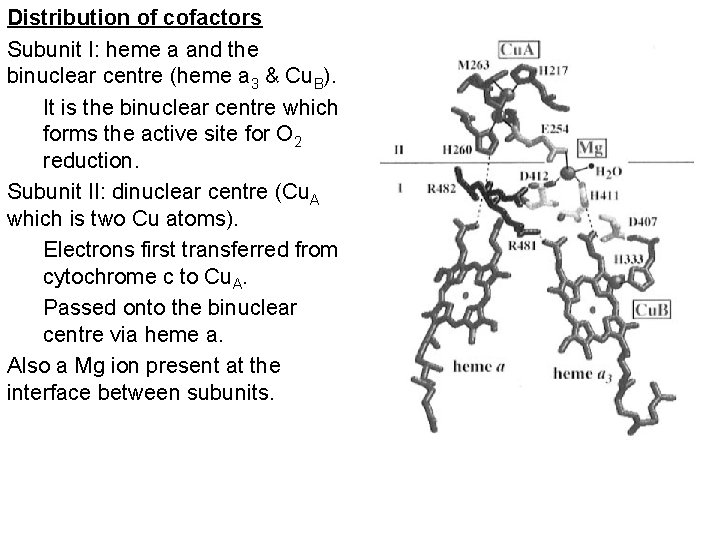
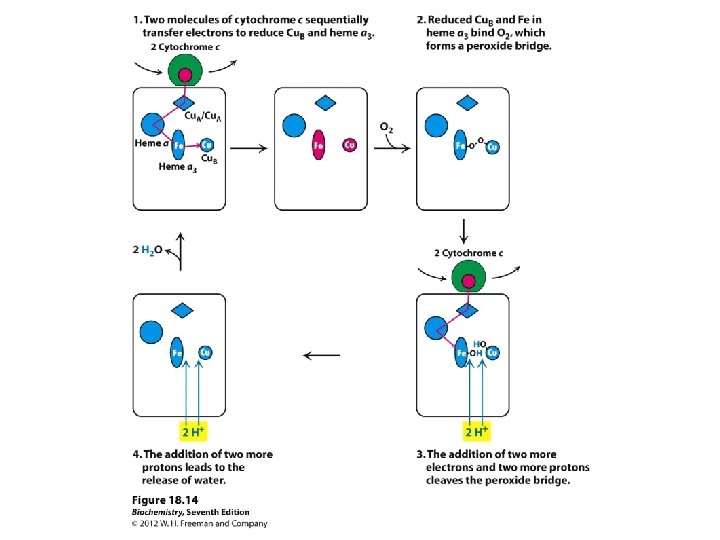
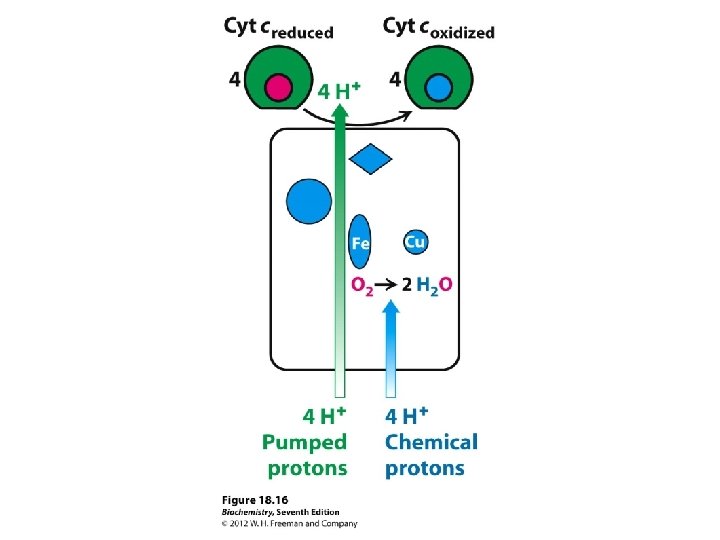
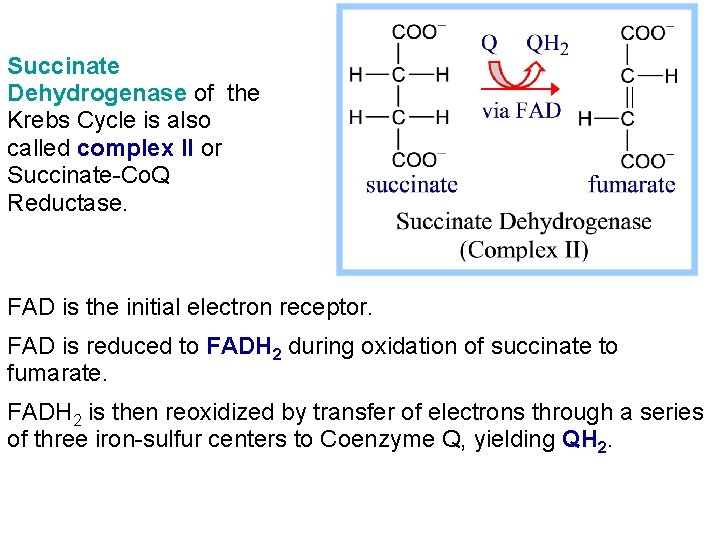
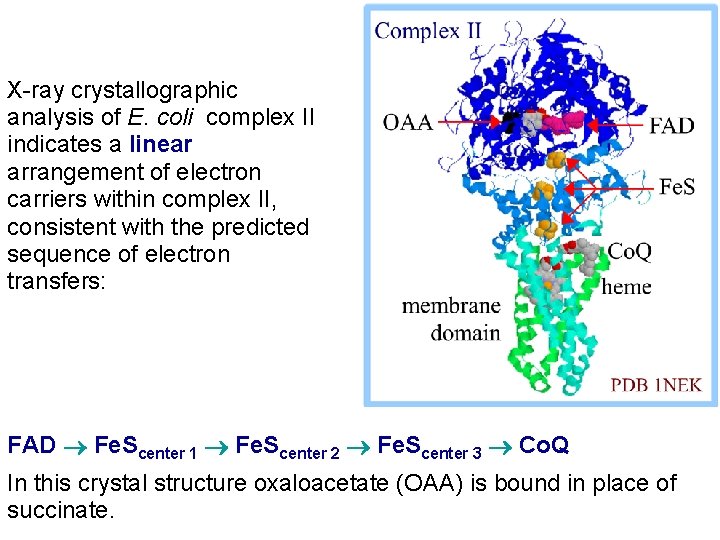
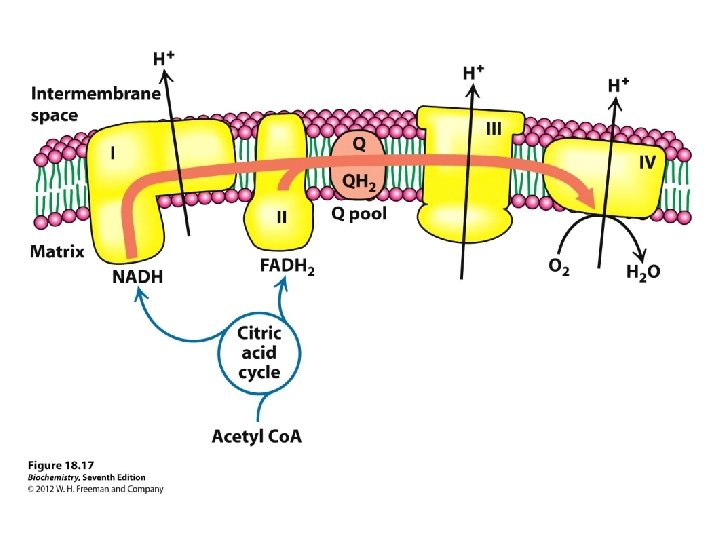
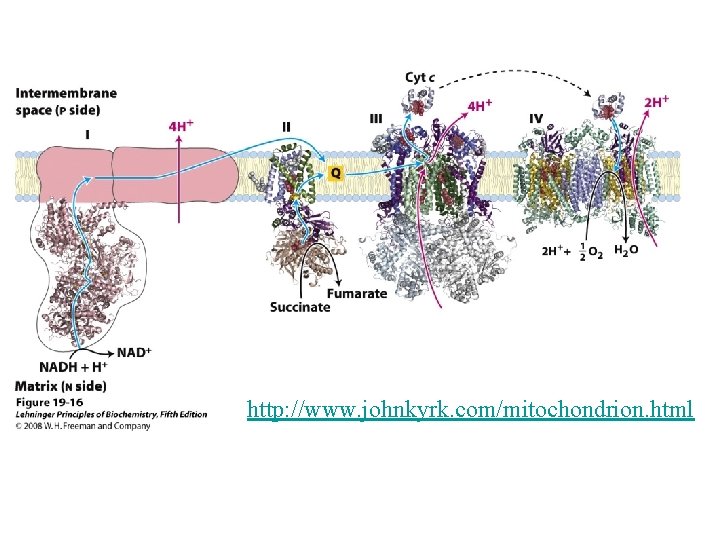
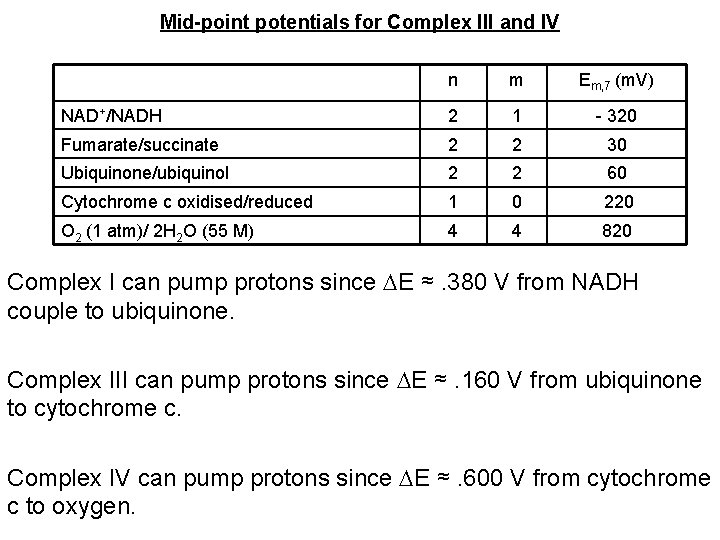
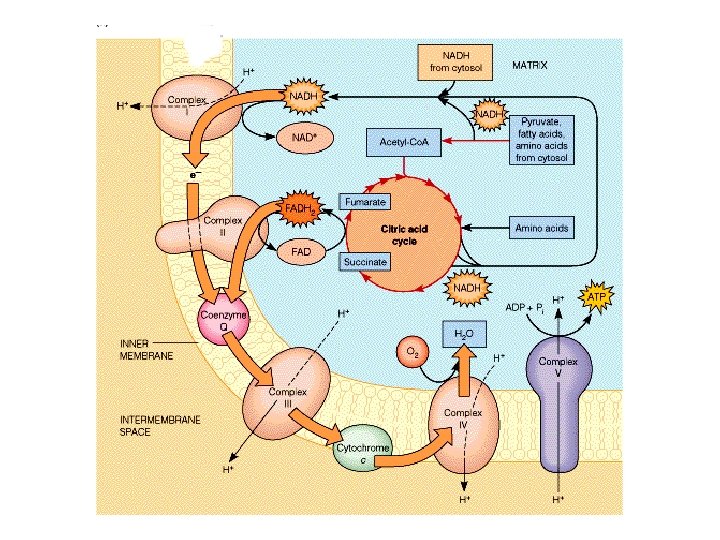
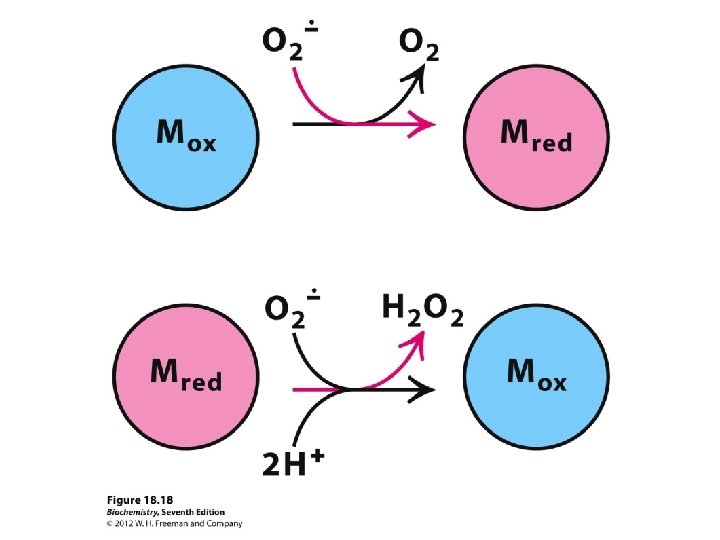
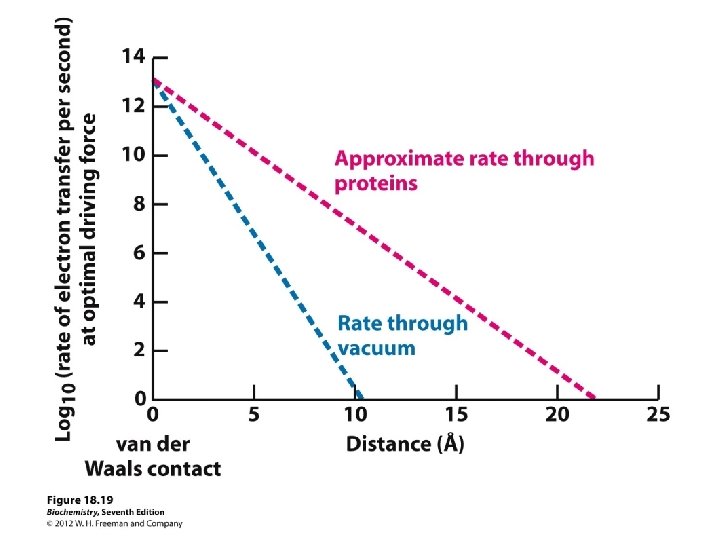
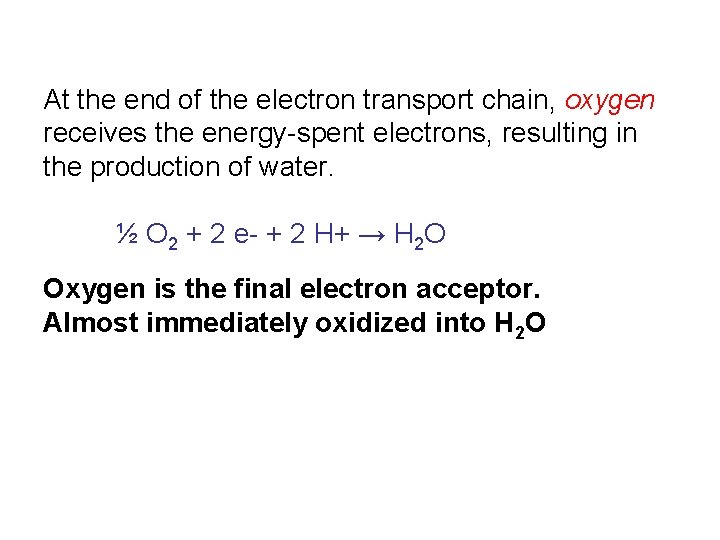
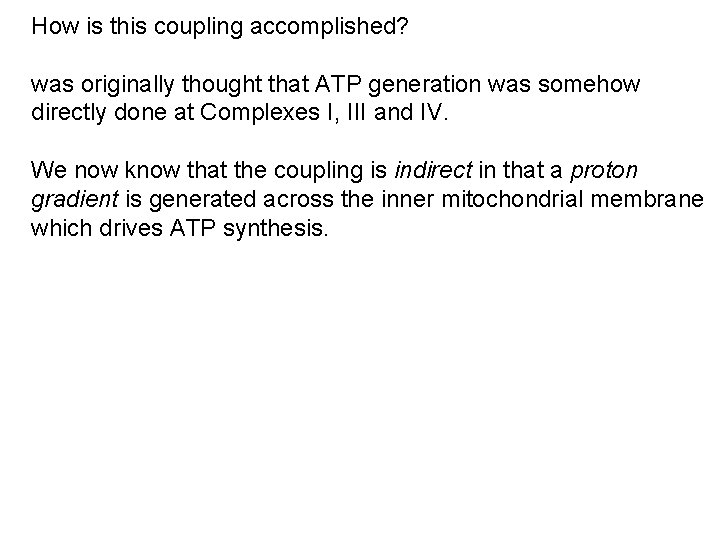
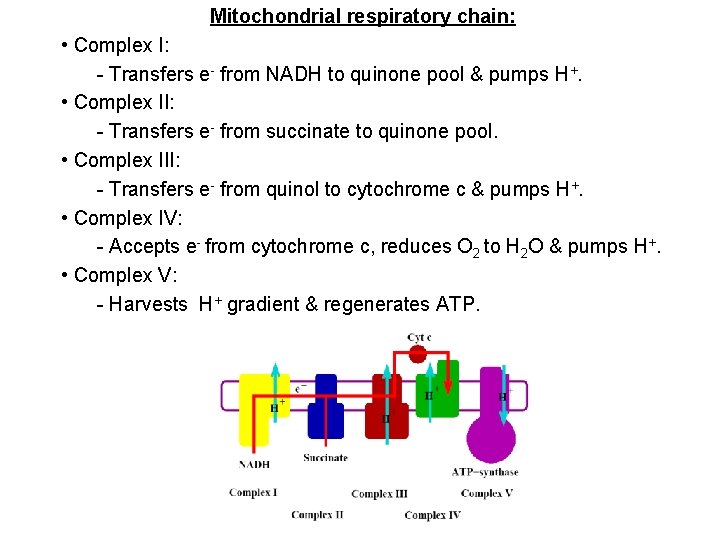
- Slides: 76
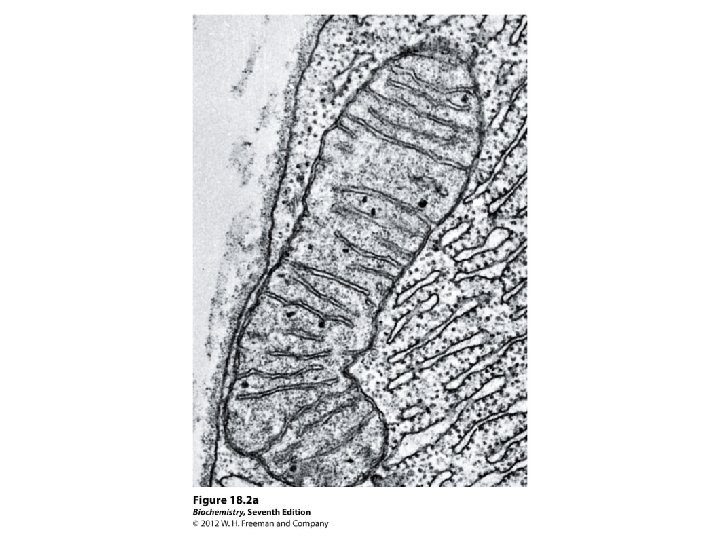
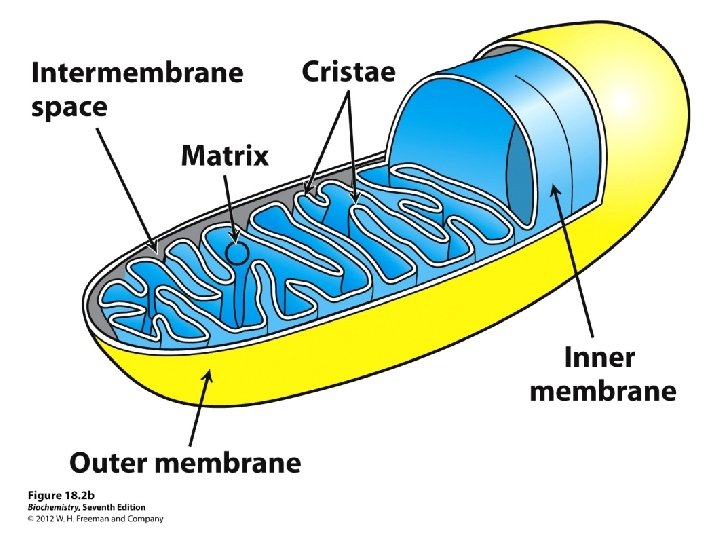
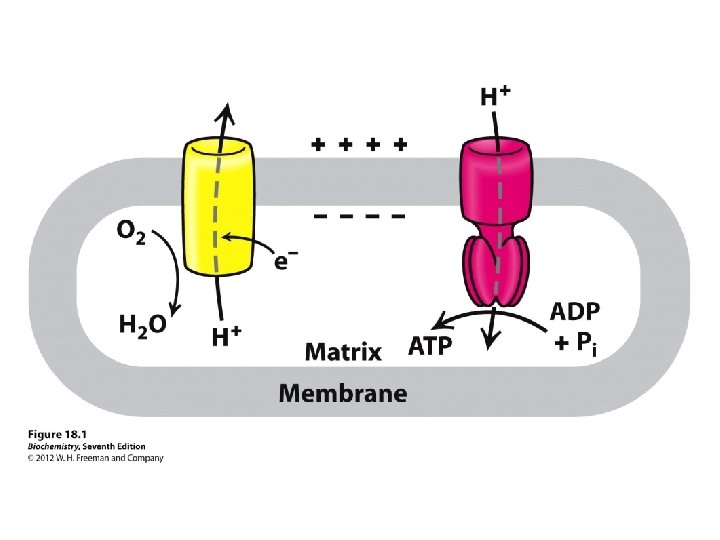
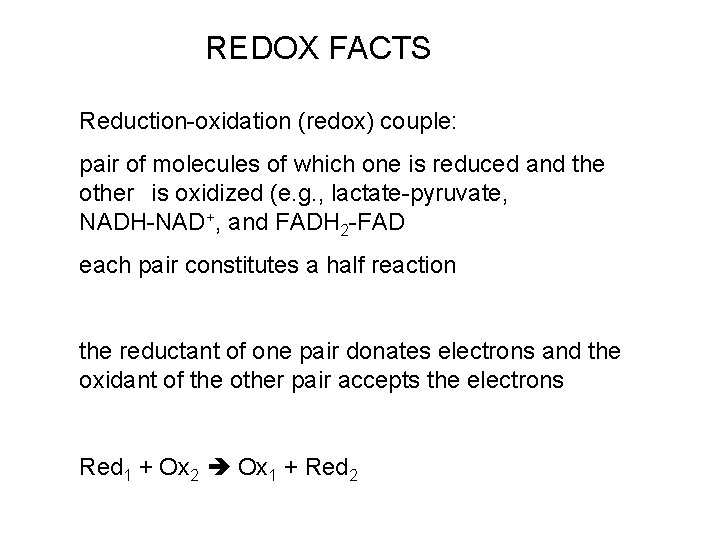
REDOX FACTS Reduction-oxidation (redox) couple: pair of molecules of which one is reduced and the other is oxidized (e. g. , lactate-pyruvate, NADH-NAD+, and FADH 2 -FAD each pair constitutes a half reaction the reductant of one pair donates electrons and the oxidant of the other pair accepts the electrons Red 1 + Ox 2 Ox 1 + Red 2
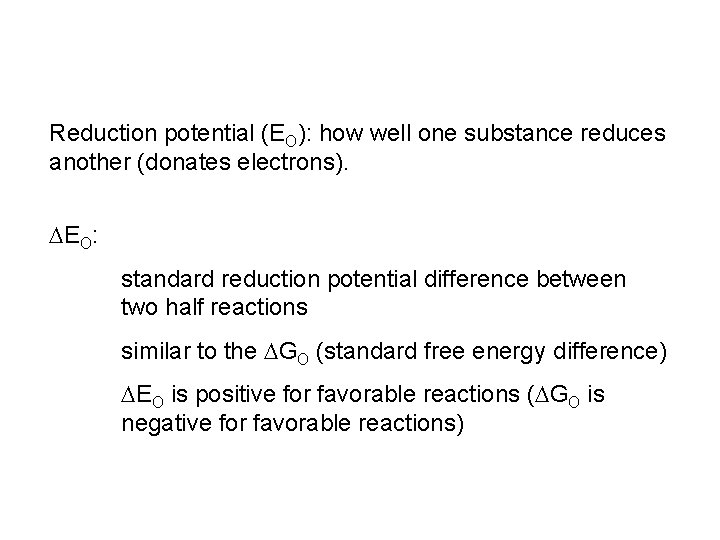
Reduction potential (EO): how well one substance reduces another (donates electrons). EO: standard reduction potential difference between two half reactions similar to the GO (standard free energy difference) EO is positive for favorable reactions ( GO is negative for favorable reactions)
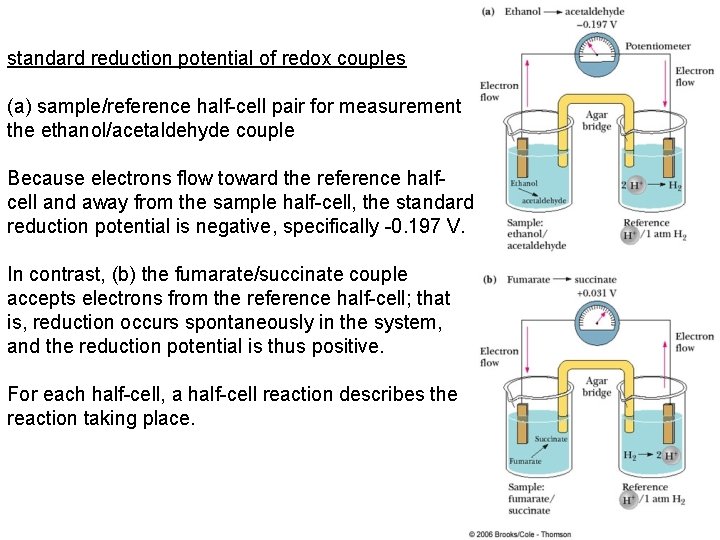
standard reduction potential of redox couples (a) sample/reference half-cell pair for measurement the ethanol/acetaldehyde couple Because electrons flow toward the reference halfcell and away from the sample half-cell, the standard reduction potential is negative, specifically -0. 197 V. In contrast, (b) the fumarate/succinate couple accepts electrons from the reference half-cell; that is, reduction occurs spontaneously in the system, and the reduction potential is thus positive. For each half-cell, a half-cell reaction describes the reaction taking place.
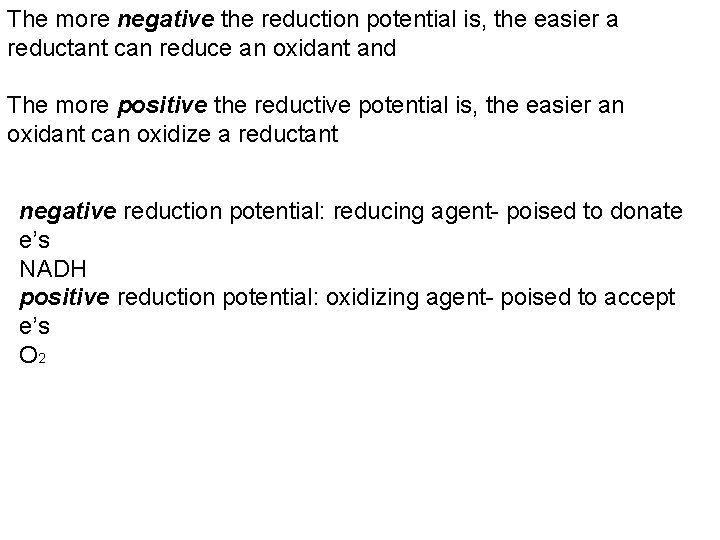
The more negative the reduction potential is, the easier a reductant can reduce an oxidant and The more positive the reductive potential is, the easier an oxidant can oxidize a reductant negative reduction potential: reducing agent- poised to donate e’s NADH positive reduction potential: oxidizing agent- poised to accept e’s O 2
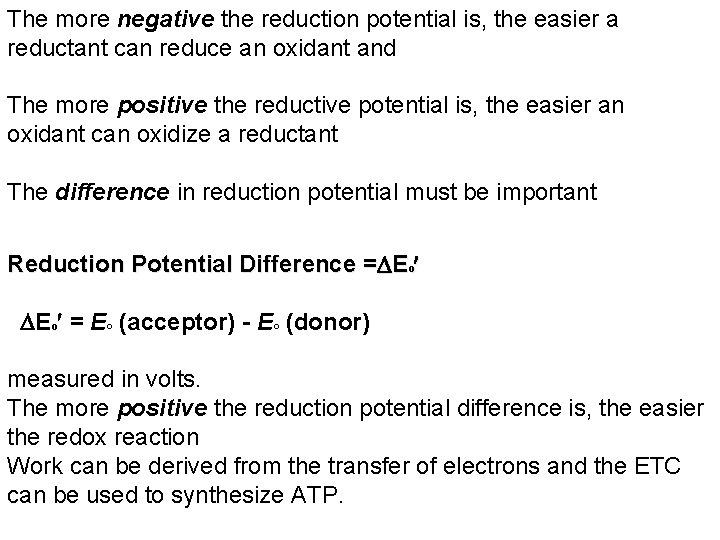
The more negative the reduction potential is, the easier a reductant can reduce an oxidant and The more positive the reductive potential is, the easier an oxidant can oxidize a reductant The difference in reduction potential must be important Reduction Potential Difference = Eº Eº = E° (acceptor) - E° (donor) measured in volts. The more positive the reduction potential difference is, the easier the redox reaction Work can be derived from the transfer of electrons and the ETC can be used to synthesize ATP.
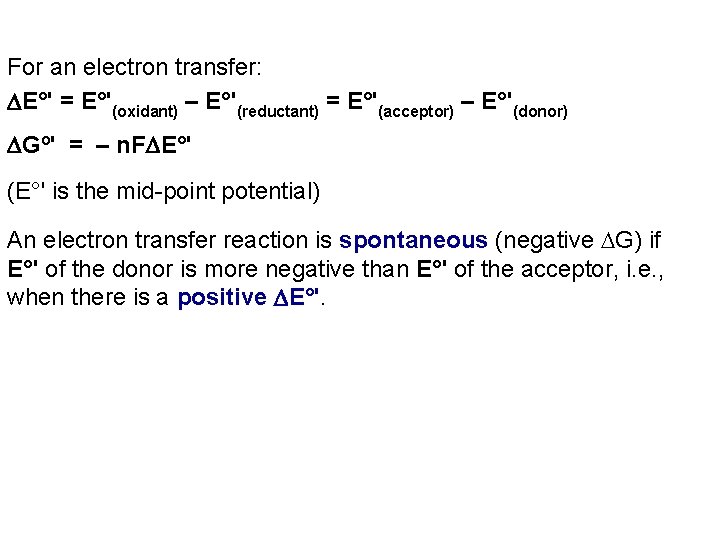
For an electron transfer: E°' = E°'(oxidant) – E°'(reductant) = E°'(acceptor) – E°'(donor) Go' = – n. F E°' (E°' is the mid-point potential) An electron transfer reaction is spontaneous (negative G) if E°' of the donor is more negative than E°' of the acceptor, i. e. , when there is a positive E°'.
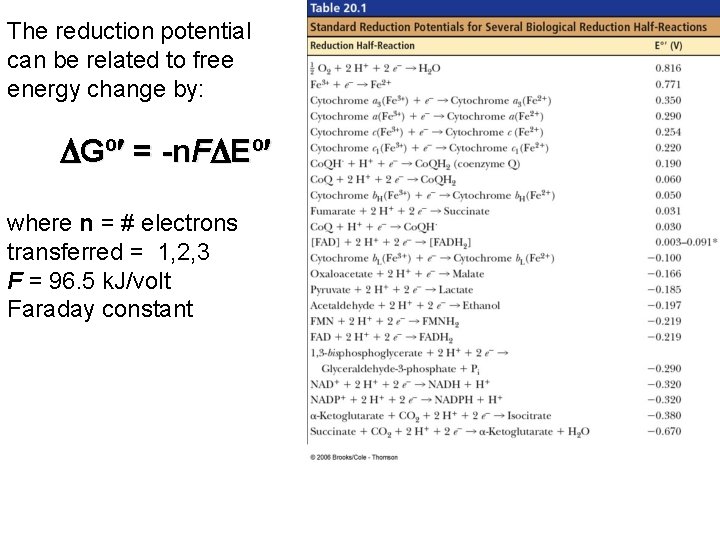
The reduction potential can be related to free energy change by: Gº = -n. F Eº where n = # electrons transferred = 1, 2, 3 F = 96. 5 k. J/volt Faraday constant
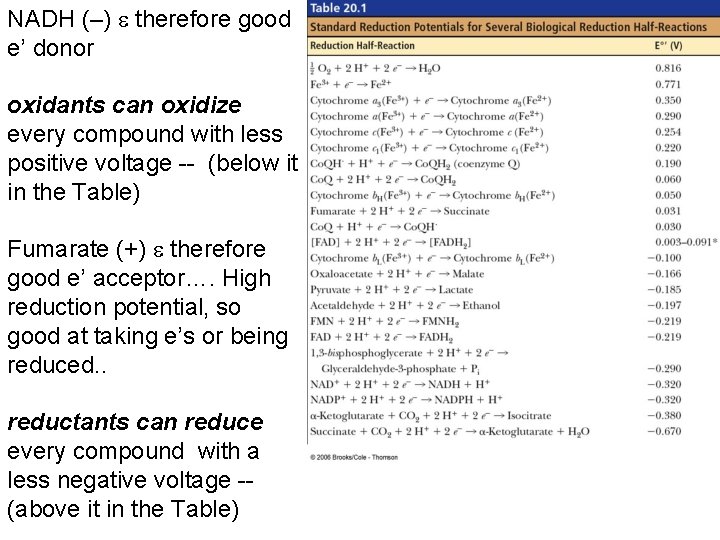
NADH (–) e therefore good e’ donor oxidants can oxidize every compound with less positive voltage -- (below it in the Table) Fumarate (+) e therefore good e’ acceptor…. High reduction potential, so good at taking e’s or being reduced. . reductants can reduce every compound with a less negative voltage -- (above it in the Table)
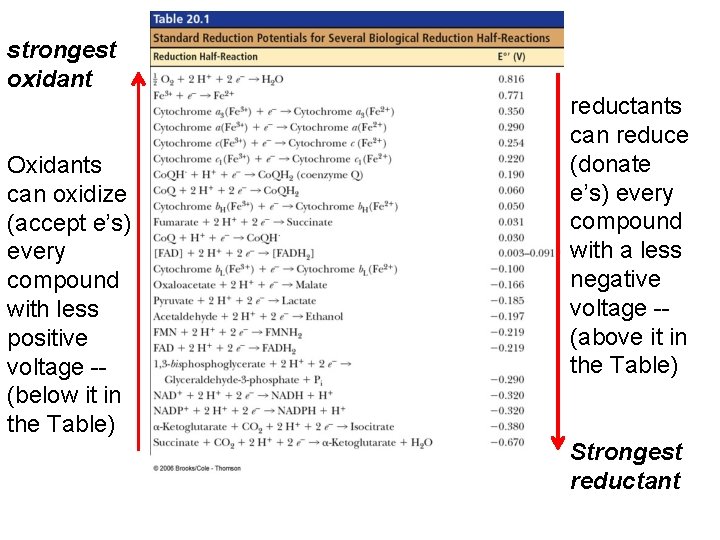
strongest oxidant Oxidants can oxidize (accept e’s) every compound with less positive voltage -- (below it in the Table) reductants can reduce (donate e’s) every compound with a less negative voltage -- (above it in the Table) Strongest reductant
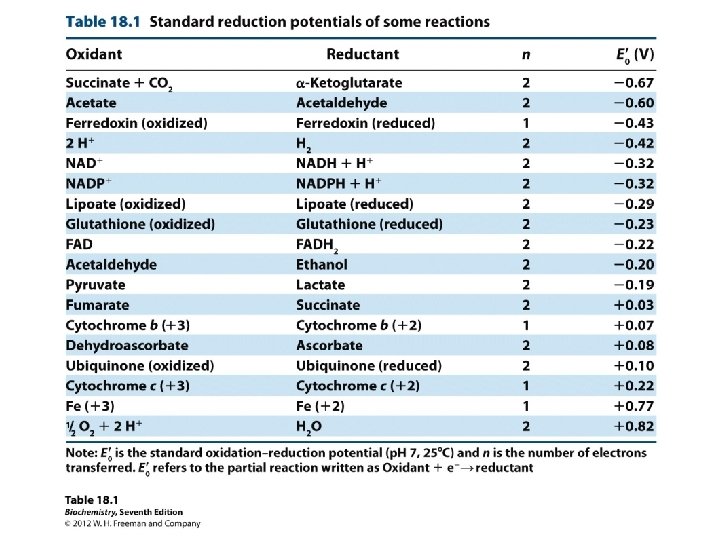
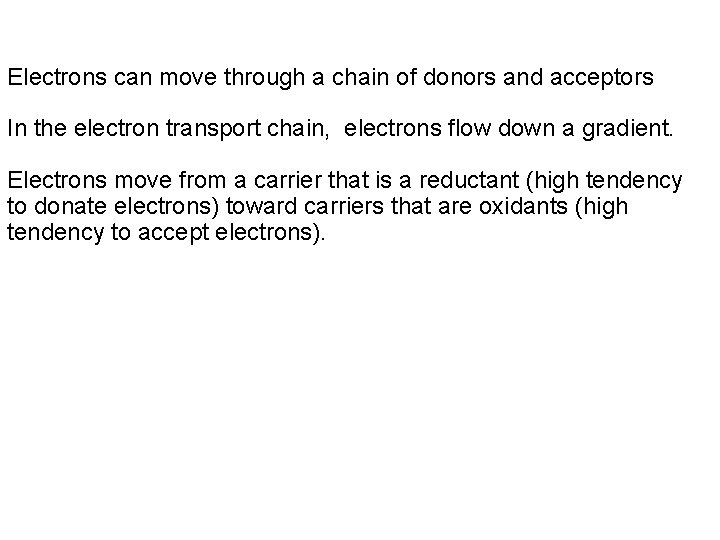
Electrons can move through a chain of donors and acceptors In the electron transport chain, electrons flow down a gradient. Electrons move from a carrier that is a reductant (high tendency to donate electrons) toward carriers that are oxidants (high tendency to accept electrons).
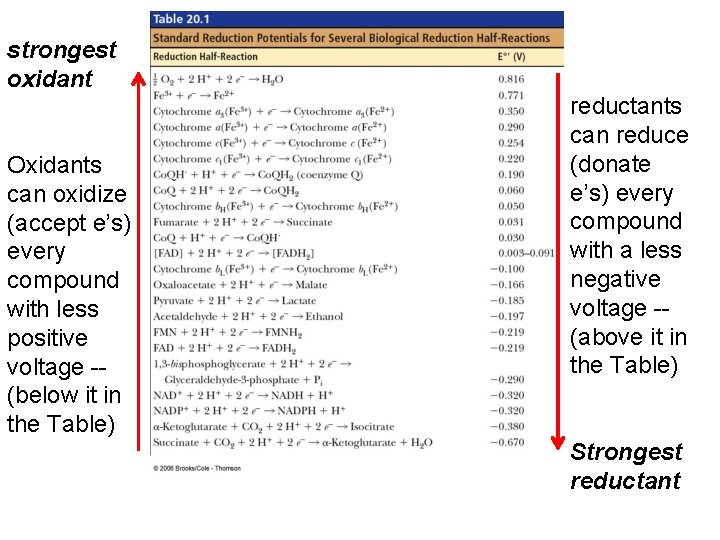
strongest oxidant Oxidants can oxidize (accept e’s) every compound with less positive voltage -- (below it in the Table) reductants can reduce (donate e’s) every compound with a less negative voltage -- (above it in the Table) Strongest reductant
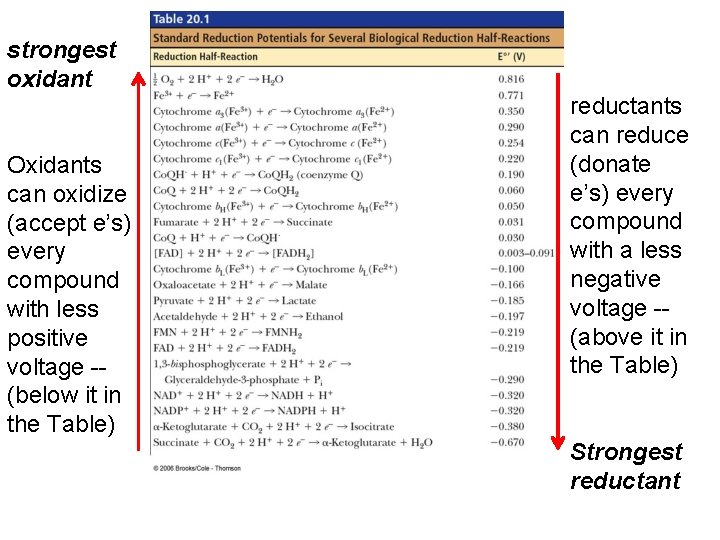
strongest oxidant Oxidants can oxidize (accept e’s) every compound with less positive voltage -- (below it in the Table) reductants can reduce (donate e’s) every compound with a less negative voltage -- (above it in the Table) Strongest reductant
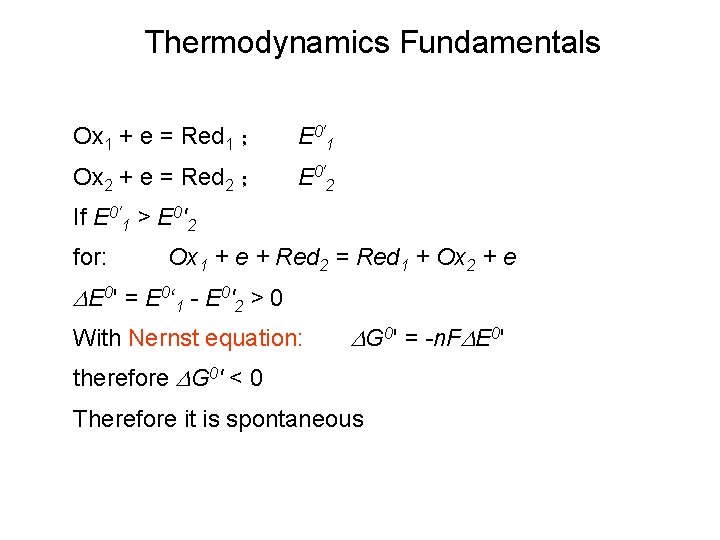
Thermodynamics Fundamentals Ox 1 + e = Red 1 ; E 0'1 Ox 2 + e = Red 2 ; E 0'2 If E 0'1 > E 0'2 for: Ox 1 + e + Red 2 = Red 1 + Ox 2 + e DE 0' = E 0‘ 1 - E 0'2 > 0 With Nernst equation: DG 0' = -n. FDE 0' therefore DG 0' < 0 Therefore it is spontaneous
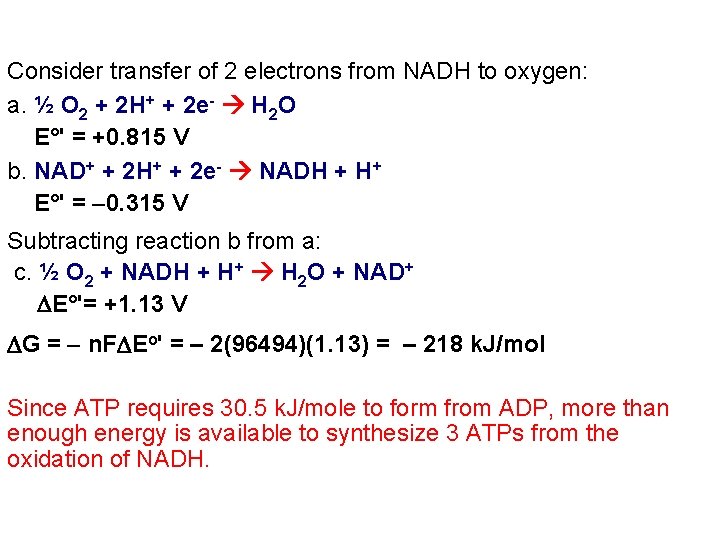
Consider transfer of 2 electrons from NADH to oxygen: a. ½ O 2 + 2 H+ + 2 e- H 2 O E°' = +0. 815 V b. NAD+ + 2 H+ + 2 e- NADH + H+ E°' = -0. 315 V Subtracting reaction b from a: c. ½ O 2 + NADH + H+ H 2 O + NAD+ E°'= +1. 13 V G = - n. F Eo' = – 2(96494)(1. 13) = – 218 k. J/mol Since ATP requires 30. 5 k. J/mole to form from ADP, more than enough energy is available to synthesize 3 ATPs from the oxidation of NADH.
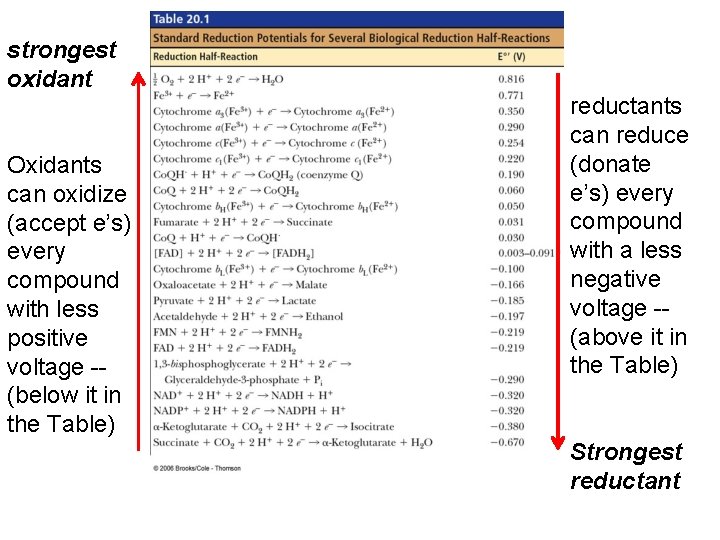
strongest oxidant Oxidants can oxidize (accept e’s) every compound with less positive voltage -- (below it in the Table) reductants can reduce (donate e’s) every compound with a less negative voltage -- (above it in the Table) Strongest reductant
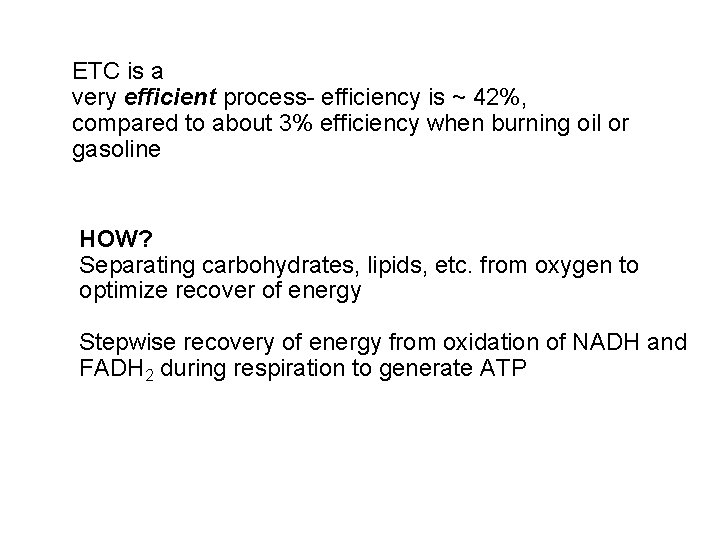
ETC is a very efficient process- efficiency is ~ 42%, compared to about 3% efficiency when burning oil or gasoline HOW? Separating carbohydrates, lipids, etc. from oxygen to optimize recover of energy Stepwise recovery of energy from oxidation of NADH and FADH 2 during respiration to generate ATP
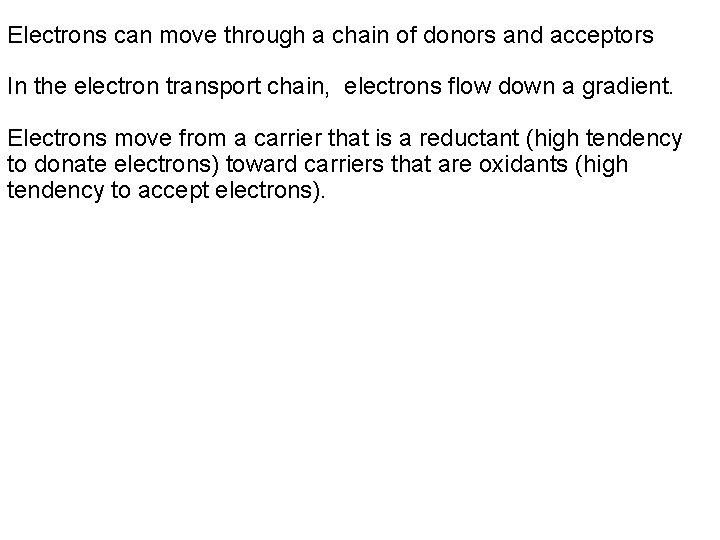
Electrons can move through a chain of donors and acceptors In the electron transport chain, electrons flow down a gradient. Electrons move from a carrier that is a reductant (high tendency to donate electrons) toward carriers that are oxidants (high tendency to accept electrons).
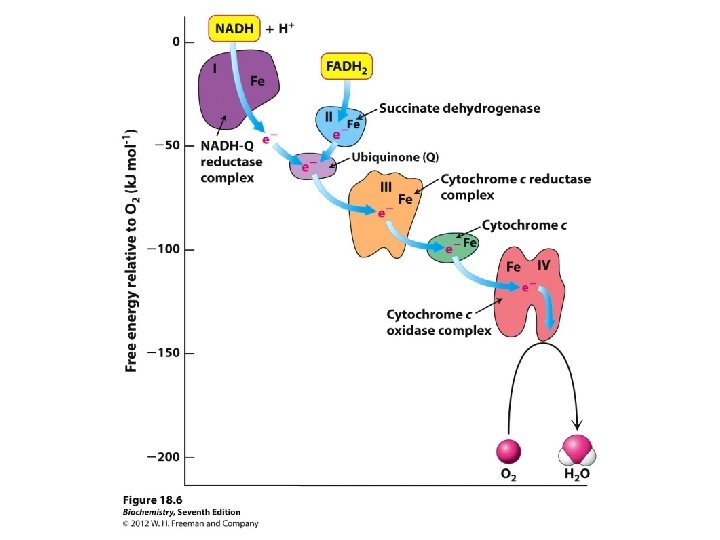
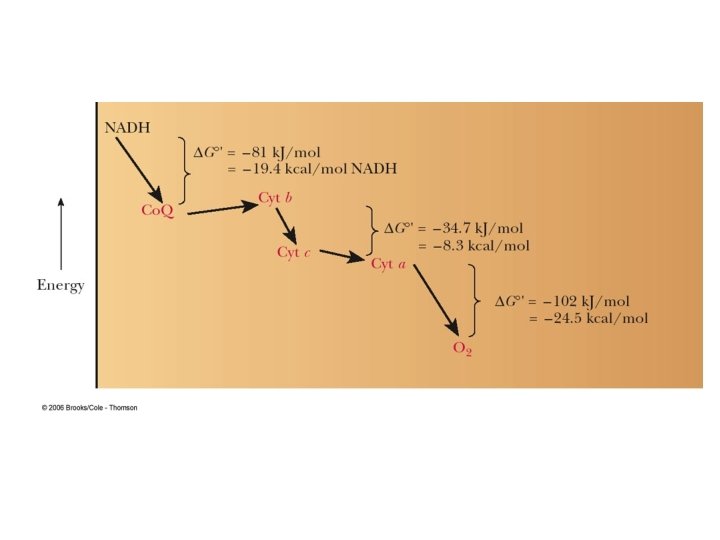
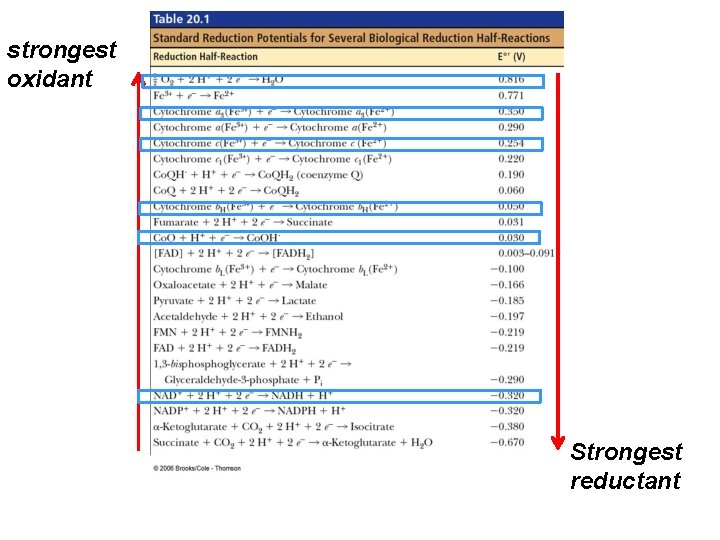
strongest oxidant Strongest reductant
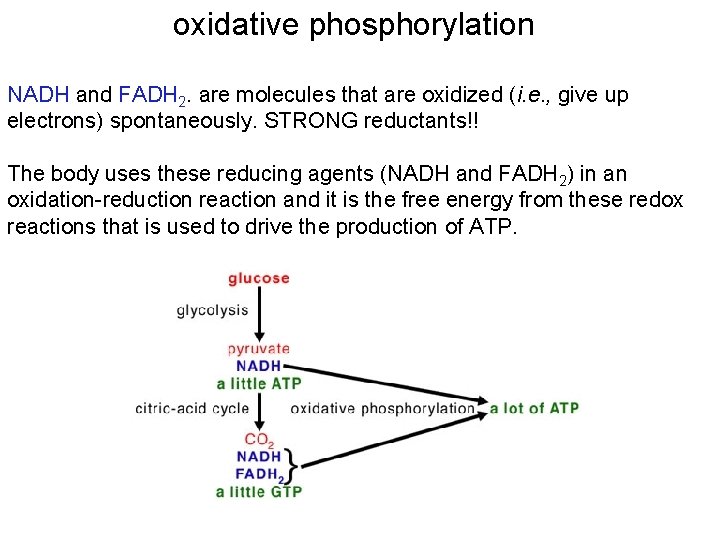
oxidative phosphorylation NADH and FADH 2. are molecules that are oxidized (i. e. , give up electrons) spontaneously. STRONG reductants!! The body uses these reducing agents (NADH and FADH 2) in an oxidation-reduction reaction and it is the free energy from these redox reactions that is used to drive the production of ATP.
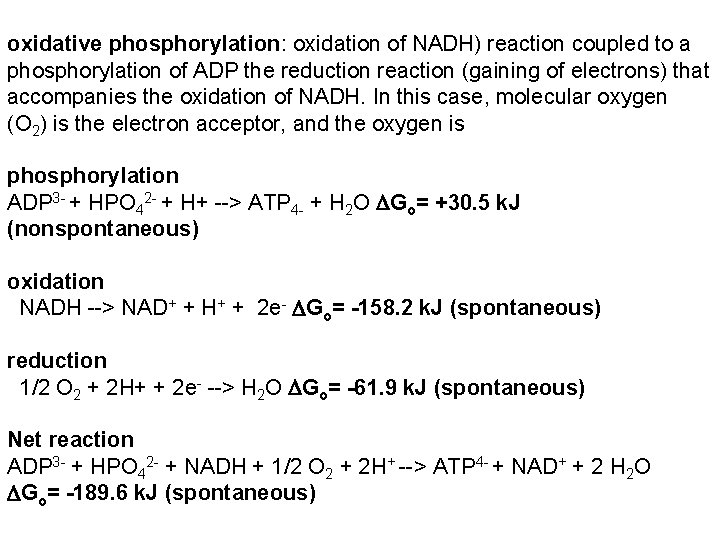
oxidative phosphorylation: oxidation of NADH) reaction coupled to a phosphorylation of ADP the reduction reaction (gaining of electrons) that accompanies the oxidation of NADH. In this case, molecular oxygen (O 2) is the electron acceptor, and the oxygen is phosphorylation ADP 3 - + HPO 42 - + H+ --> ATP 4 - + H 2 O Go= +30. 5 k. J (nonspontaneous) oxidation NADH --> NAD+ + H+ + 2 e- Go= -158. 2 k. J (spontaneous) reduction 1/2 O 2 + 2 H+ + 2 e- --> H 2 O Go= -61. 9 k. J (spontaneous) Net reaction ADP 3 - + HPO 42 - + NADH + 1/2 O 2 + 2 H+ --> ATP 4 - + NAD+ + 2 H 2 O Go= -189. 6 k. J (spontaneous)
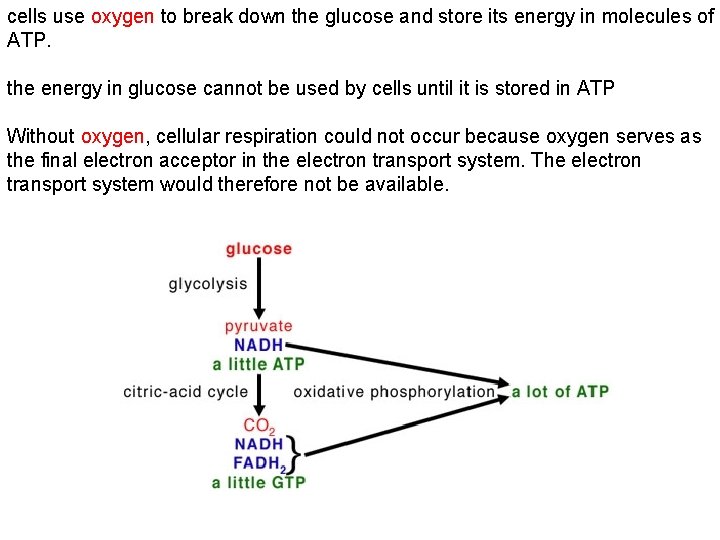
cells use oxygen to break down the glucose and store its energy in molecules of ATP. the energy in glucose cannot be used by cells until it is stored in ATP Without oxygen, cellular respiration could not occur because oxygen serves as the final electron acceptor in the electron transport system. The electron transport system would therefore not be available.
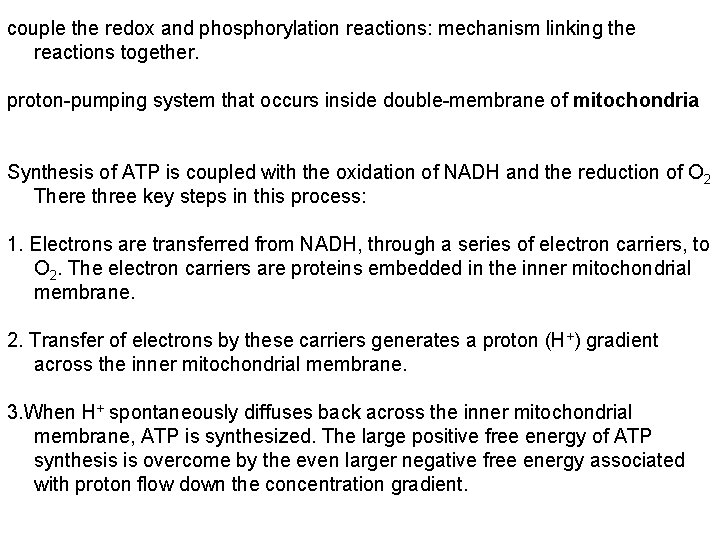
couple the redox and phosphorylation reactions: mechanism linking the reactions together. proton-pumping system that occurs inside double-membrane of mitochondria Synthesis of ATP is coupled with the oxidation of NADH and the reduction of O 2 There three key steps in this process: 1. Electrons are transferred from NADH, through a series of electron carriers, to O 2. The electron carriers are proteins embedded in the inner mitochondrial membrane. 2. Transfer of electrons by these carriers generates a proton (H+) gradient across the inner mitochondrial membrane. 3. When H+ spontaneously diffuses back across the inner mitochondrial membrane, ATP is synthesized. The large positive free energy of ATP synthesis is overcome by the even larger negative free energy associated with proton flow down the concentration gradient.
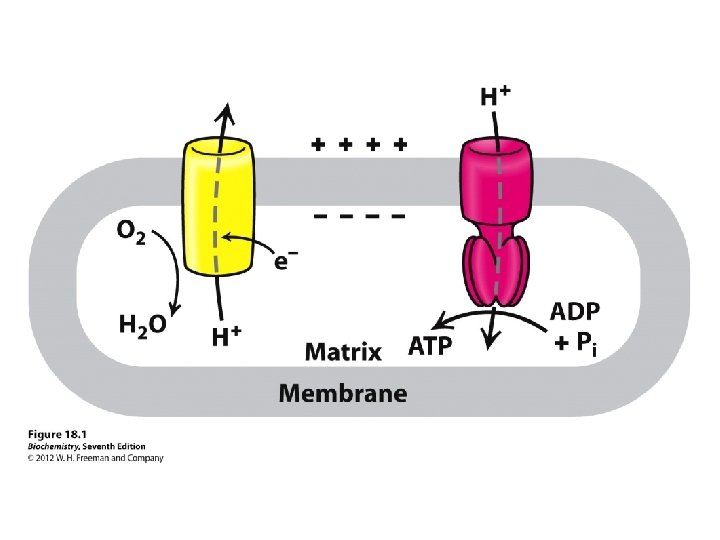
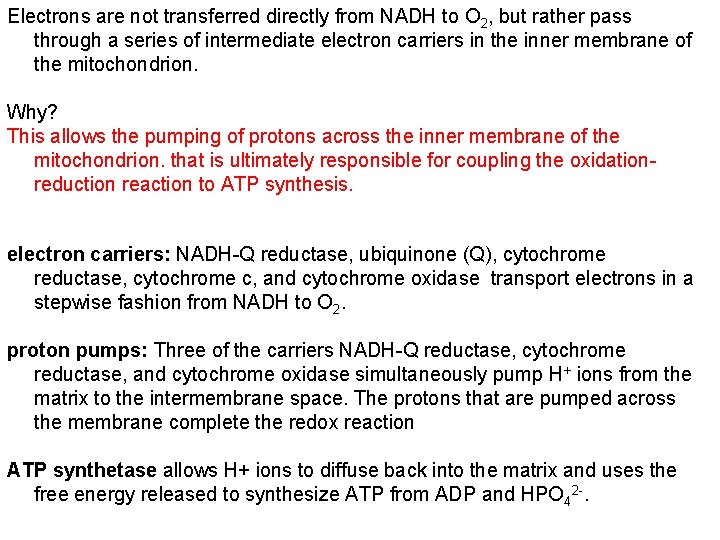
Electrons are not transferred directly from NADH to O 2, but rather pass through a series of intermediate electron carriers in the inner membrane of the mitochondrion. Why? This allows the pumping of protons across the inner membrane of the mitochondrion. that is ultimately responsible for coupling the oxidationreduction reaction to ATP synthesis. electron carriers: NADH-Q reductase, ubiquinone (Q), cytochrome reductase, cytochrome c, and cytochrome oxidase transport electrons in a stepwise fashion from NADH to O 2. proton pumps: Three of the carriers NADH-Q reductase, cytochrome reductase, and cytochrome oxidase simultaneously pump H+ ions from the matrix to the intermembrane space. The protons that are pumped across the membrane complete the redox reaction ATP synthetase allows H+ ions to diffuse back into the matrix and uses the free energy released to synthesize ATP from ADP and HPO 42 -.
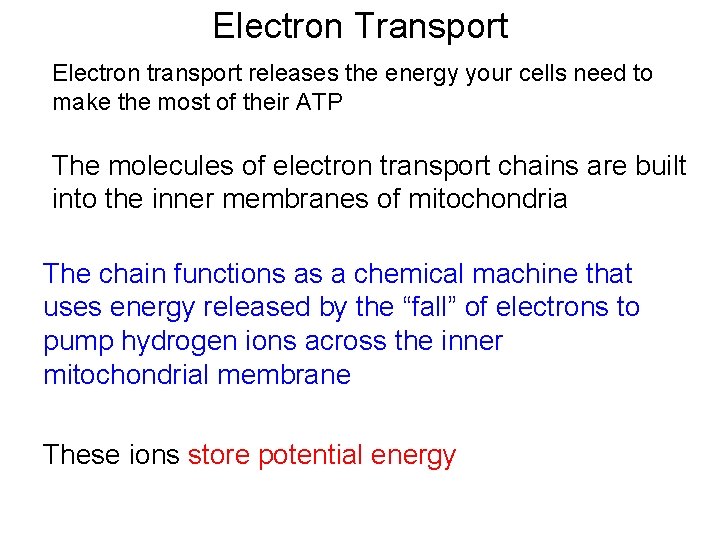
Electron Transport Electron transport releases the energy your cells need to make the most of their ATP The molecules of electron transport chains are built into the inner membranes of mitochondria The chain functions as a chemical machine that uses energy released by the “fall” of electrons to pump hydrogen ions across the inner mitochondrial membrane These ions store potential energy
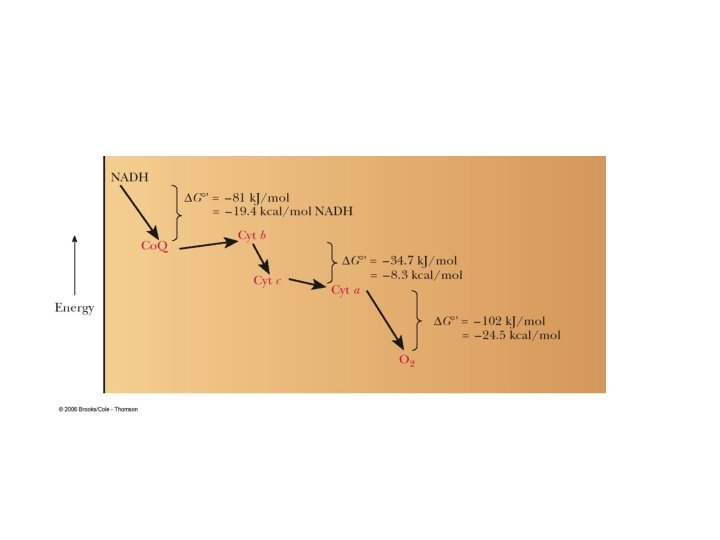
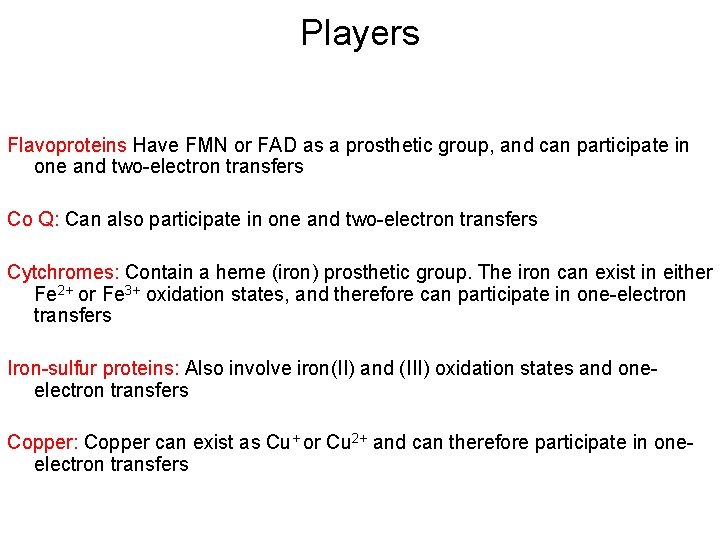
Players Flavoproteins Have FMN or FAD as a prosthetic group, and can participate in one and two-electron transfers Co Q: Can also participate in one and two-electron transfers Cytchromes: Contain a heme (iron) prosthetic group. The iron can exist in either Fe 2+ or Fe 3+ oxidation states, and therefore can participate in one-electron transfers Iron-sulfur proteins: Also involve iron(II) and (III) oxidation states and oneelectron transfers Copper: Copper can exist as Cu+ or Cu 2+ and can therefore participate in oneelectron transfers
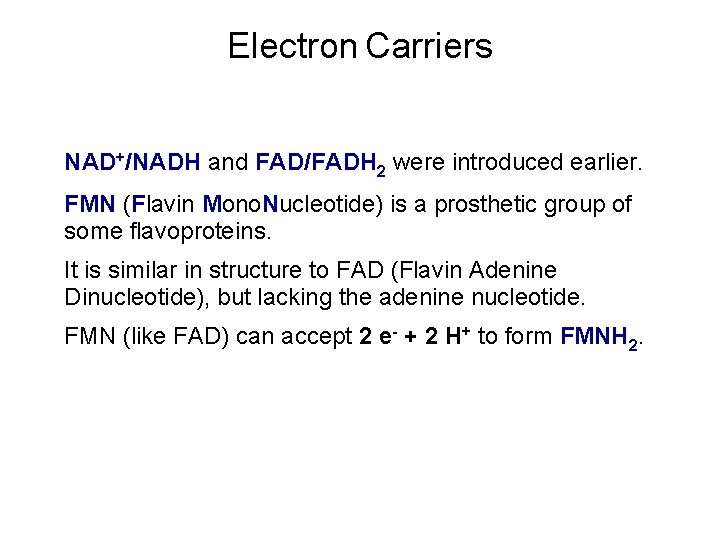
Electron Carriers NAD+/NADH and FAD/FADH 2 were introduced earlier. FMN (Flavin Mono. Nucleotide) is a prosthetic group of some flavoproteins. It is similar in structure to FAD (Flavin Adenine Dinucleotide), but lacking the adenine nucleotide. FMN (like FAD) can accept 2 e- + 2 H+ to form FMNH 2.
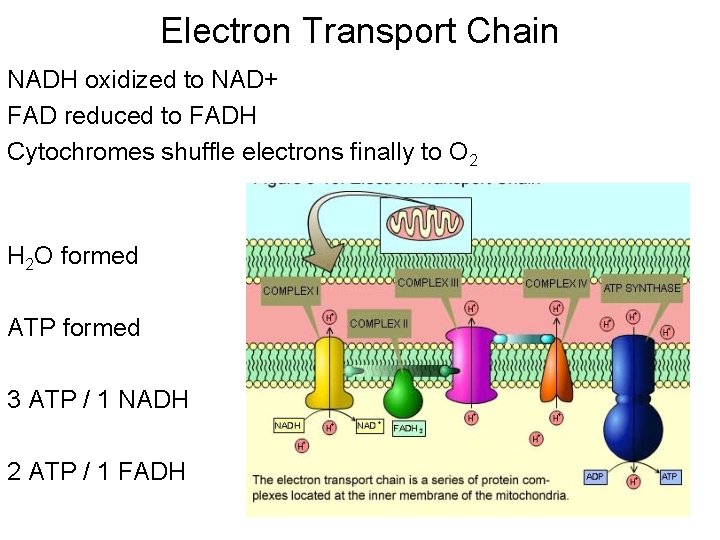
Electron Transport Chain NADH oxidized to NAD+ FAD reduced to FADH Cytochromes shuffle electrons finally to O 2 H 2 O formed ATP formed 3 ATP / 1 NADH 2 ATP / 1 FADH
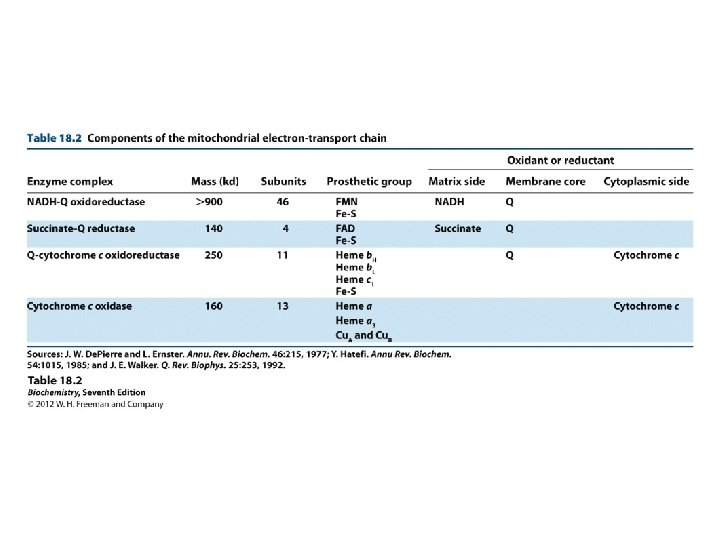
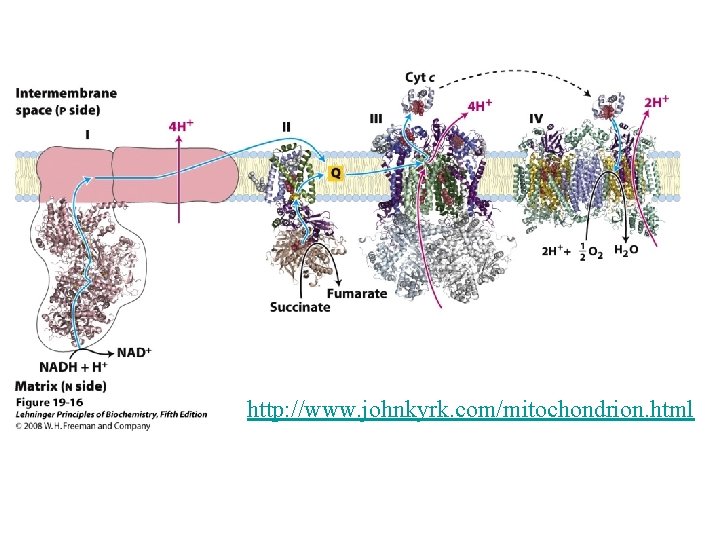
http: //www. johnkyrk. com/mitochondrion. html
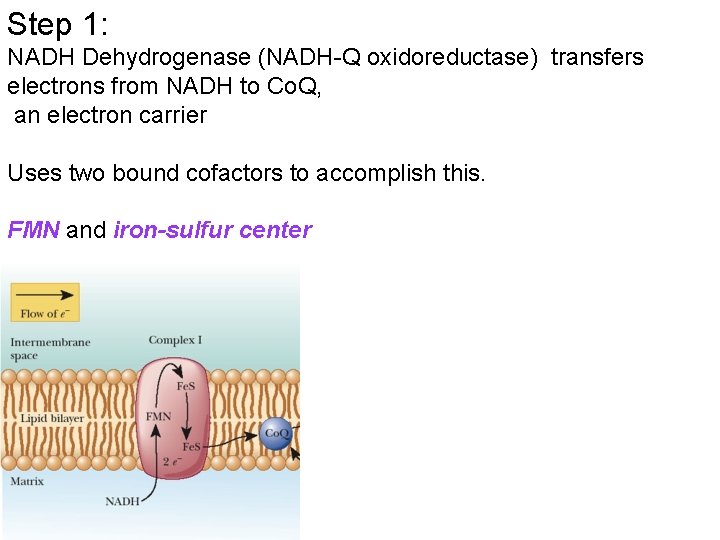
Step 1: NADH Dehydrogenase (NADH-Q oxidoreductase) transfers electrons from NADH to Co. Q, an electron carrier Uses two bound cofactors to accomplish this. FMN and iron-sulfur center
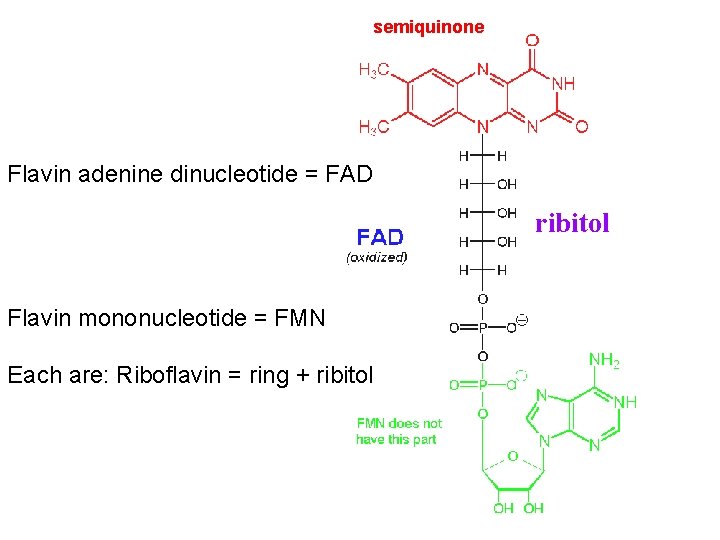
semiquinone Flavin adenine dinucleotide = FAD ribitol Flavin mononucleotide = FMN Each are: Riboflavin = ring + ribitol
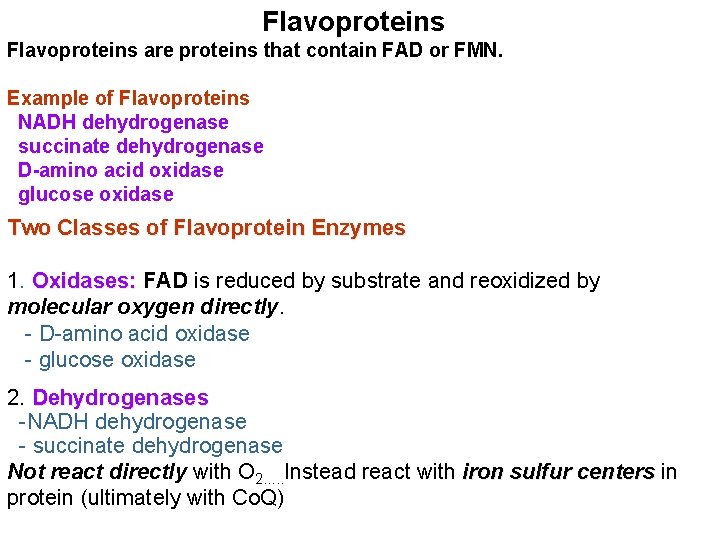
Flavoproteins are proteins that contain FAD or FMN. Example of Flavoproteins NADH dehydrogenase succinate dehydrogenase D-amino acid oxidase glucose oxidase Two Classes of Flavoprotein Enzymes 1. Oxidases: FAD is reduced by substrate and reoxidized by Oxidases: molecular oxygen directly. - D-amino acid oxidase - glucose oxidase 2. Dehydrogenases -NADH dehydrogenase - succinate dehydrogenase Not react directly with O 2. . . Instead react with iron sulfur centers in protein (ultimately with Co. Q)
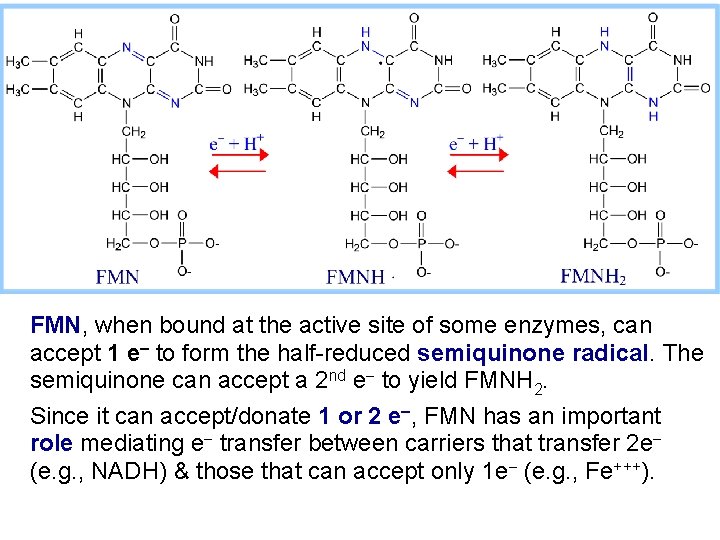
FMN, when bound at the active site of some enzymes, can accept 1 e- to form the half-reduced semiquinone radical. The semiquinone can accept a 2 nd e- to yield FMNH 2. Since it can accept/donate 1 or 2 e-, FMN has an important role mediating e- transfer between carriers that transfer 2 e- (e. g. , NADH) & those that can accept only 1 e- (e. g. , Fe+++).
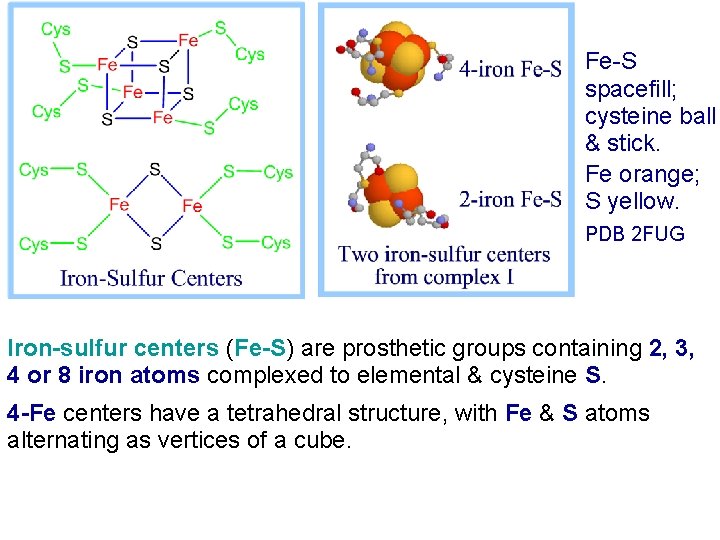
Fe-S spacefill; cysteine ball & stick. Fe orange; S yellow. PDB 2 FUG Iron-sulfur centers (Fe-S) are prosthetic groups containing 2, 3, 4 or 8 iron atoms complexed to elemental & cysteine S. 4 -Fe centers have a tetrahedral structure, with Fe & S atoms alternating as vertices of a cube.
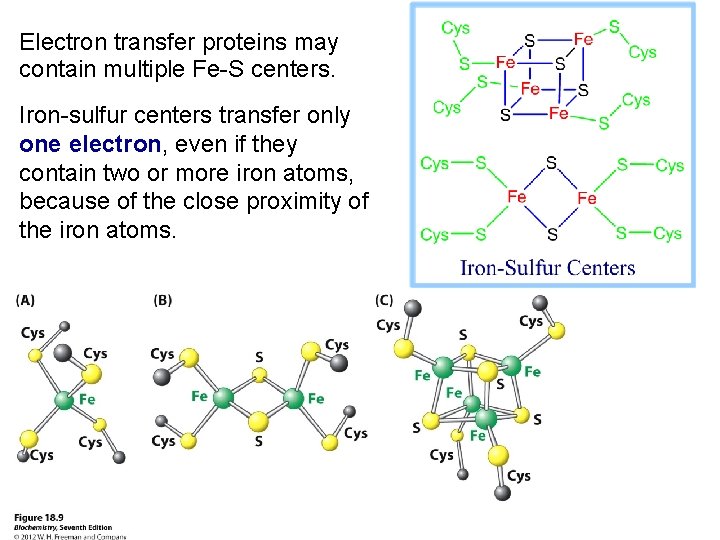
Electron transfer proteins may contain multiple Fe-S centers. Iron-sulfur centers transfer only one electron, even if they contain two or more iron atoms, because of the close proximity of the iron atoms.
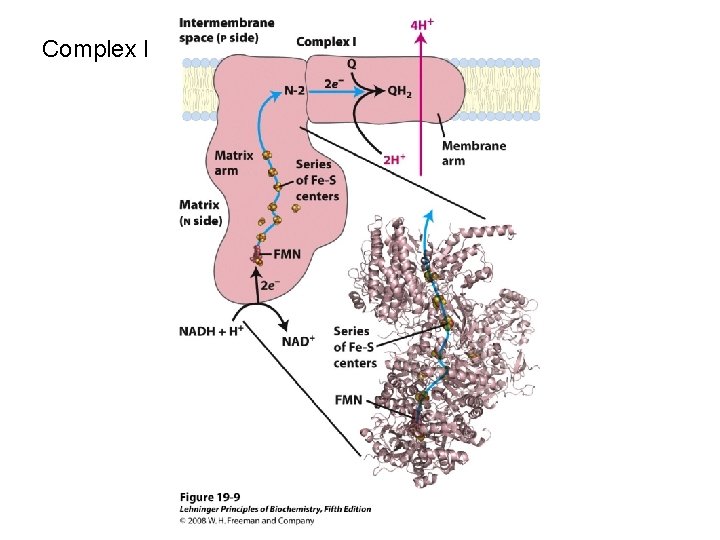
Complex I
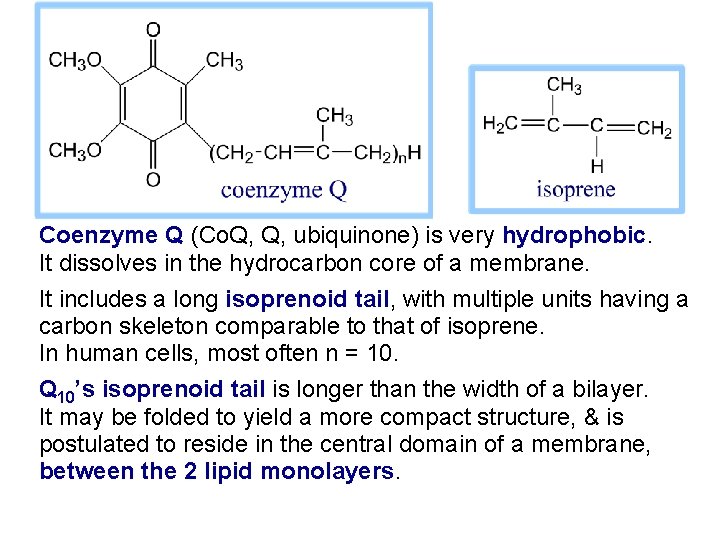
Coenzyme Q (Co. Q, Q, ubiquinone) is very hydrophobic. It dissolves in the hydrocarbon core of a membrane. It includes a long isoprenoid tail, with multiple units having a carbon skeleton comparable to that of isoprene. In human cells, most often n = 10. Q 10’s isoprenoid tail is longer than the width of a bilayer. It may be folded to yield a more compact structure, & is postulated to reside in the central domain of a membrane, between the 2 lipid monolayers.
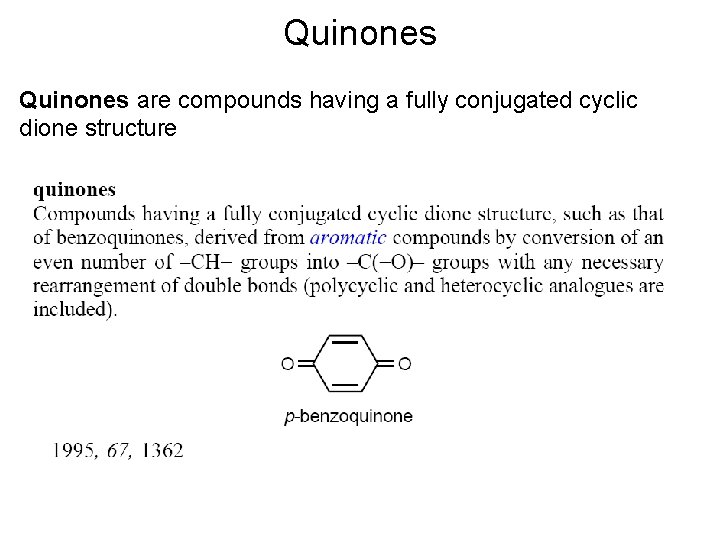
Quinones are compounds having a fully conjugated cyclic dione structure
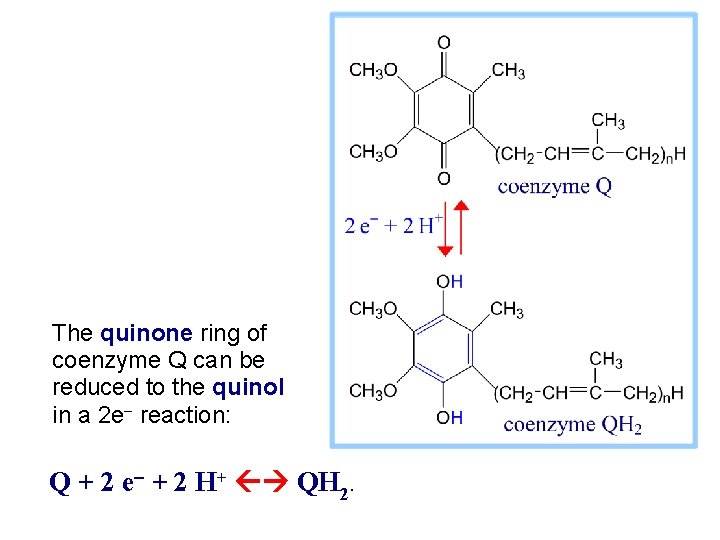
The quinone ring of coenzyme Q can be reduced to the quinol in a 2 e- reaction: Q + 2 e- + 2 H+ QH 2.
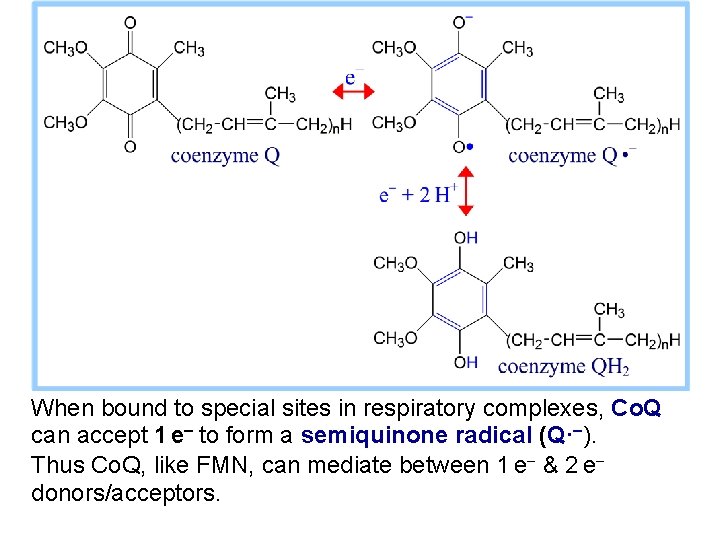
When bound to special sites in respiratory complexes, Co. Q can accept 1 e- to form a semiquinone radical (Q·-). Thus Co. Q, like FMN, can mediate between 1 e- & 2 e- donors/acceptors.
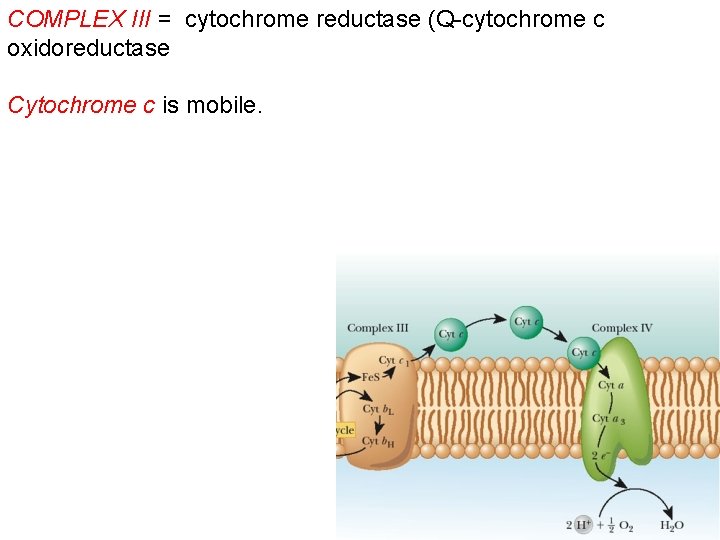
COMPLEX III = cytochrome reductase (Q-cytochrome c oxidoreductase Cytochrome c is mobile.
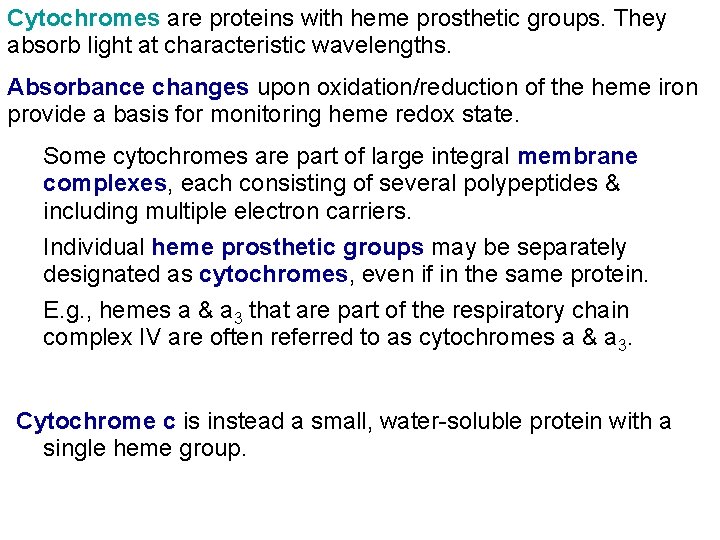
Cytochromes are proteins with heme prosthetic groups. They absorb light at characteristic wavelengths. Absorbance changes upon oxidation/reduction of the heme iron provide a basis for monitoring heme redox state. Some cytochromes are part of large integral membrane complexes, each consisting of several polypeptides & including multiple electron carriers. Individual heme prosthetic groups may be separately designated as cytochromes, even if in the same protein. E. g. , hemes a & a 3 that are part of the respiratory chain complex IV are often referred to as cytochromes a & a 3. Cytochrome c is instead a small, water-soluble protein with a single heme group.
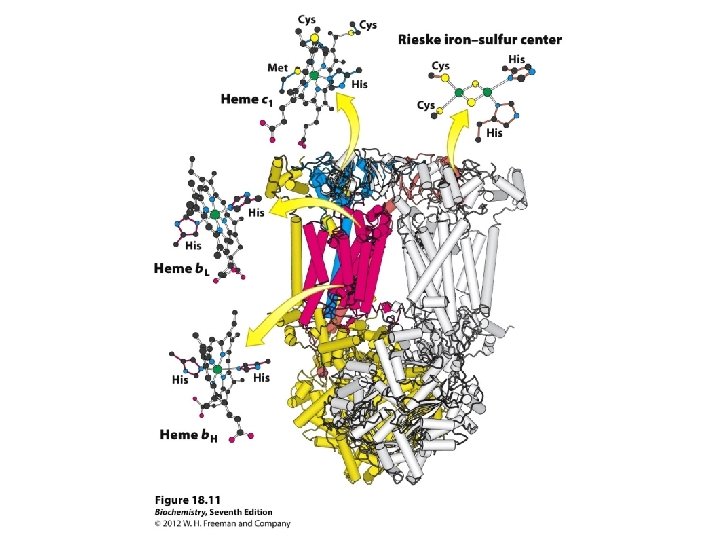
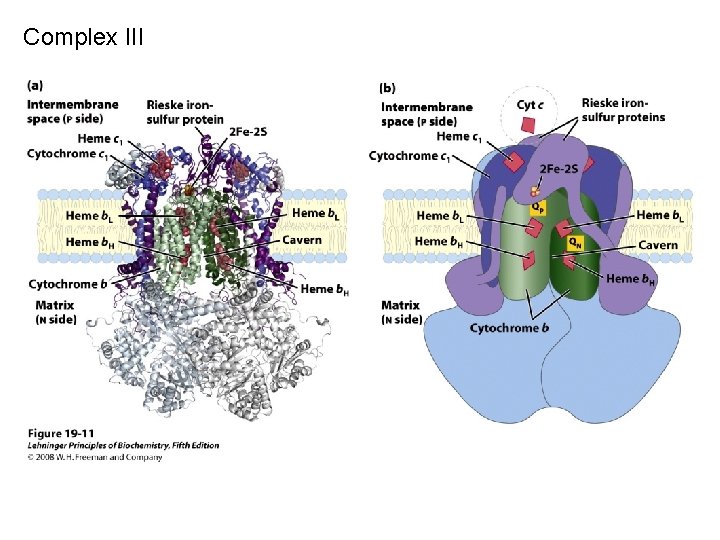
Complex III
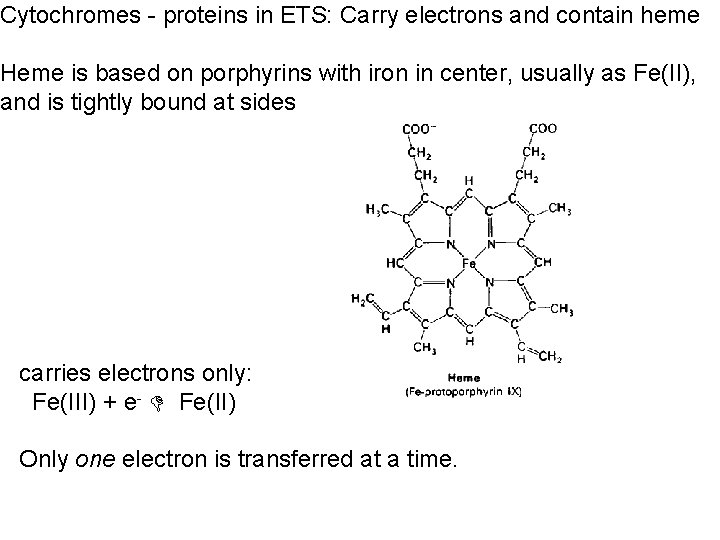
Cytochromes - proteins in ETS: Carry electrons and contain heme Heme is based on porphyrins with iron in center, usually as Fe(II), and is tightly bound at sides carries electrons only: Fe(III) + e- Fe(II) Only one electron is transferred at a time.
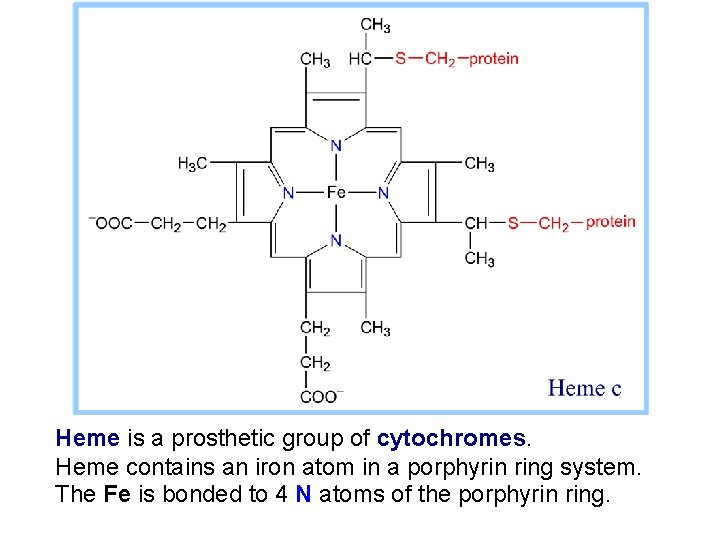
Heme is a prosthetic group of cytochromes. Heme contains an iron atom in a porphyrin ring system. The Fe is bonded to 4 N atoms of the porphyrin ring.
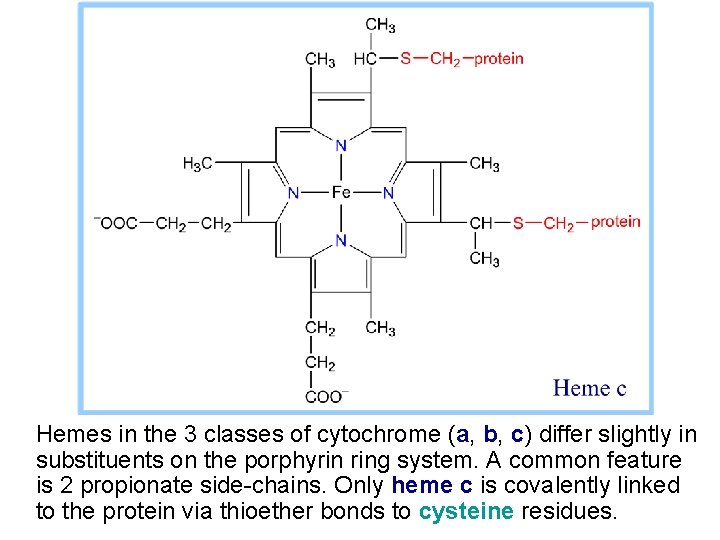
Hemes in the 3 classes of cytochrome (a, b, c) differ slightly in substituents on the porphyrin ring system. A common feature is 2 propionate side-chains. Only heme c is covalently linked to the protein via thioether bonds to cysteine residues.
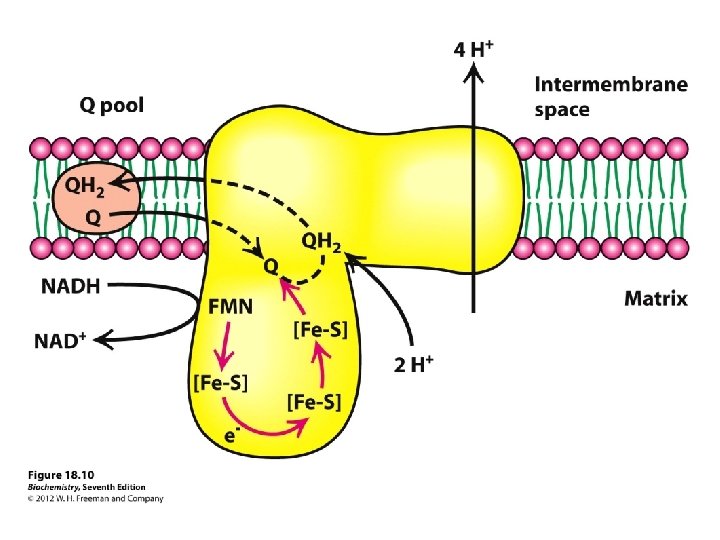
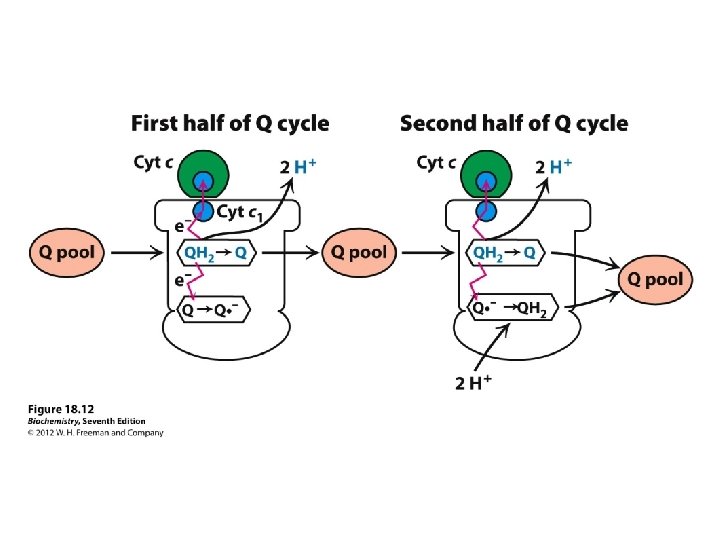
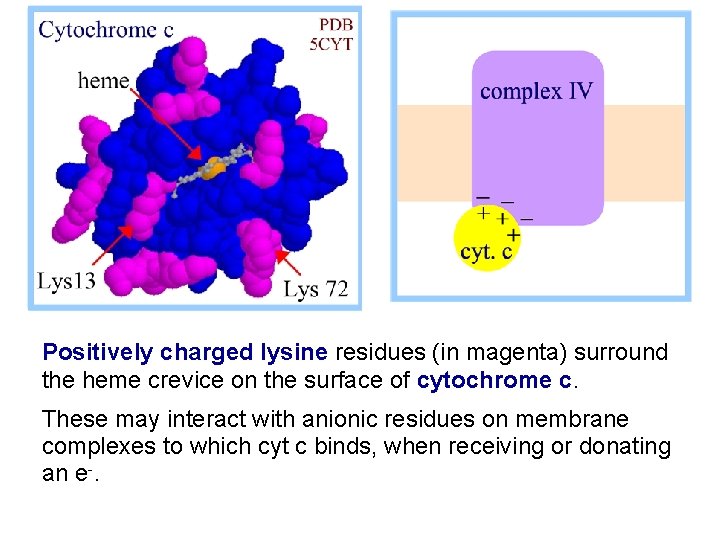
Positively charged lysine residues (in magenta) surround the heme crevice on the surface of cytochrome c. These may interact with anionic residues on membrane complexes to which cyt c binds, when receiving or donating an e-.
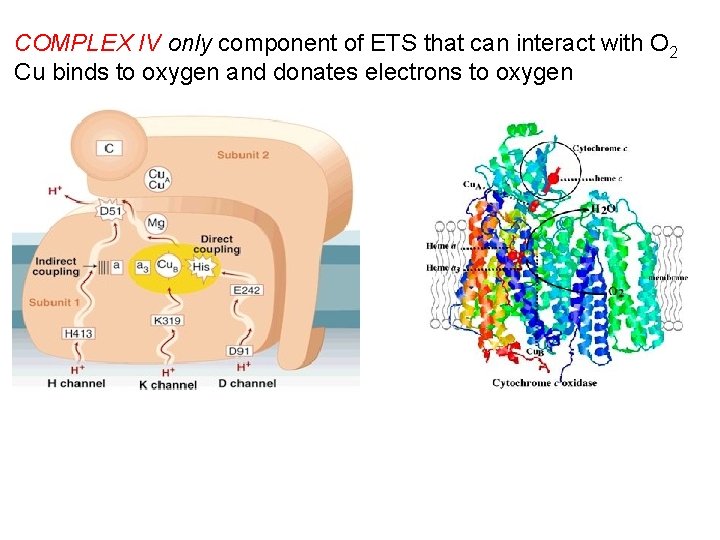
COMPLEX IV only component of ETS that can interact with O 2 Cu binds to oxygen and donates electrons to oxygen
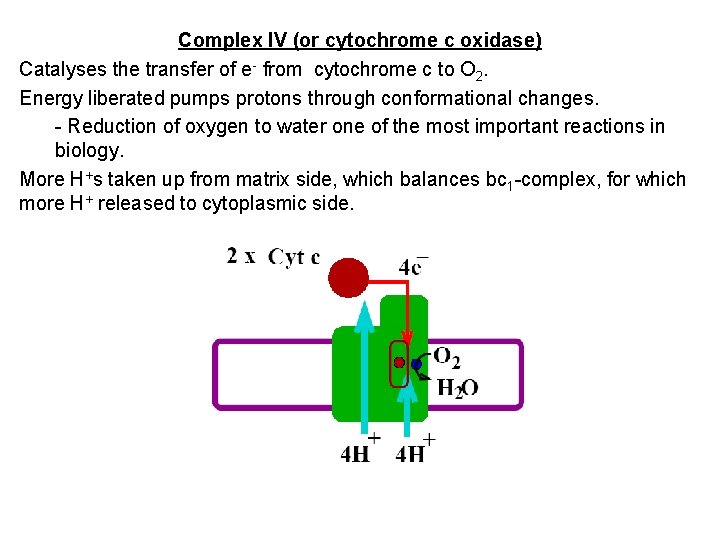
Complex IV (or cytochrome c oxidase) Catalyses the transfer of e- from cytochrome c to O 2. Energy liberated pumps protons through conformational changes. - Reduction of oxygen to water one of the most important reactions in biology. More H+s taken up from matrix side, which balances bc 1 -complex, for which more H+ released to cytoplasmic side.
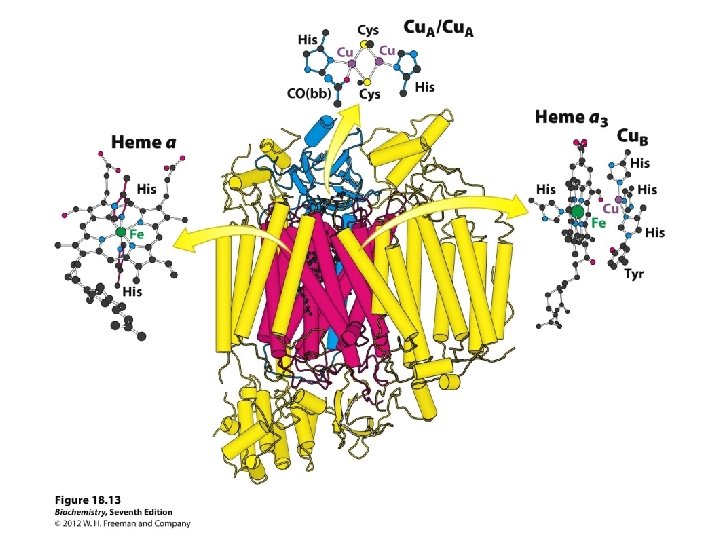
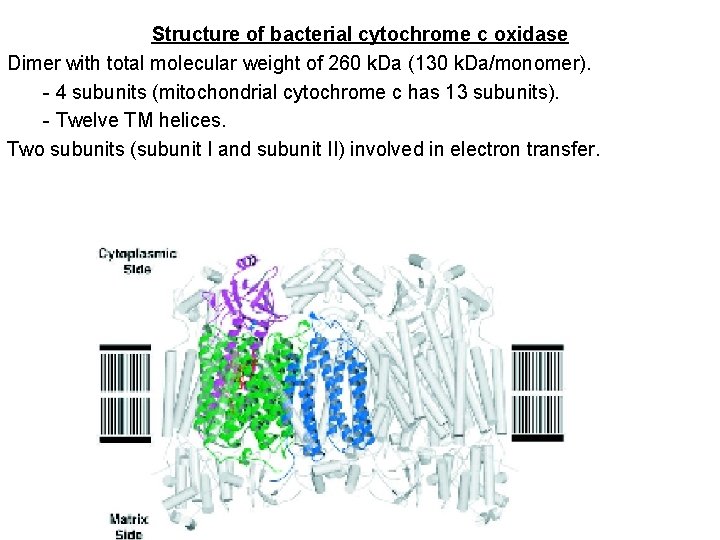
Structure of bacterial cytochrome c oxidase Dimer with total molecular weight of 260 k. Da (130 k. Da/monomer). - 4 subunits (mitochondrial cytochrome c has 13 subunits). - Twelve TM helices. Two subunits (subunit I and subunit II) involved in electron transfer.
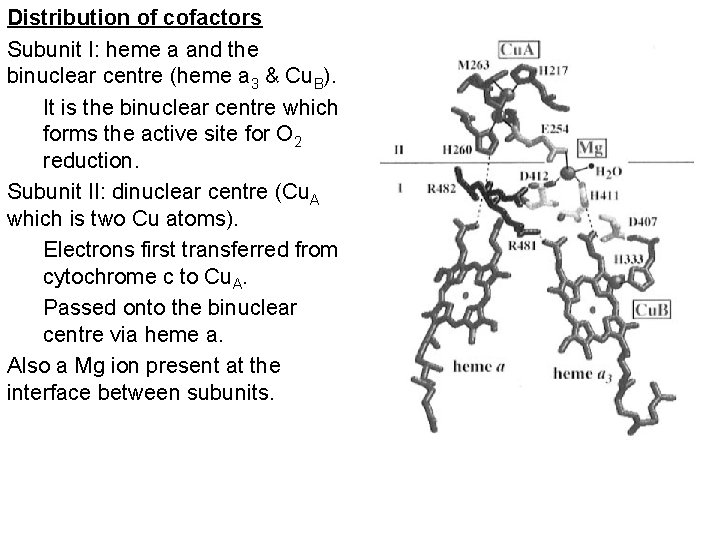
Distribution of cofactors Subunit I: heme a and the binuclear centre (heme a 3 & Cu. B). It is the binuclear centre which forms the active site for O 2 reduction. Subunit II: dinuclear centre (Cu. A which is two Cu atoms). Electrons first transferred from cytochrome c to Cu. A. Passed onto the binuclear centre via heme a. Also a Mg ion present at the interface between subunits.
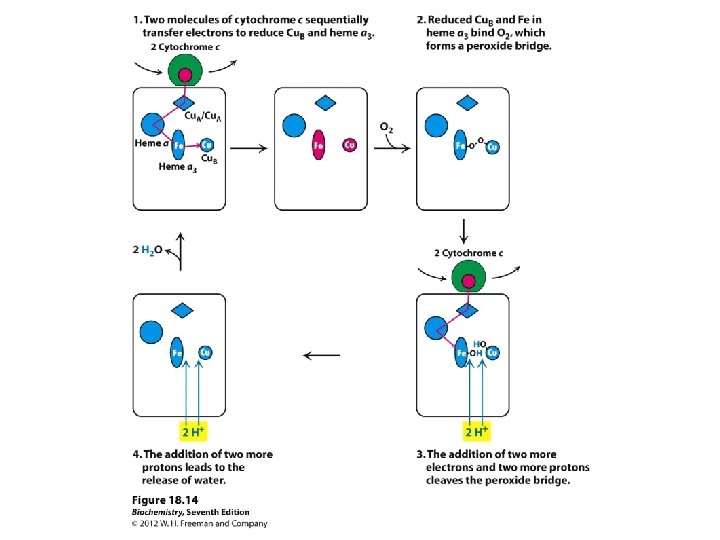
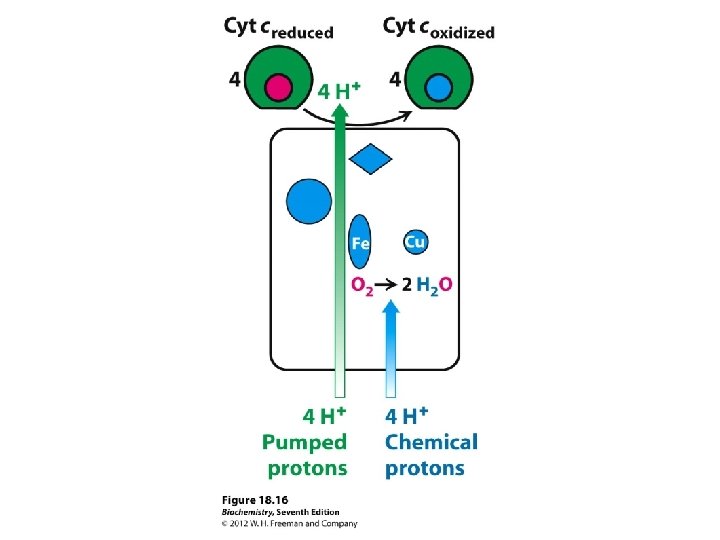
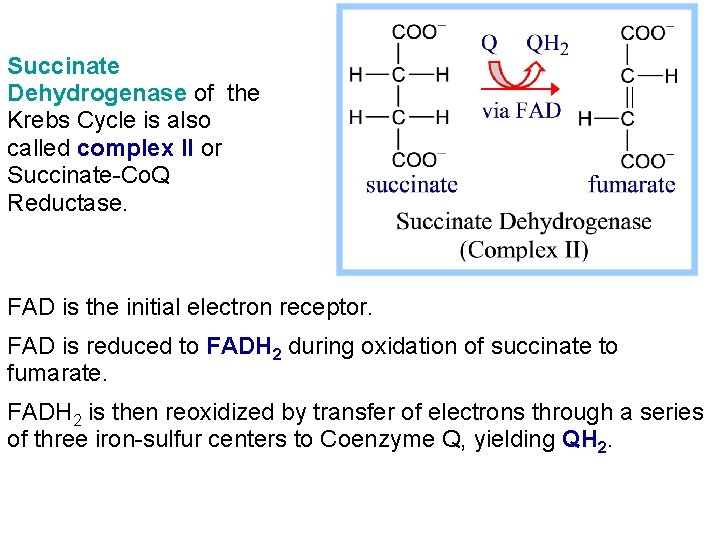
Succinate Dehydrogenase of the Krebs Cycle is also called complex II or Succinate-Co. Q Reductase. FAD is the initial electron receptor. FAD is reduced to FADH 2 during oxidation of succinate to fumarate. FADH 2 is then reoxidized by transfer of electrons through a series of three iron-sulfur centers to Coenzyme Q, yielding QH 2.
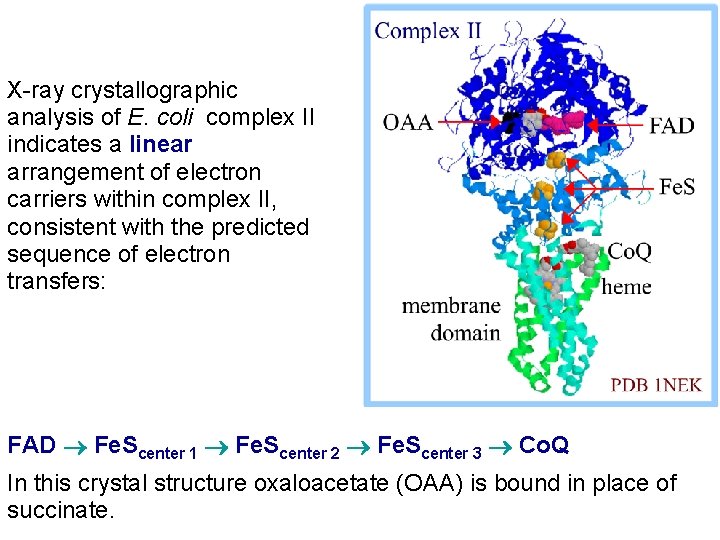
X-ray crystallographic analysis of E. coli complex II indicates a linear arrangement of electron carriers within complex II, consistent with the predicted sequence of electron transfers: FAD Fe. Scenter 1 Fe. Scenter 2 Fe. Scenter 3 Co. Q In this crystal structure oxaloacetate (OAA) is bound in place of succinate.
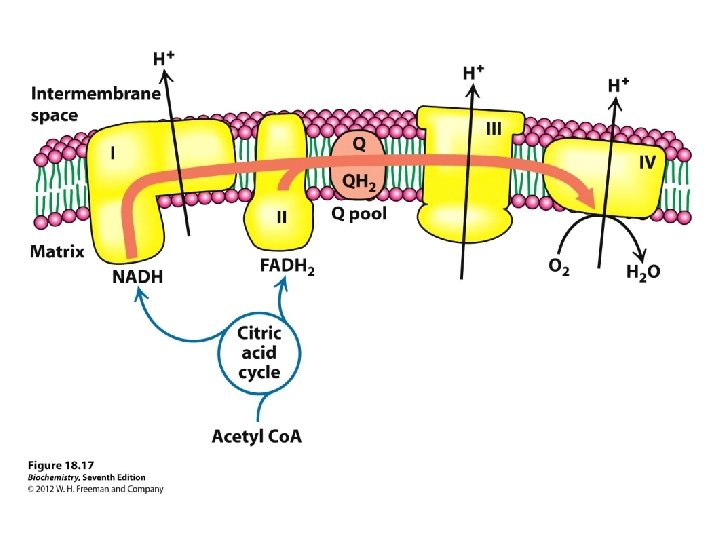
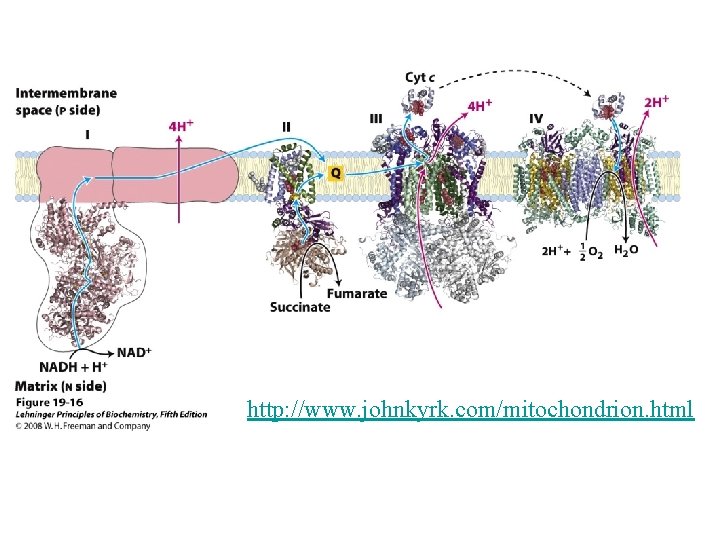
http: //www. johnkyrk. com/mitochondrion. html
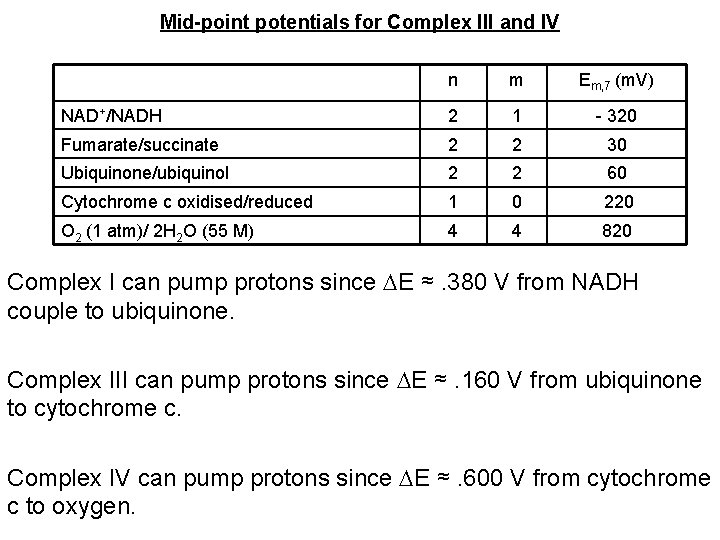
Mid-point potentials for Complex III and IV n m Em, 7 (m. V) NAD+/NADH 2 1 - 320 Fumarate/succinate 2 2 30 Ubiquinone/ubiquinol 2 2 60 Cytochrome c oxidised/reduced 1 0 220 O 2 (1 atm)/ 2 H 2 O (55 M) 4 4 820 Complex I can pump protons since E ≈. 380 V from NADH couple to ubiquinone. Complex III can pump protons since E ≈. 160 V from ubiquinone to cytochrome c. Complex IV can pump protons since E ≈. 600 V from cytochrome c to oxygen.
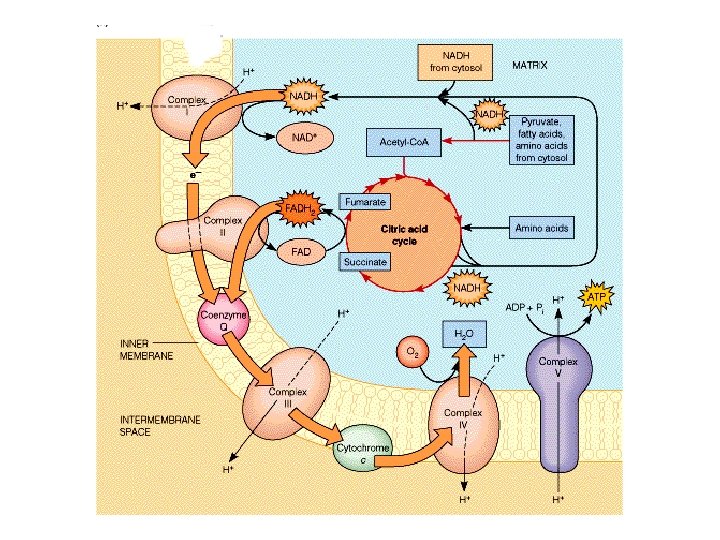
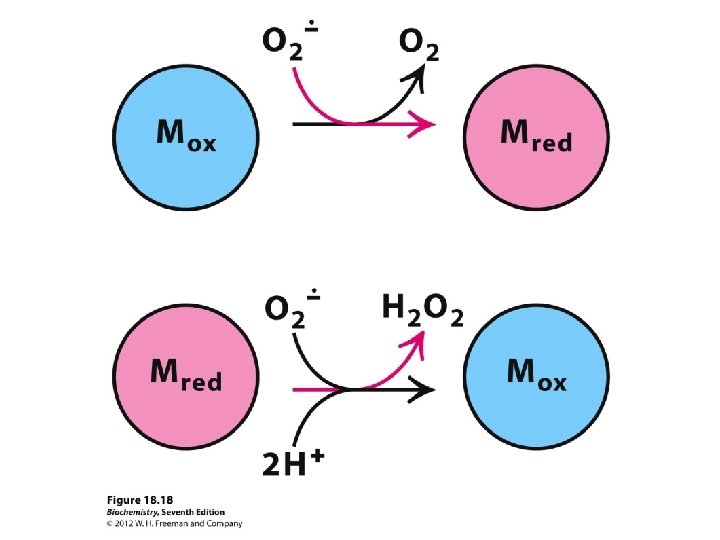
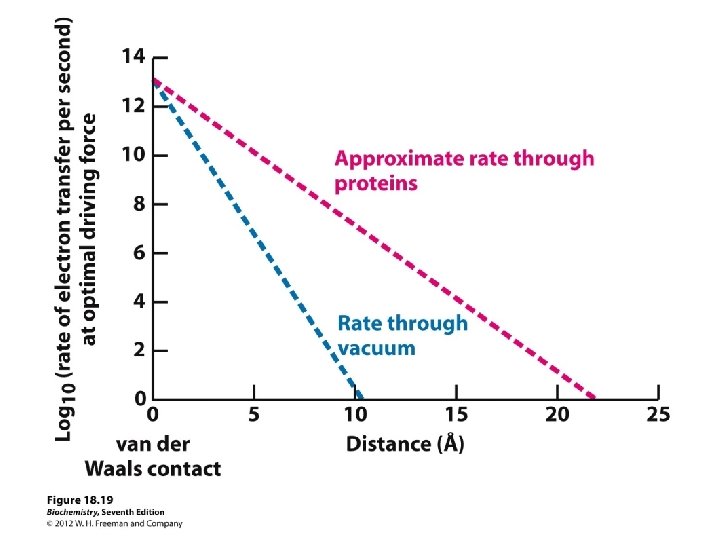
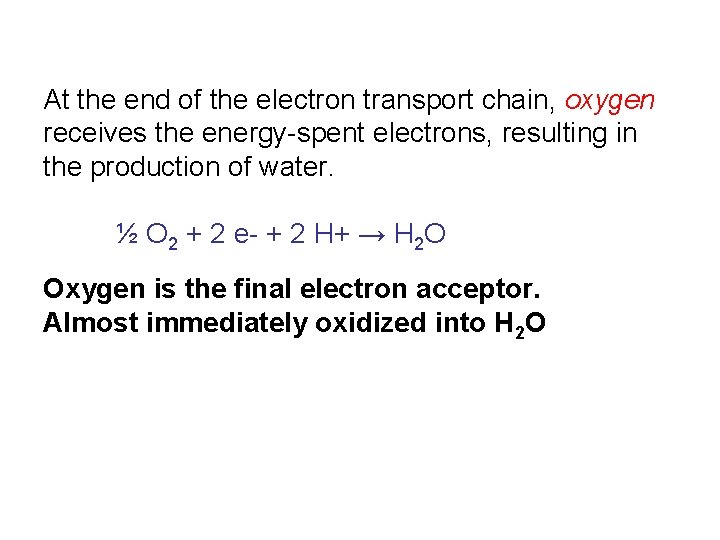
At the end of the electron transport chain, oxygen receives the energy-spent electrons, resulting in the production of water. ½ O 2 + 2 e- + 2 H+ → H 2 O Oxygen is the final electron acceptor. Almost immediately oxidized into H 2 O
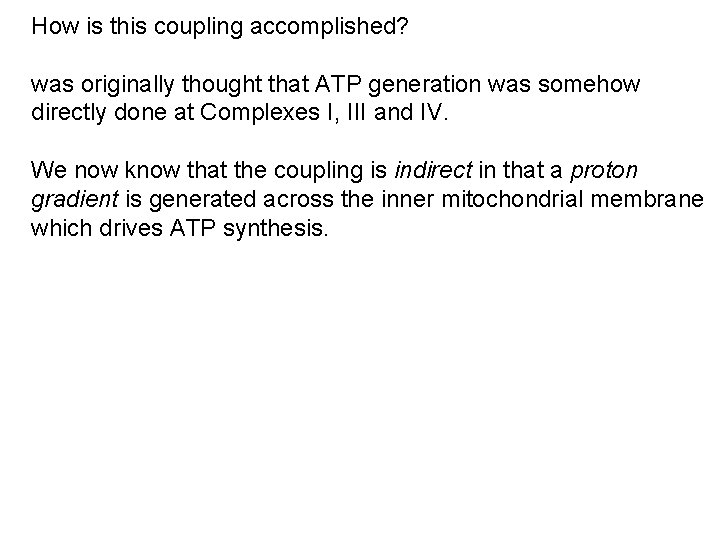
How is this coupling accomplished? was originally thought that ATP generation was somehow directly done at Complexes I, III and IV. We now know that the coupling is indirect in that a proton gradient is generated across the inner mitochondrial membrane which drives ATP synthesis.
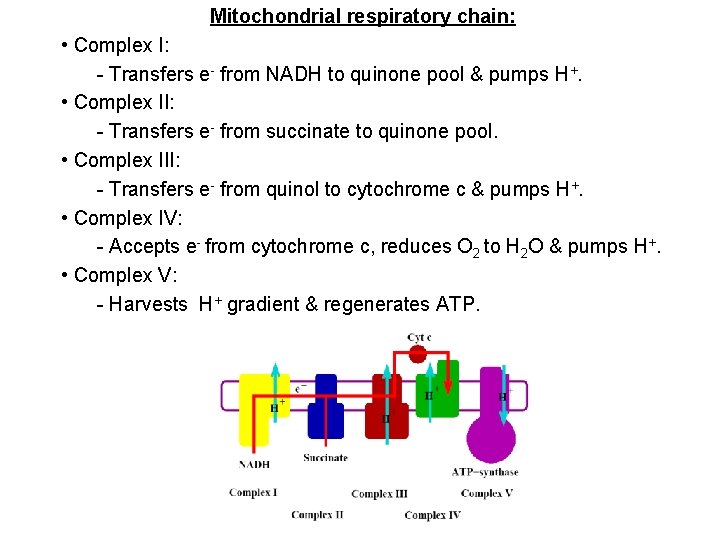
Mitochondrial respiratory chain: • Complex I: - Transfers e- from NADH to quinone pool & pumps H+. • Complex II: - Transfers e- from succinate to quinone pool. • Complex III: - Transfers e- from quinol to cytochrome c & pumps H+. • Complex IV: - Accepts e- from cytochrome c, reduces O 2 to H 2 O & pumps H+. • Complex V: - Harvests H+ gradient & regenerates ATP.