Radio Galaxies Part 1 Raffaella Morganti ASTRON The
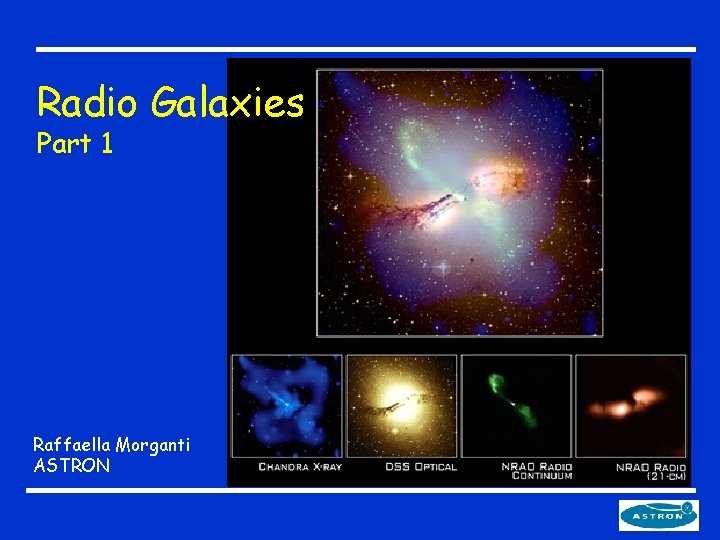
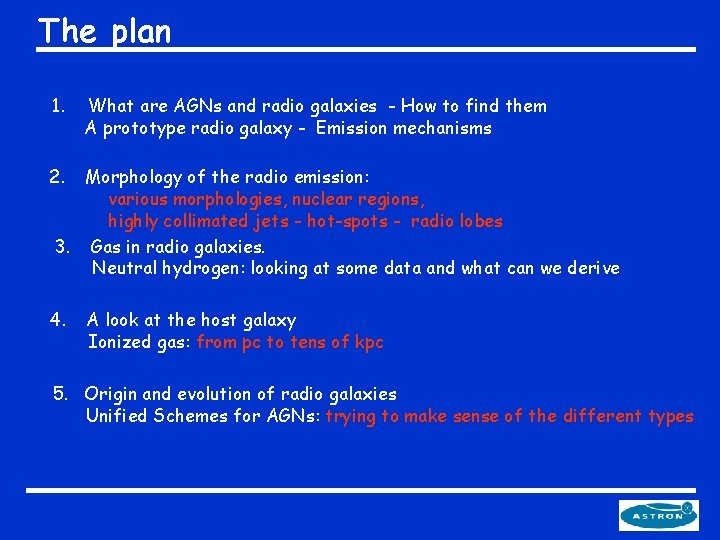
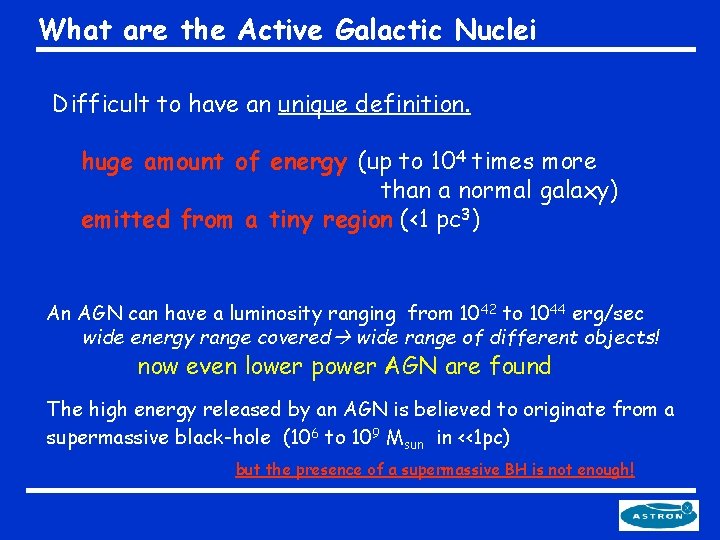
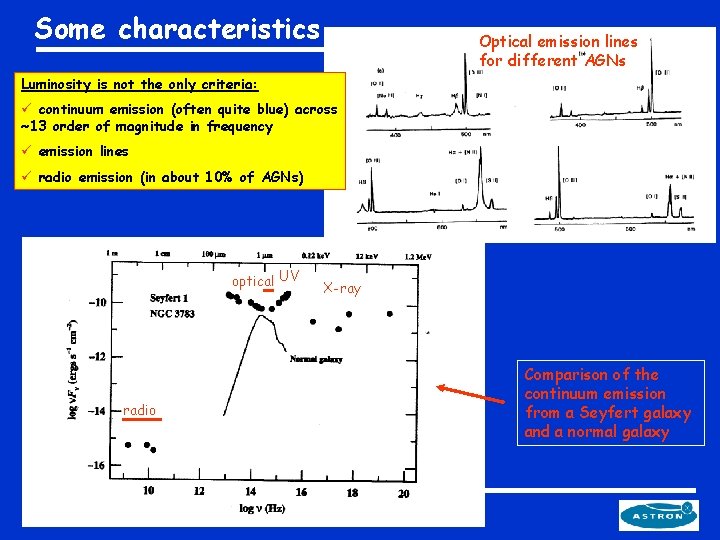
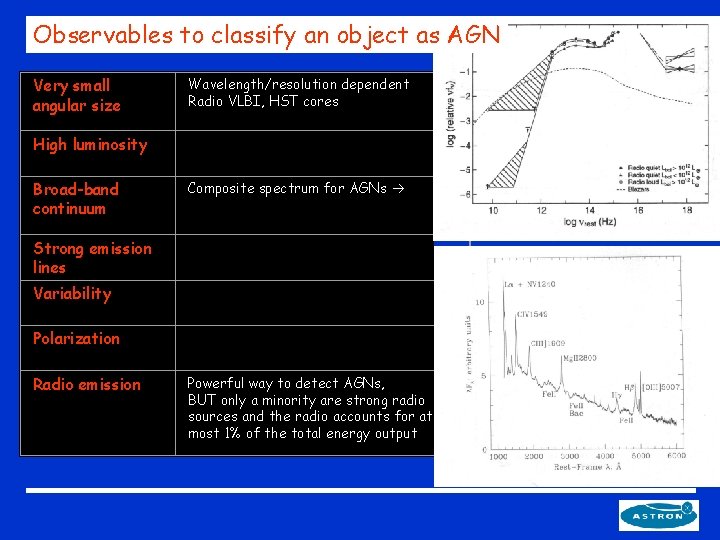
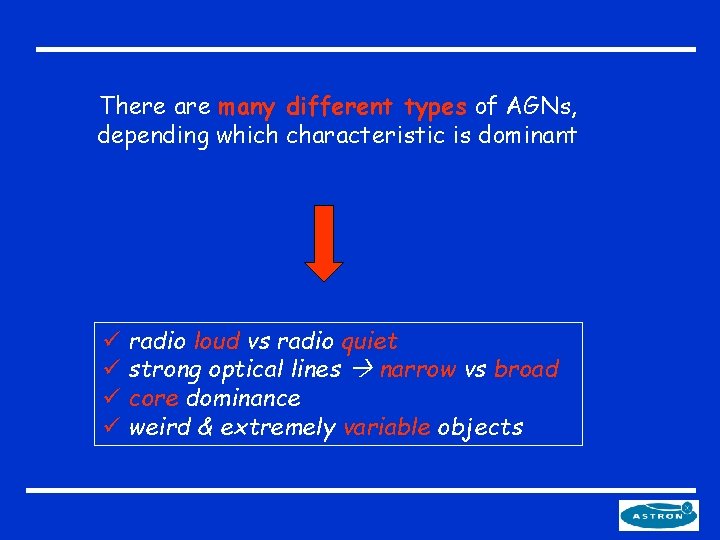
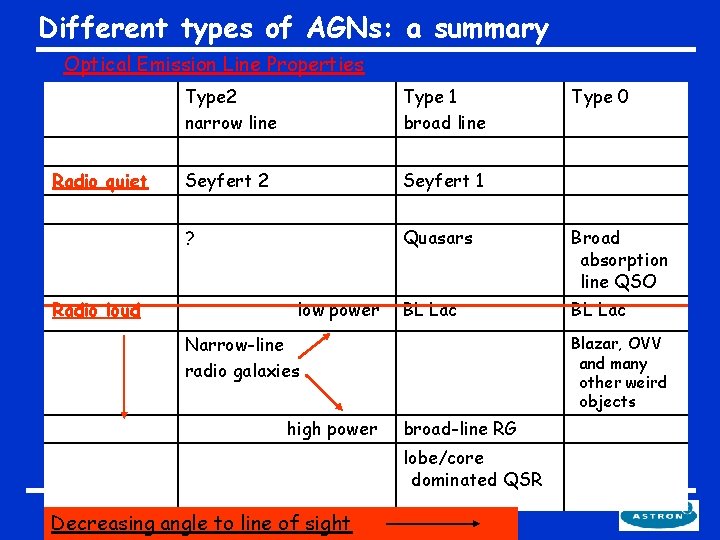
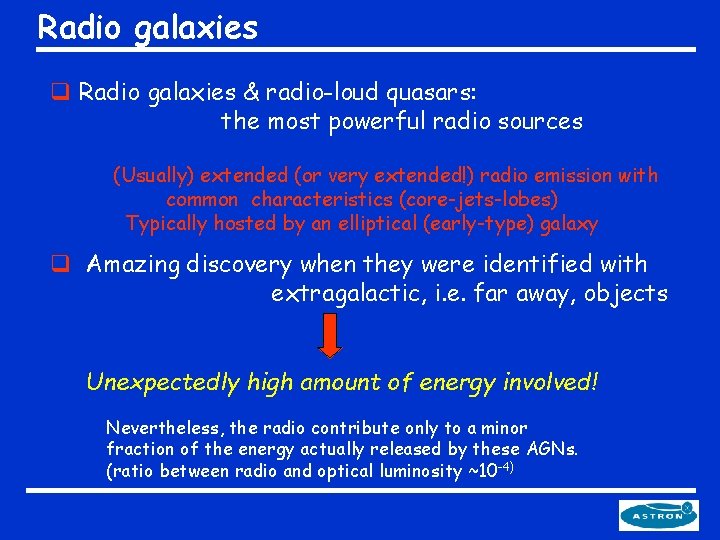
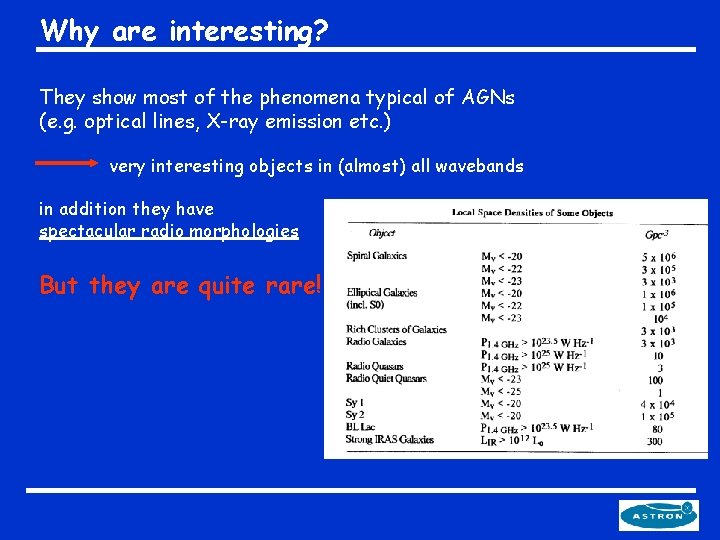
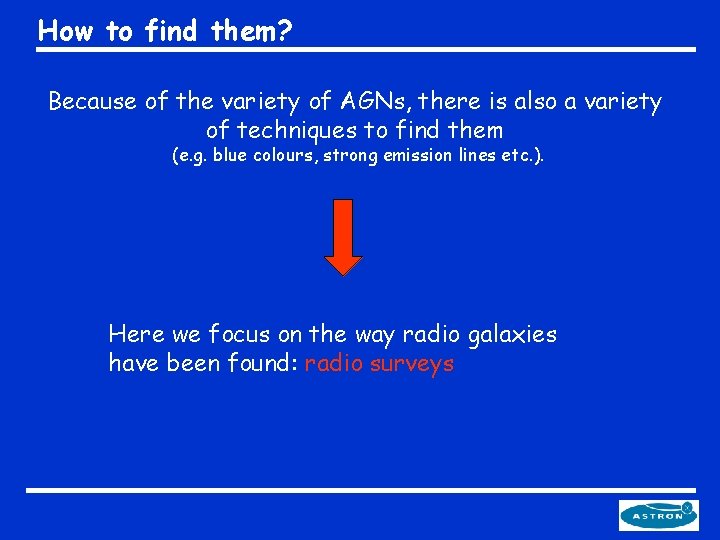
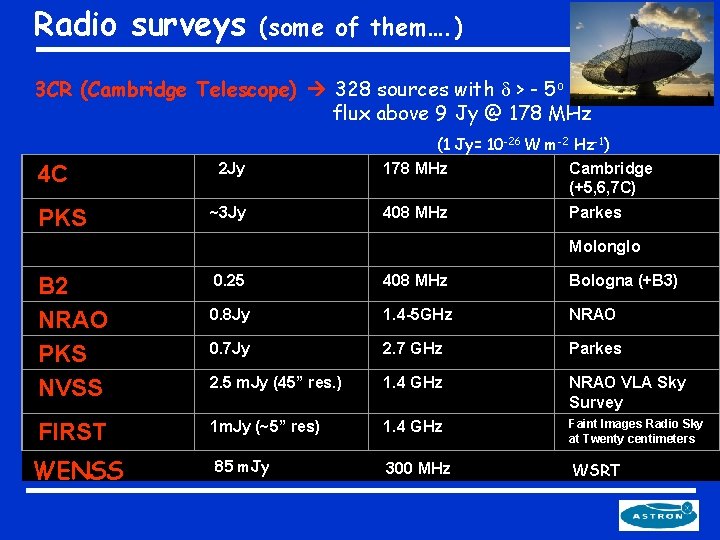
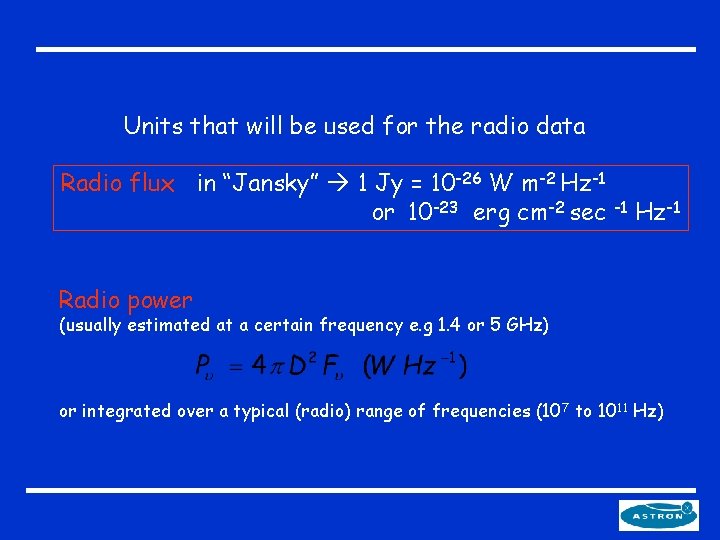
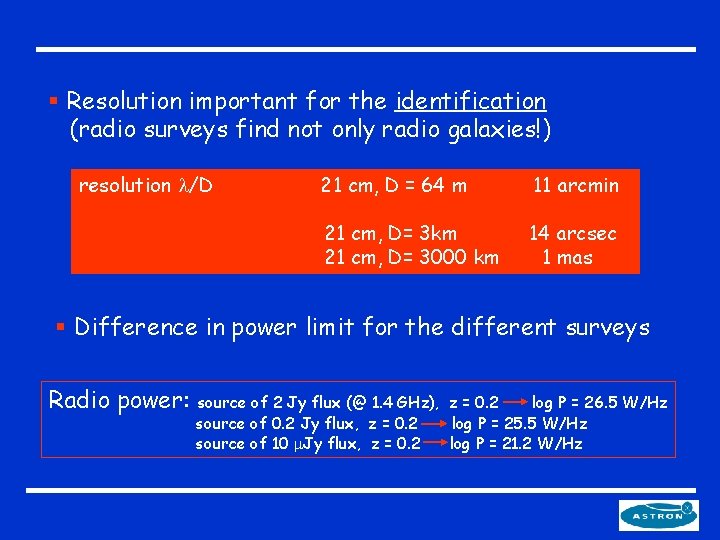
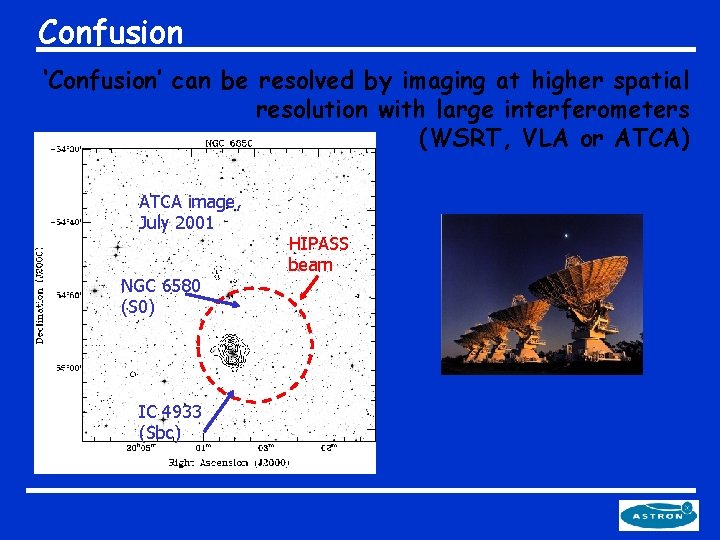
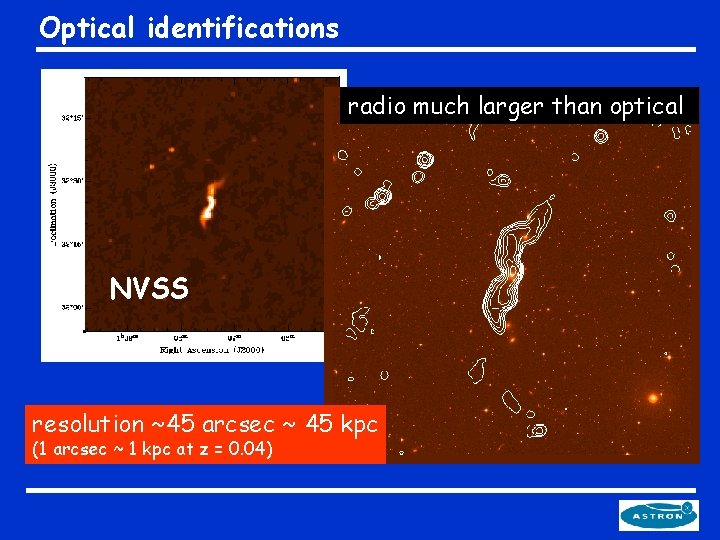
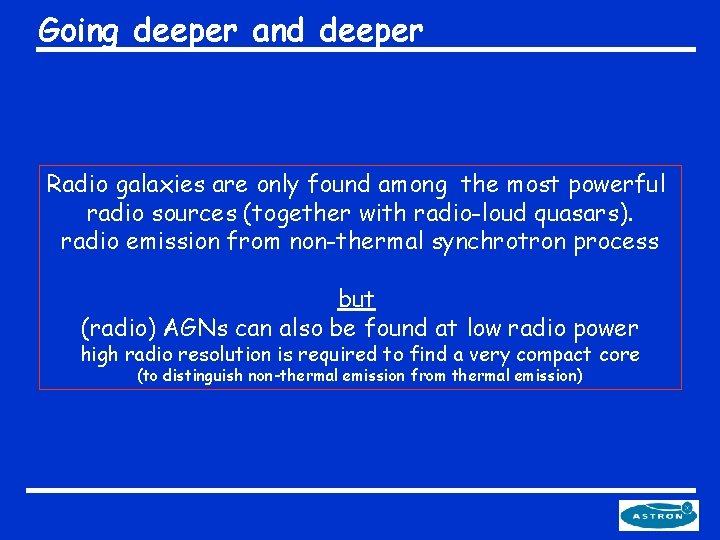
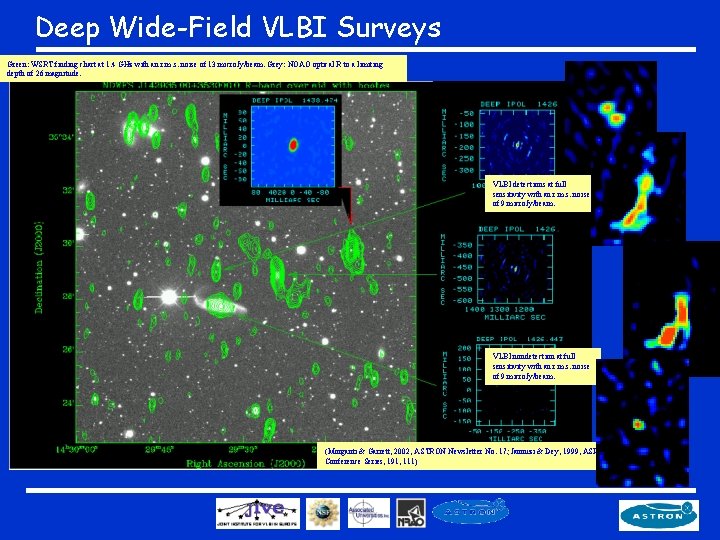
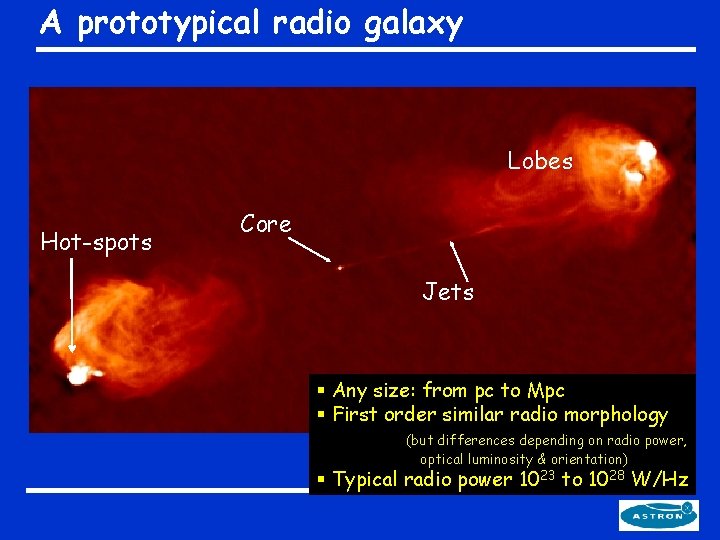
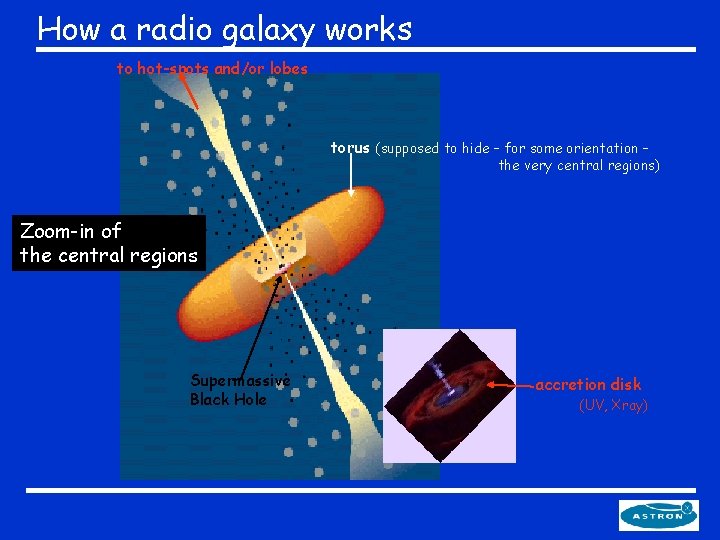
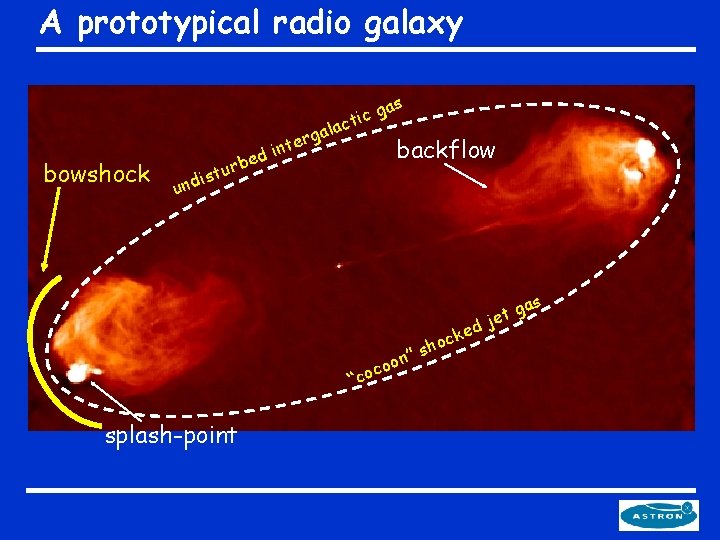
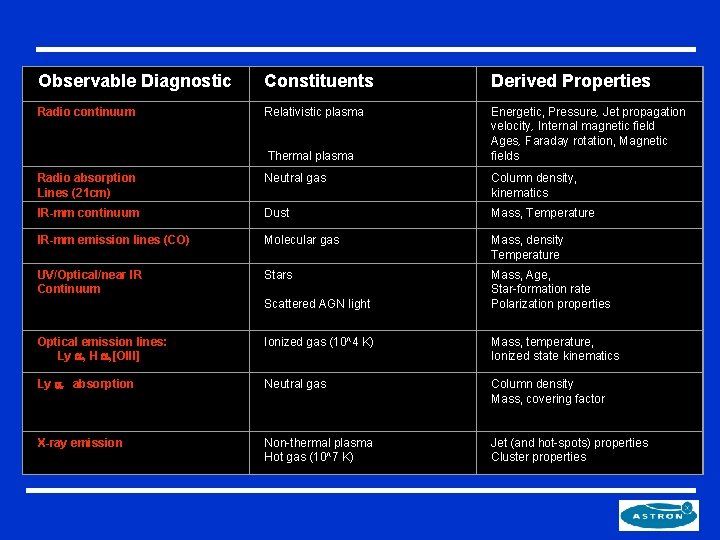
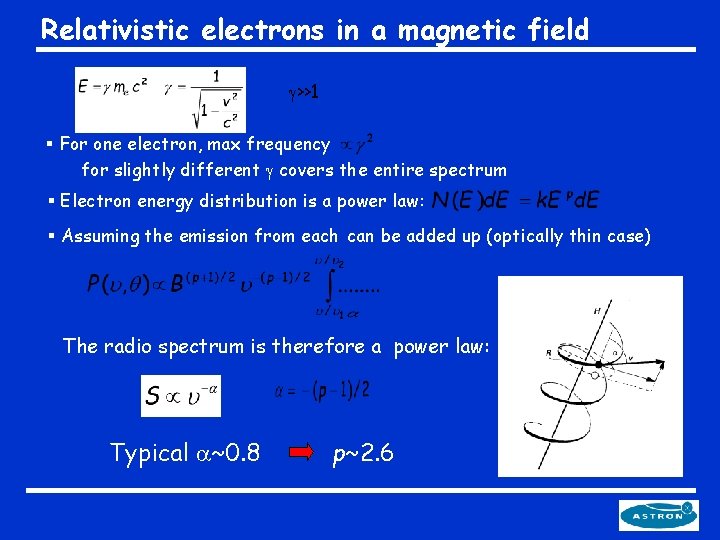
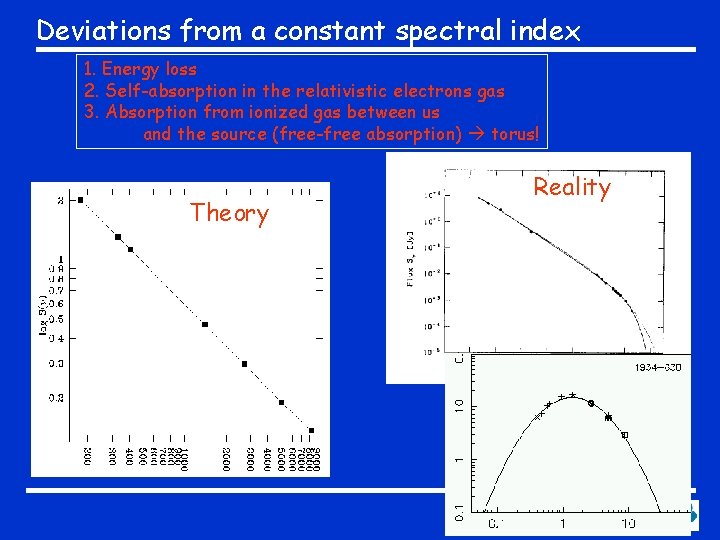
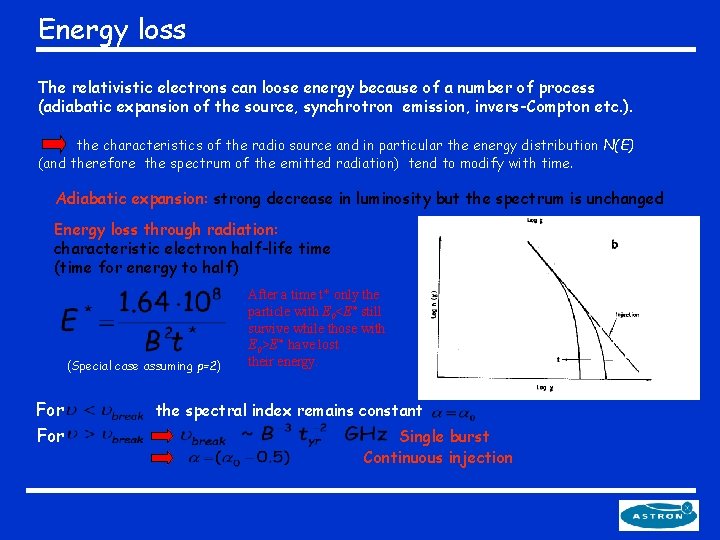
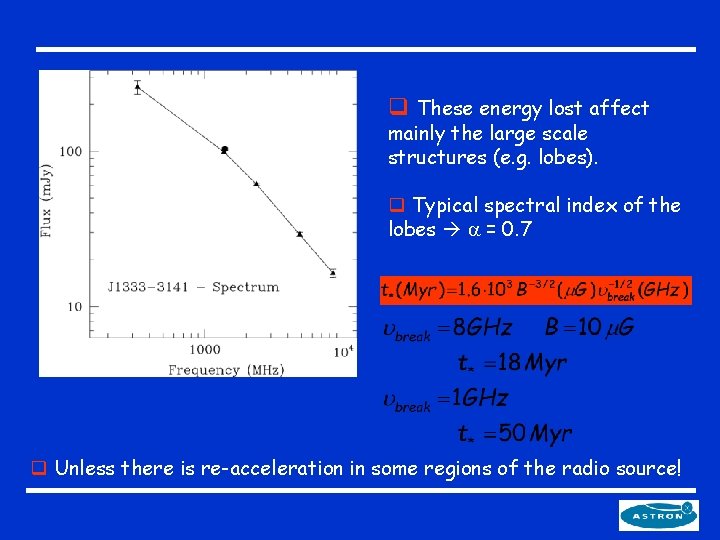
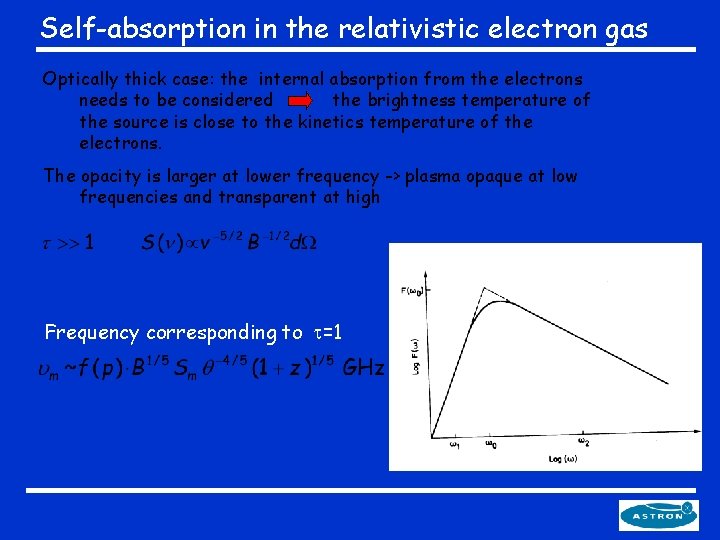
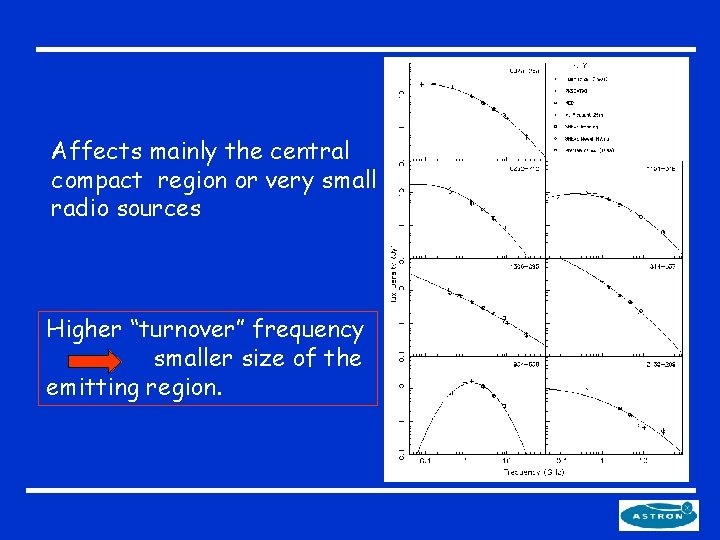
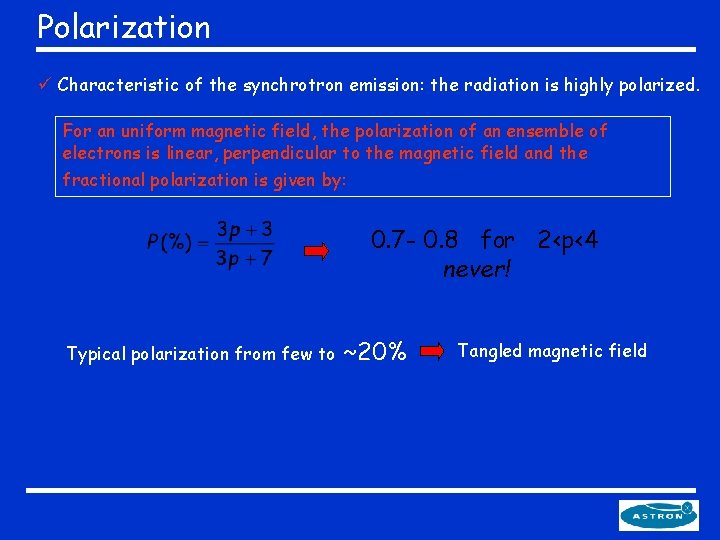
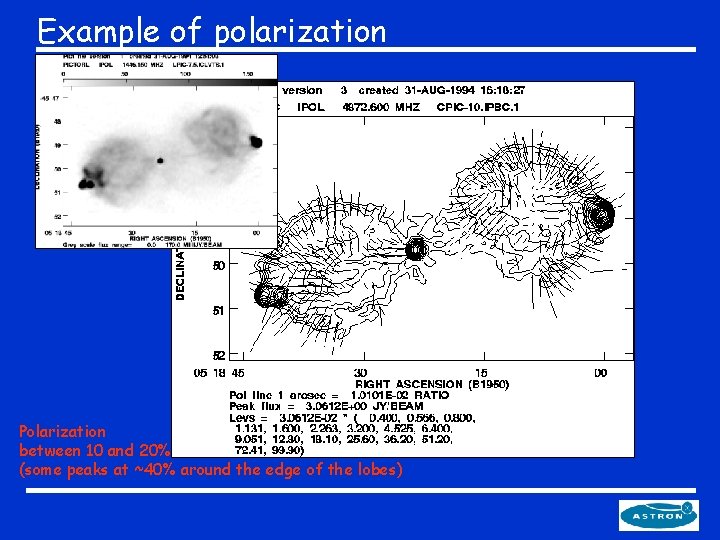
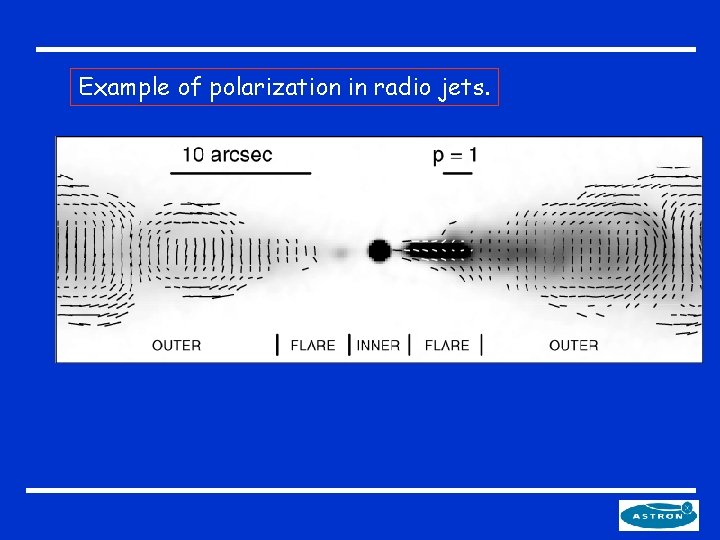
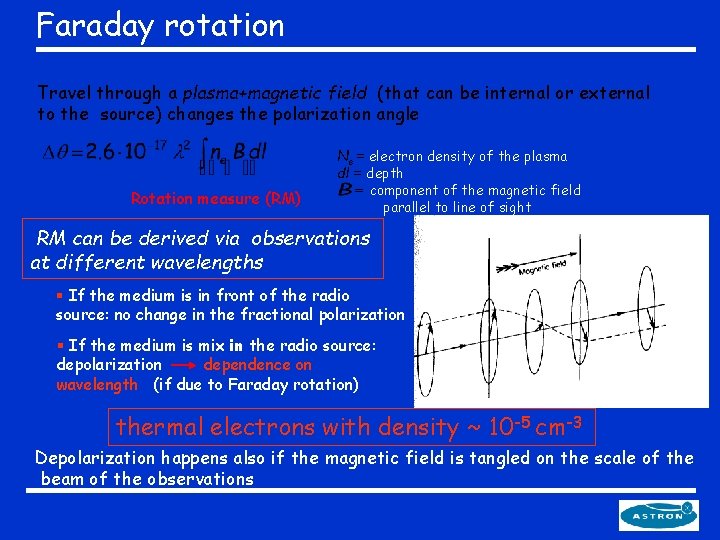
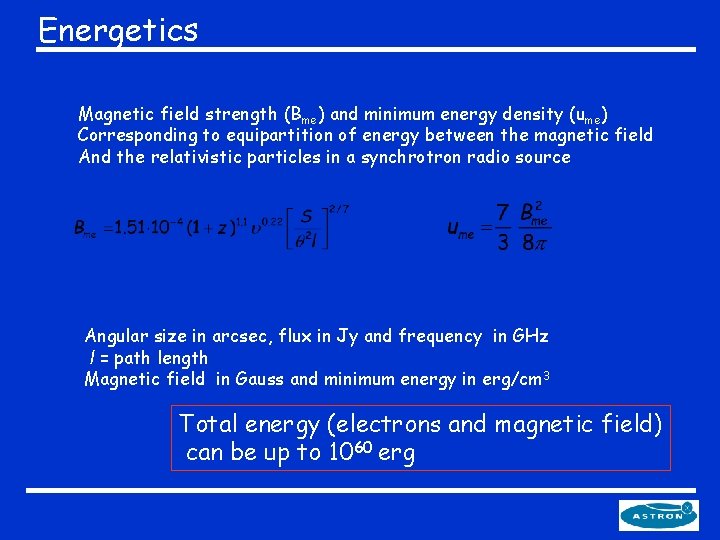
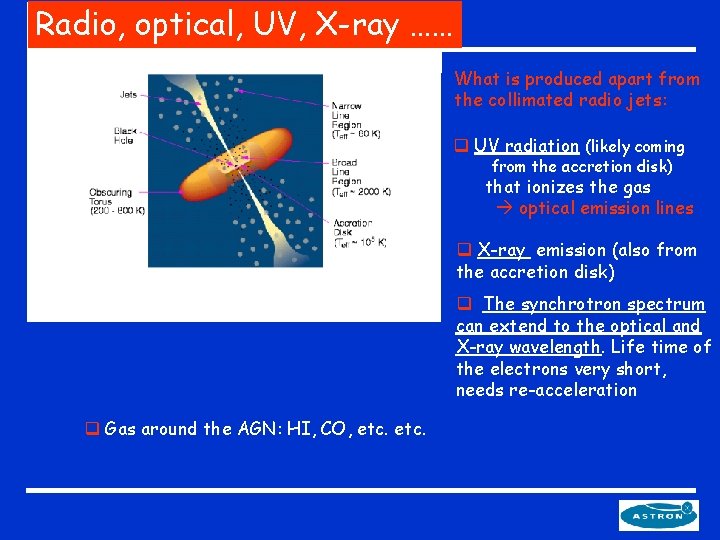
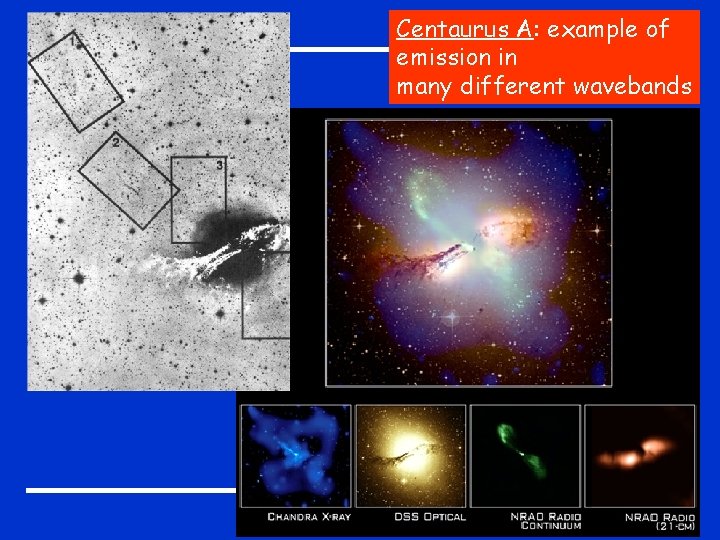
- Slides: 34
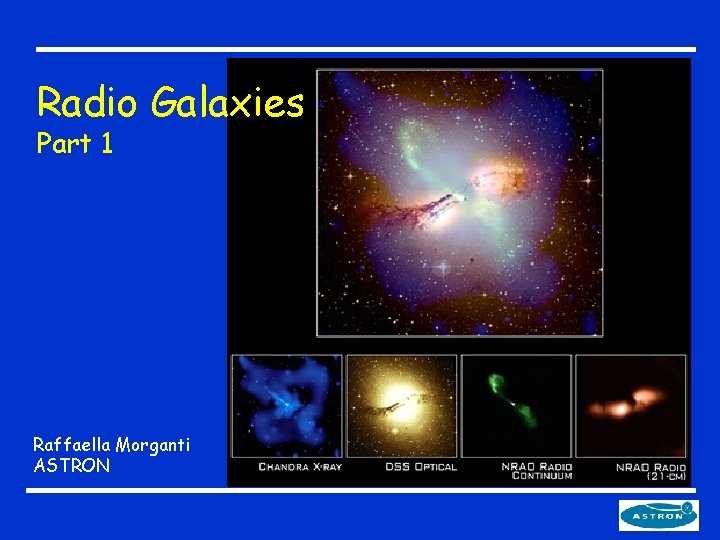
Radio Galaxies Part 1 Raffaella Morganti ASTRON
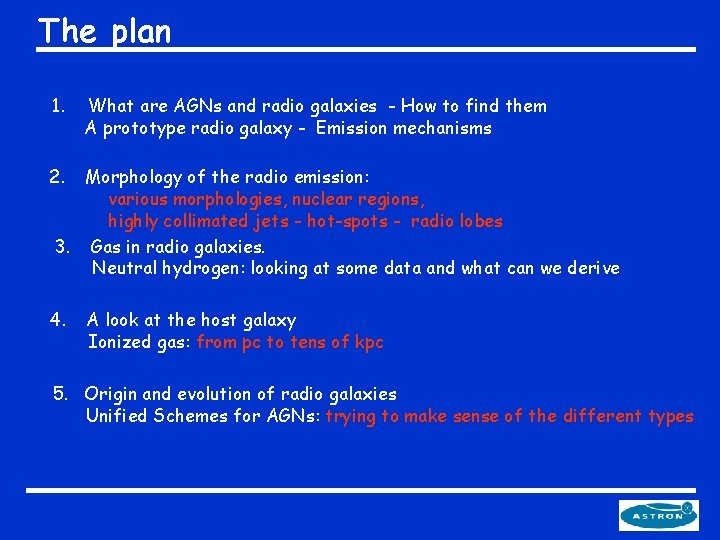
The plan 1. What are AGNs and radio galaxies - How to find them A prototype radio galaxy - Emission mechanisms 2. Morphology of the radio emission: various morphologies, nuclear regions, highly collimated jets - hot-spots - radio lobes 3. Gas in radio galaxies. Neutral hydrogen: looking at some data and what can we derive 4. A look at the host galaxy Ionized gas: from pc to tens of kpc 5. Origin and evolution of radio galaxies Unified Schemes for AGNs: trying to make sense of the different types
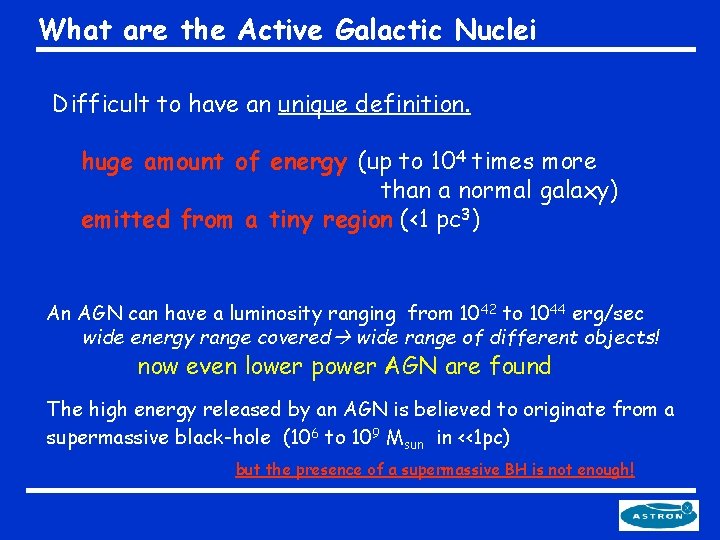
What are the Active Galactic Nuclei Difficult to have an unique definition. huge amount of energy (up to 104 times more than a normal galaxy) emitted from a tiny region (<1 pc 3) An AGN can have a luminosity ranging from 1042 to 1044 erg/sec wide energy range covered wide range of different objects! now even lower power AGN are found The high energy released by an AGN is believed to originate from a supermassive black-hole (106 to 109 Msun in <<1 pc) but the presence of a supermassive BH is not enough!
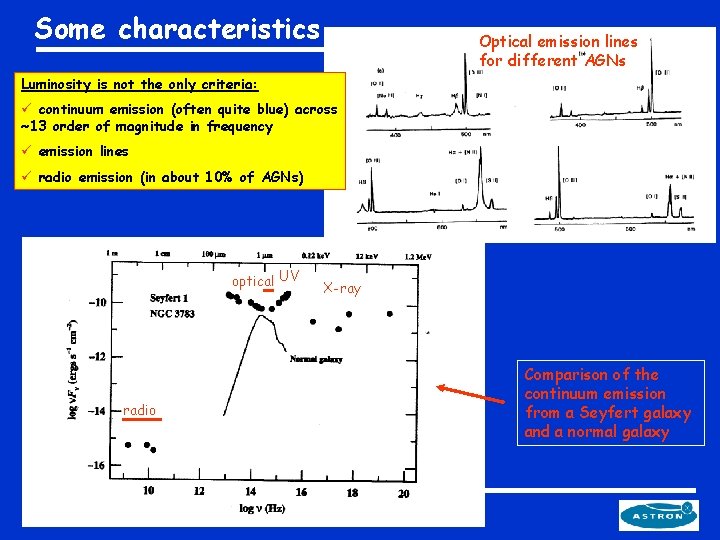
Some characteristics Optical emission lines for different AGNs Luminosity is not the only criteria: ü continuum emission (often quite blue) across ~13 order of magnitude in frequency ü emission lines ü radio emission (in about 10% of AGNs) optical UV radio X-ray Comparison of the continuum emission from a Seyfert galaxy and a normal galaxy
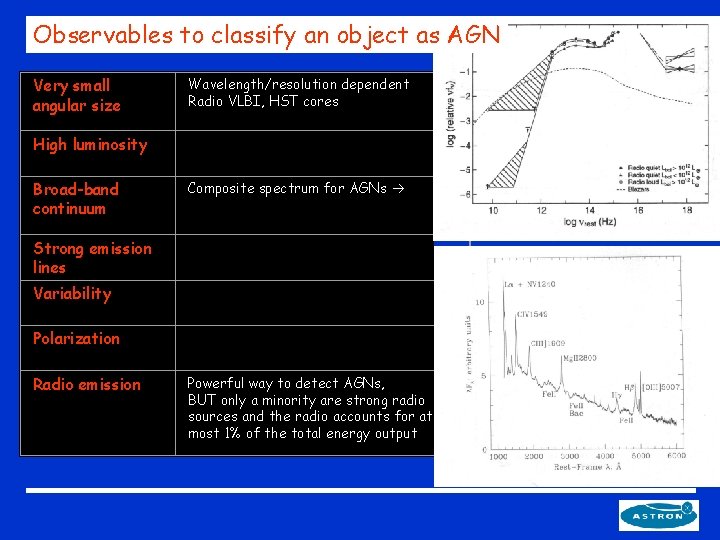
Observables to classify an object as AGN Very small angular size Wavelength/resolution dependent Radio VLBI, HST cores High luminosity Broad-band continuum Composite spectrum for AGNs Strong emission lines Variability Polarization Radio emission Powerful way to detect AGNs, BUT only a minority are strong radio sources and the radio accounts for at most 1% of the total energy output
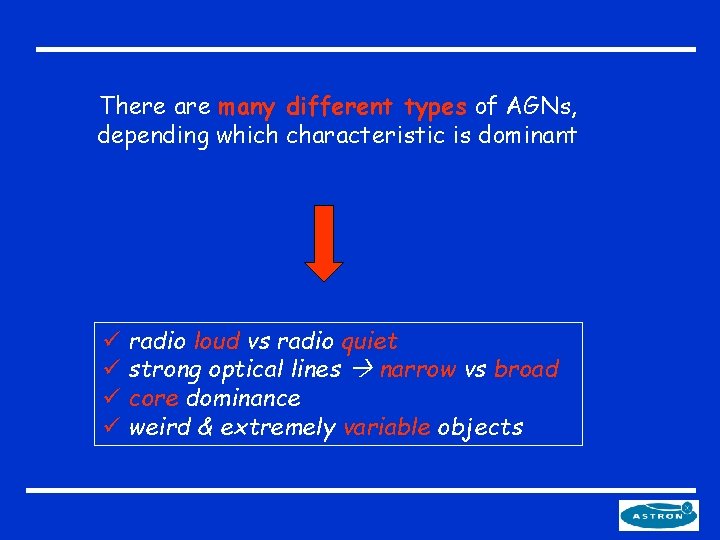
There are many different types of AGNs, depending which characteristic is dominant ü radio loud vs radio quiet ü strong optical lines narrow vs broad ü core dominance ü weird & extremely variable objects
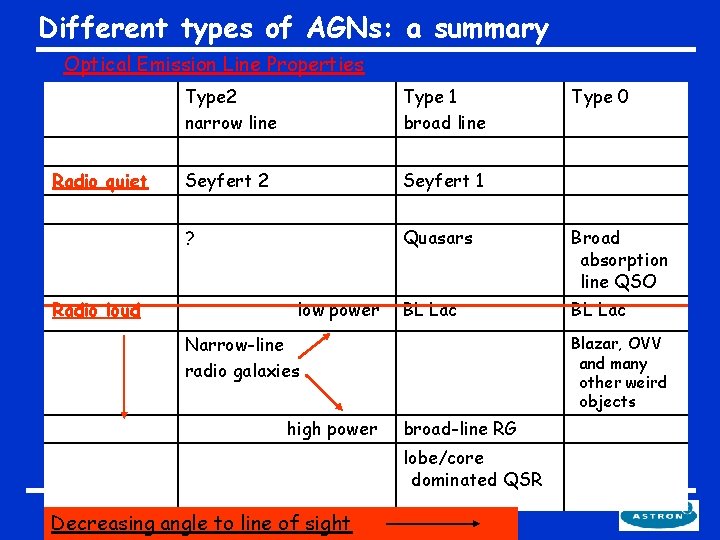
Different types of AGNs: a summary Optical Emission Line Properties Radio quiet Radio loud Type 2 narrow line Type 1 broad line Seyfert 2 Seyfert 1 ? Quasars Broad absorption line QSO BL Lac low power Narrow-line radio galaxies high power Blazar, OVV and many other weird objects broad-line RG lobe/core dominated QSR Decreasing angle to line of sight Type 0
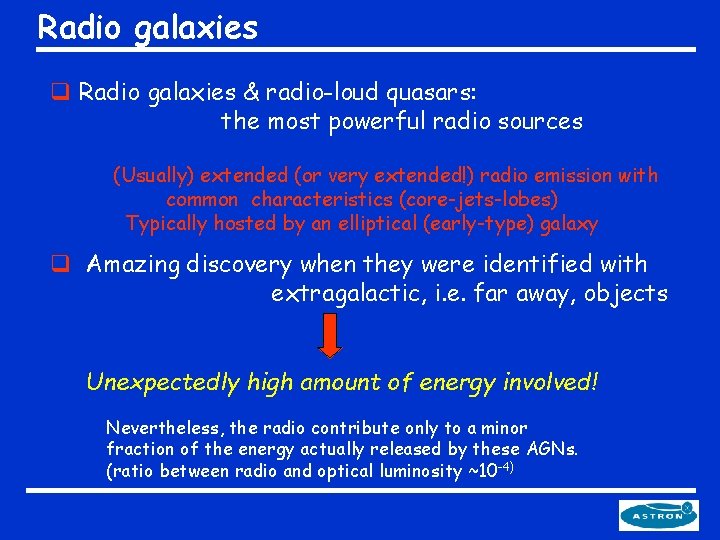
Radio galaxies q Radio galaxies & radio-loud quasars: the most powerful radio sources (Usually) extended (or very extended!) radio emission with common characteristics (core-jets-lobes) Typically hosted by an elliptical (early-type) galaxy q Amazing discovery when they were identified with extragalactic, i. e. far away, objects Unexpectedly high amount of energy involved! Nevertheless, the radio contribute only to a minor fraction of the energy actually released by these AGNs. (ratio between radio and optical luminosity ~10 -4)
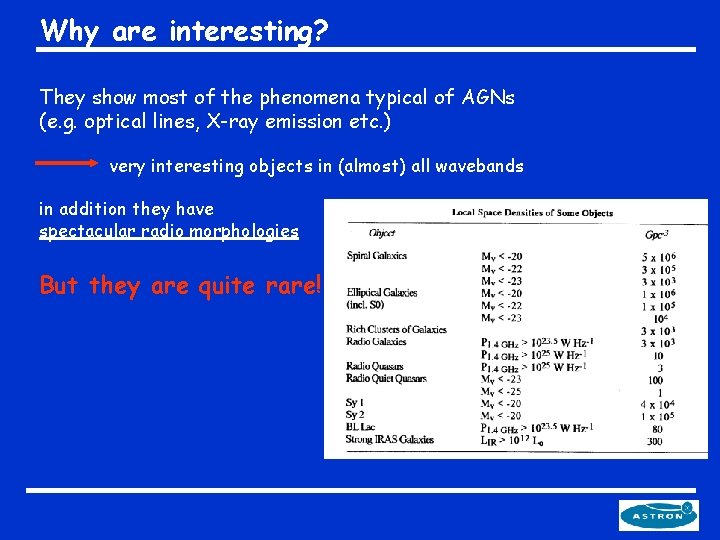
Why are interesting? They show most of the phenomena typical of AGNs (e. g. optical lines, X-ray emission etc. ) very interesting objects in (almost) all wavebands in addition they have spectacular radio morphologies But they are quite rare!
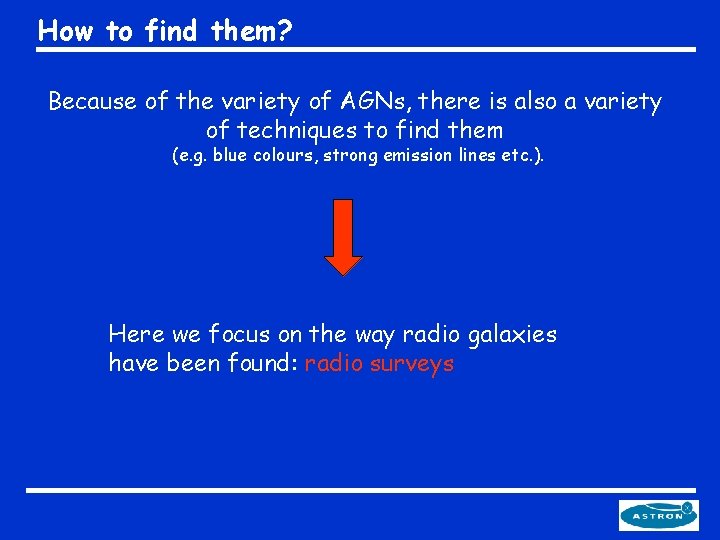
How to find them? Because of the variety of AGNs, there is also a variety of techniques to find them (e. g. blue colours, strong emission lines etc. ). Here we focus on the way radio galaxies have been found: radio surveys
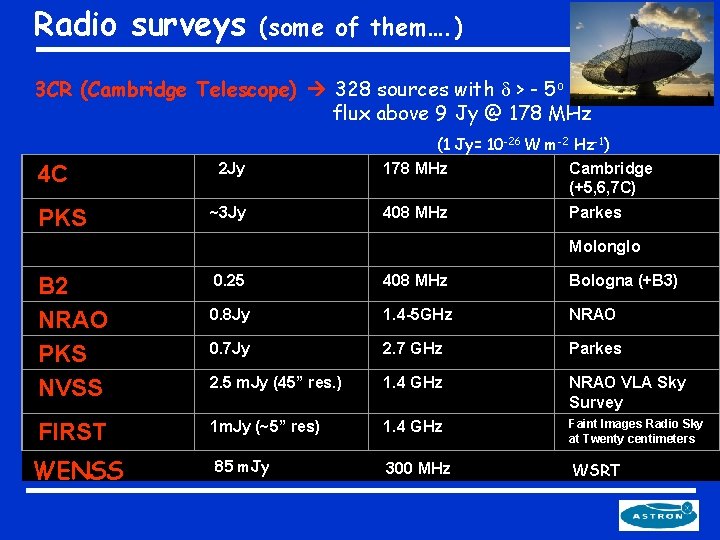
Radio surveys (some of them…. ) 3 CR (Cambridge Telescope) 328 sources with > - 5 o flux above 9 Jy @ 178 MHz (1 Jy= 10 -26 W m-2 Hz-1) 2 Jy 178 MHz Cambridge (+5, 6, 7 C) PKS ~3 Jy 408 MHz Parkes Molonglo 0. 25 408 MHz Bologna (+B 3) 0. 8 Jy 1. 4 -5 GHz NRAO 0. 7 Jy 2. 7 GHz Parkes 2. 5 m. Jy (45” res. ) 1. 4 GHz NRAO VLA Sky Survey 1 m. Jy (~5” res) 1. 4 GHz Faint Images Radio Sky at Twenty centimeters 85 m. Jy 300 MHz WSRT 4 C B 2 NRAO PKS NVSS FIRST WENSS
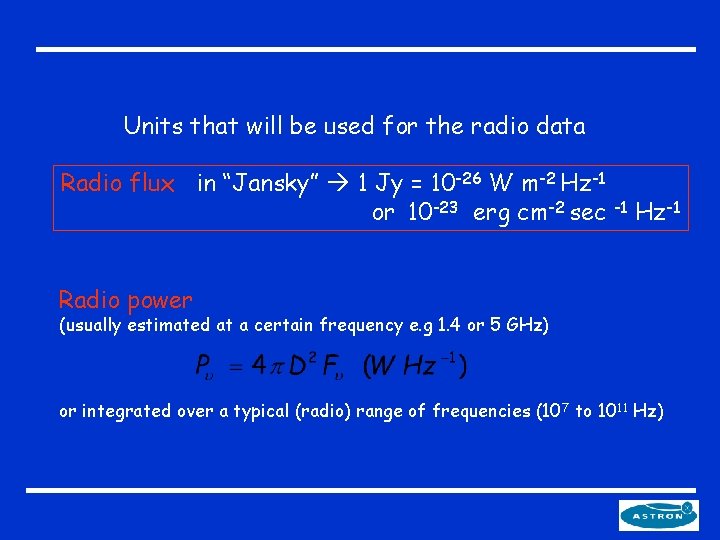
Units that will be used for the radio data Radio flux in “Jansky” 1 Jy = 10 -26 W m-2 Hz-1 or 10 -23 erg cm-2 sec -1 Hz-1 Radio power (usually estimated at a certain frequency e. g 1. 4 or 5 GHz) or integrated over a typical (radio) range of frequencies (10 7 to 1011 Hz)
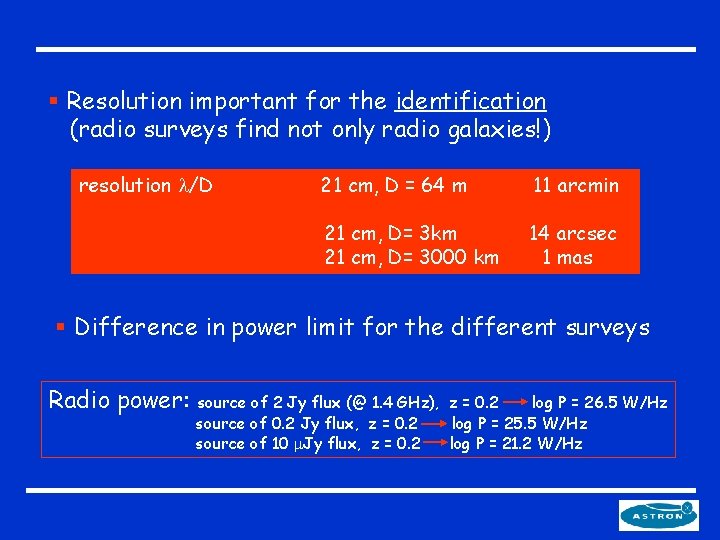
§ Resolution important for the identification (radio surveys find not only radio galaxies!) resolution /D 21 cm, D = 64 m 11 arcmin 21 cm, D= 3 km 21 cm, D= 3000 km 14 arcsec 1 mas § Difference in power limit for the different surveys Radio power: source of 2 Jy flux (@ 1. 4 GHz), z = 0. 2 log P = 26. 5 W/Hz source of 0. 2 Jy flux, z = 0. 2 log P = 25. 5 W/Hz source of 10 Jy flux, z = 0. 2 log P = 21. 2 W/Hz
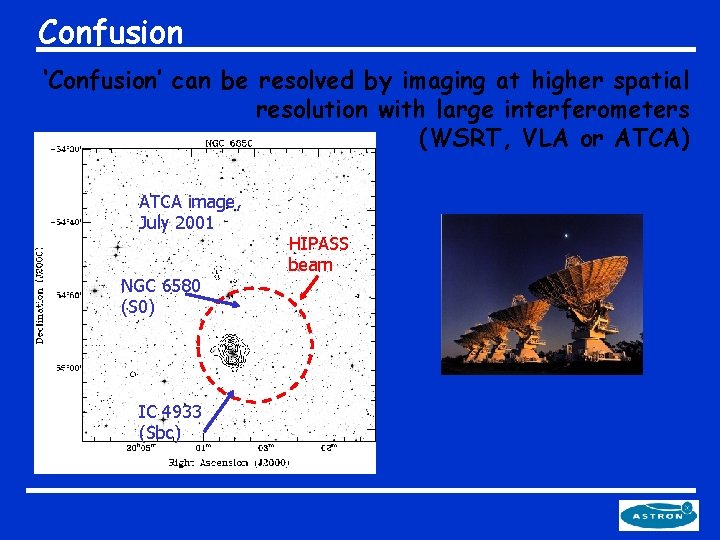
Confusion ‘Confusion’ can be resolved by imaging at higher spatial resolution with large interferometers (WSRT, VLA or ATCA) ATCA image, July 2001 NGC 6580 (S 0) IC 4933 (Sbc) HIPASS beam
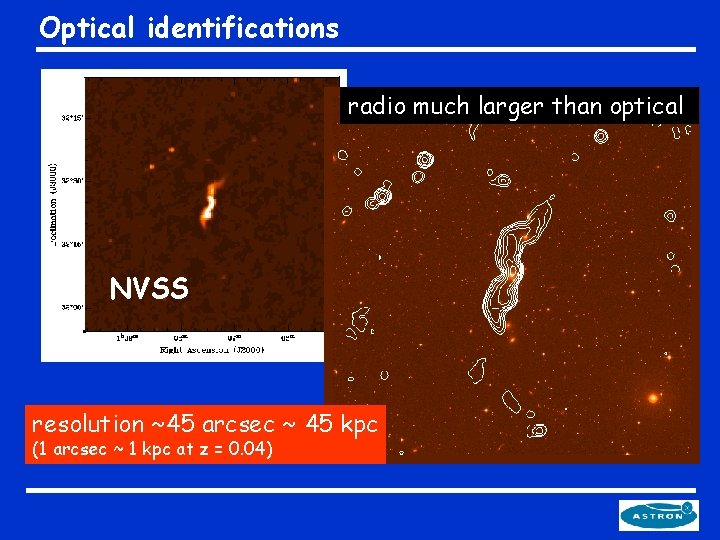
Optical identifications radio much larger than optical NVSS resolution ~45 arcsec ~ 45 kpc (1 arcsec ~ 1 kpc at z = 0. 04)
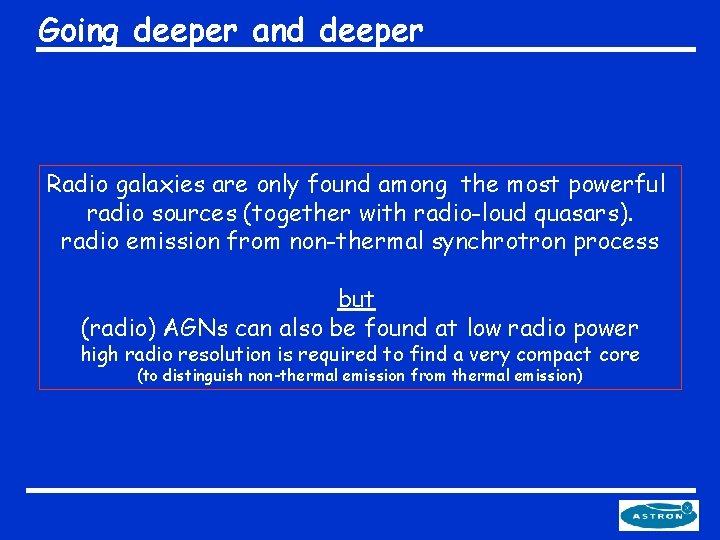
Going deeper and deeper Radio galaxies are only found among the most powerful radio sources (together with radio-loud quasars). radio emission from non-thermal synchrotron process but (radio) AGNs can also be found at low radio power high radio resolution is required to find a very compact core (to distinguish non-thermal emission from thermal emission)
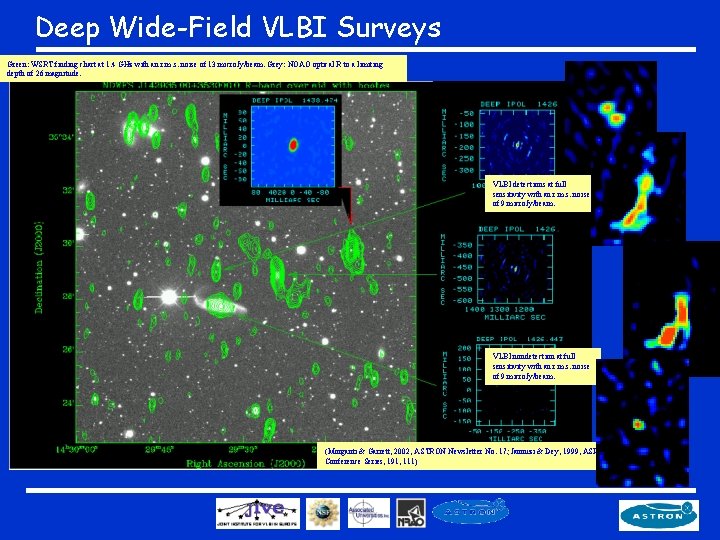
Deep Wide-Field VLBI Surveys Green: WSRT finding chart at 1. 4 GHz with an r. m. s. noise of 13 micro. Jy/beam. Grey: NOAO optical R to a limiting depth of 26 magnitude. VLBI detections at full sensitivity with an r. m. s. noise of 9 micro. Jy/beam. VLBI nondetection at full sensitivity with an r. m. s. noise of 9 micro. Jy/beam. (Morganti & Garrett, 2002, ASTRON Newsletter No. 17; Jannuzi & Dey, 1999, ASP Conference Series, 191, 111)
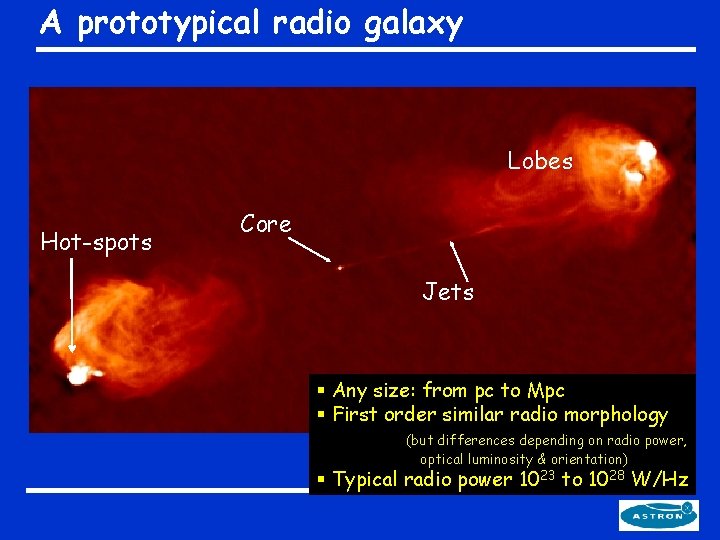
A prototypical radio galaxy Lobes Hot-spots Core Jets § Any size: from pc to Mpc § First order similar radio morphology (but differences depending on radio power, optical luminosity & orientation) § Typical radio power 1023 to 1028 W/Hz
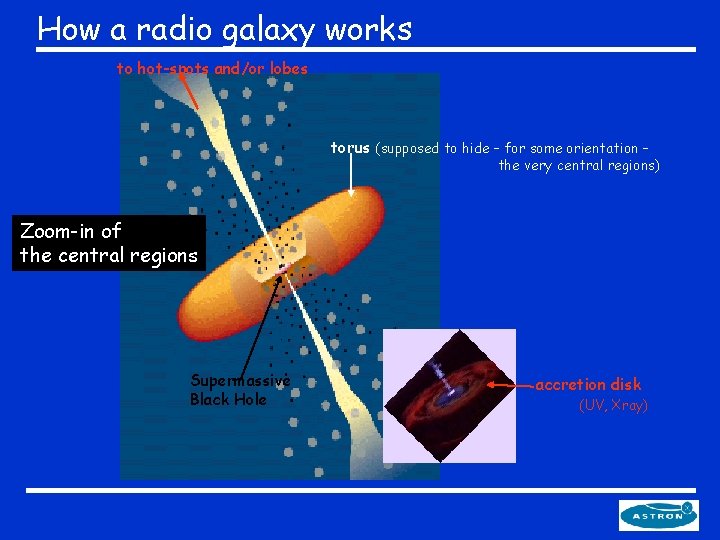
How a radio galaxy works to hot-spots and/or lobes torus (supposed to hide – for some orientation – the very central regions) Zoom-in of the central regions Supermassive Black Hole accretion disk (UV, Xray)
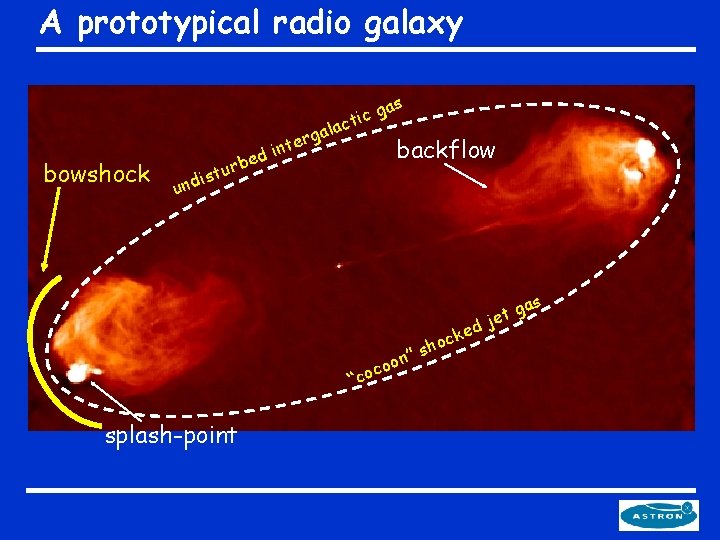
A prototypical radio galaxy bowshock is und d rbe tu in ala g r te s ga c i t c backflow as g et j d n” o o oc “c splash-point ke c o sh
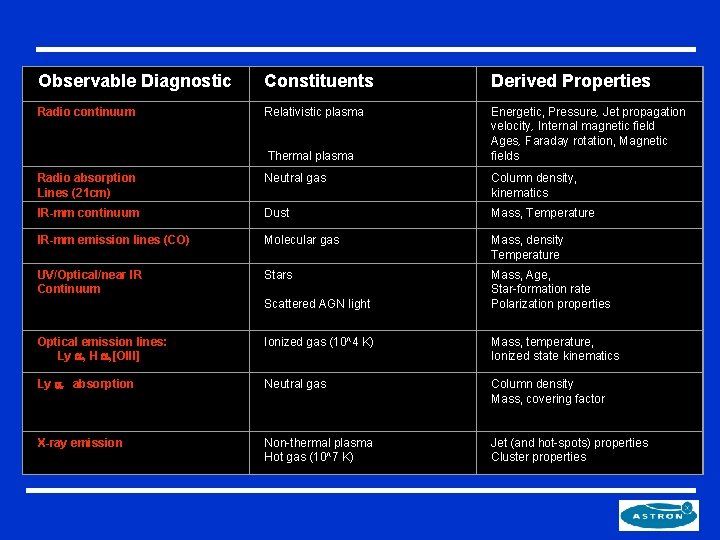
Observable Diagnostic Constituents Derived Properties Radio continuum Relativistic plasma Thermal plasma Energetic, Pressure, Jet propagation velocity, Internal magnetic field Ages, Faraday rotation, Magnetic fields Radio absorption Lines (21 cm) Neutral gas Column density, kinematics IR-mm continuum Dust Mass, Temperature IR-mm emission lines (CO) Molecular gas Mass, density Temperature UV/Optical/near IR Continuum Stars Scattered AGN light Mass, Age, Star-formation rate Polarization properties Optical emission lines: Ly , H , [OIII] Ionized gas (10^4 K) Mass, temperature, Ionized state kinematics Ly absorption Neutral gas Column density Mass, covering factor X-ray emission Non-thermal plasma Hot gas (10^7 K) Jet (and hot-spots) properties Cluster properties
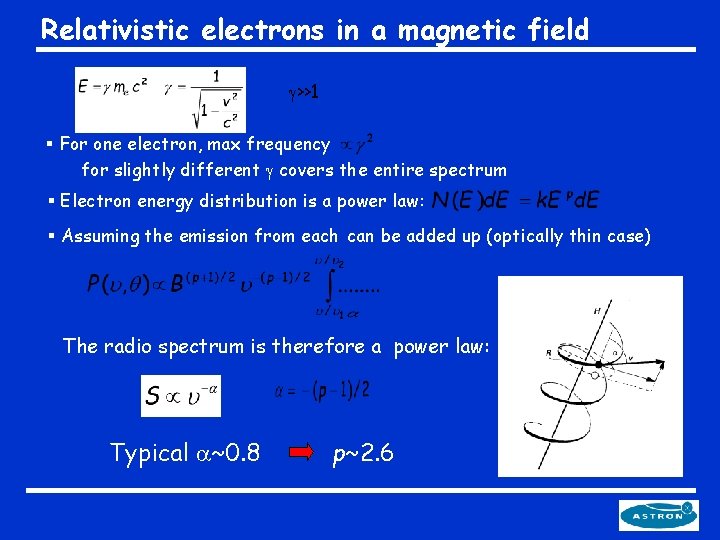
Relativistic electrons in a magnetic field >>1 § For one electron, max frequency for slightly different covers the entire spectrum § Electron energy distribution is a power law: § Assuming the emission from each can be added up (optically thin case) The radio spectrum is therefore a power law: Typical ~0. 8 p~2. 6
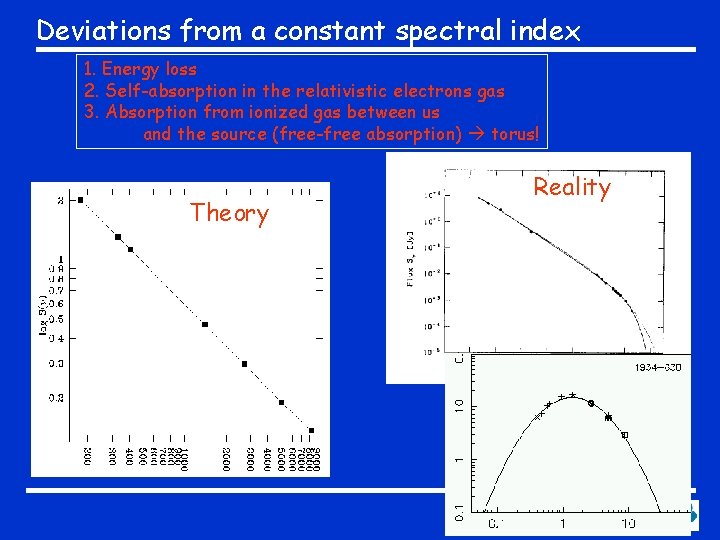
Deviations from a constant spectral index 1. Energy loss 2. Self-absorption in the relativistic electrons gas 3. Absorption from ionized gas between us and the source (free-free absorption) torus! Theory Reality
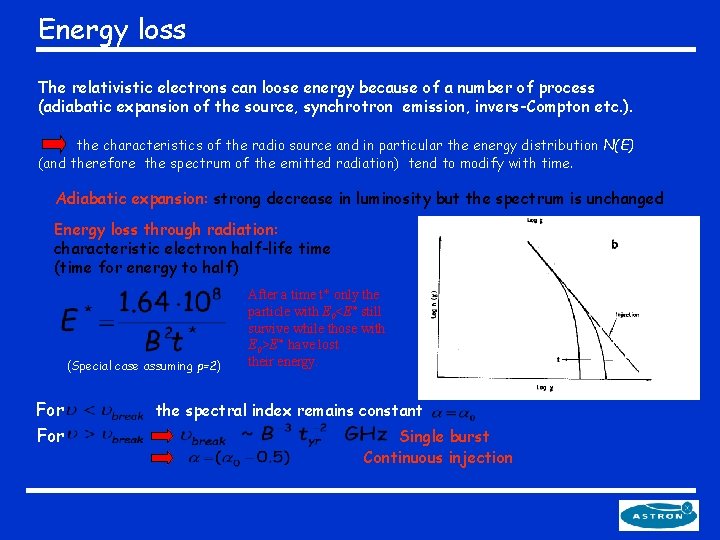
Energy loss The relativistic electrons can loose energy because of a number of process (adiabatic expansion of the source, synchrotron emission, invers-Compton etc. ). the characteristics of the radio source and in particular the energy distribution N(E) (and therefore the spectrum of the emitted radiation) tend to modify with time. Adiabatic expansion: strong decrease in luminosity but the spectrum is unchanged Energy loss through radiation: characteristic electron half-life time (time for energy to half) (Special case assuming p=2) For After a time t* only the particle with E 0<E* still survive while those with E 0>E* have lost their energy. the spectral index remains constant Single burst Continuous injection
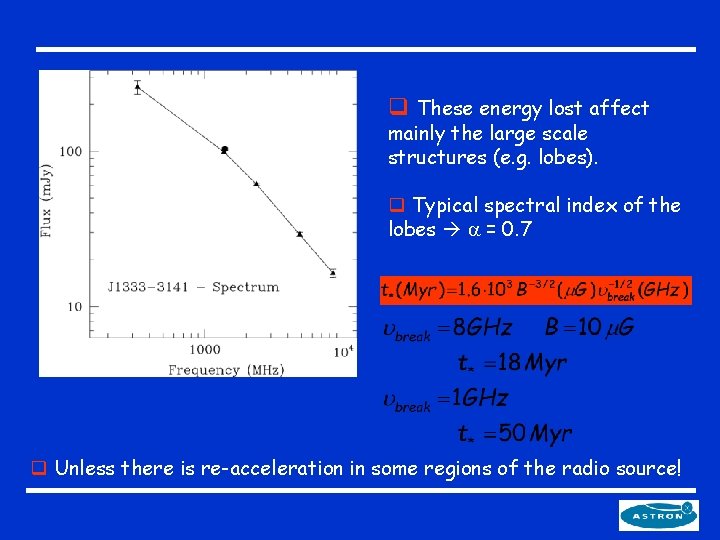
q These energy lost affect mainly the large scale structures (e. g. lobes). q Typical spectral index of the lobes = 0. 7 q Unless there is re-acceleration in some regions of the radio source!
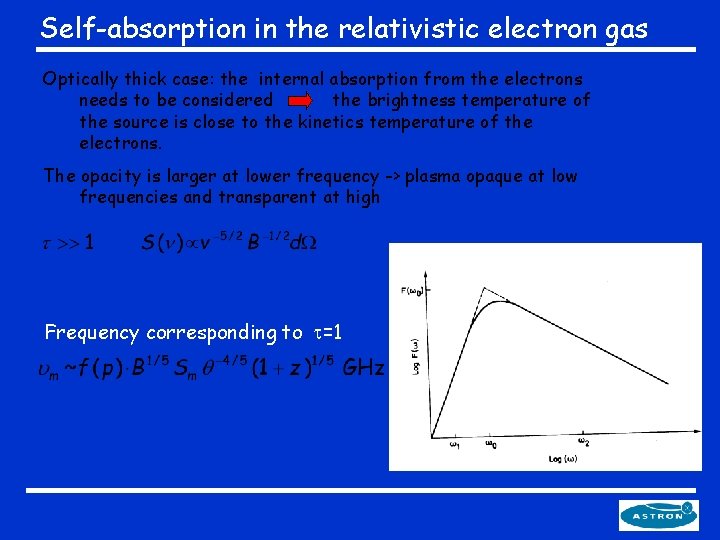
Self-absorption in the relativistic electron gas Optically thick case: the internal absorption from the electrons needs to be considered the brightness temperature of the source is close to the kinetics temperature of the electrons. The opacity is larger at lower frequency -> plasma opaque at low frequencies and transparent at high Frequency corresponding to =1
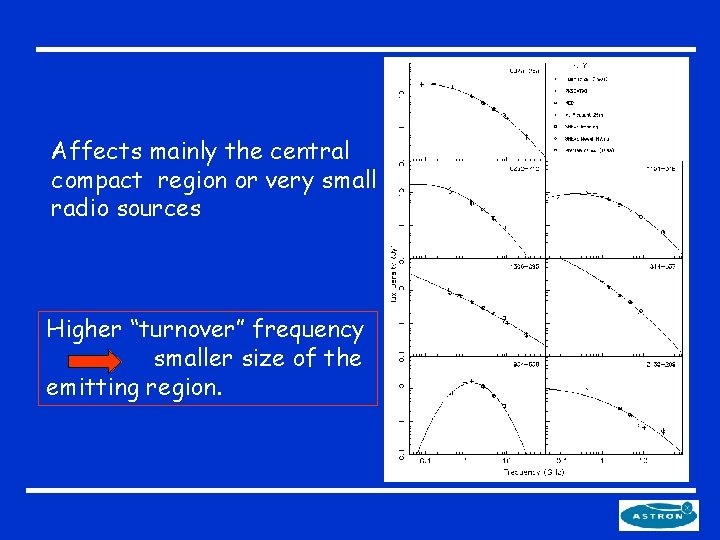
Affects mainly the central compact region or very small radio sources Higher “turnover” frequency smaller size of the emitting region.
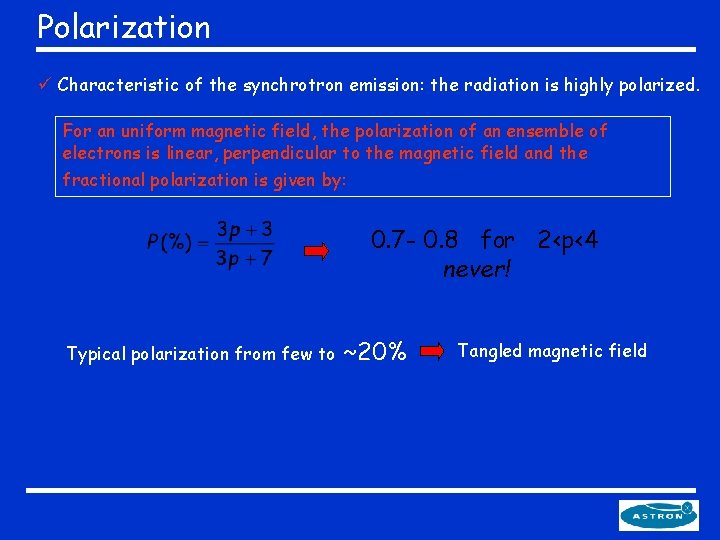
Polarization ü Characteristic of the synchrotron emission: the radiation is highly polarized. For an uniform magnetic field, the polarization of an ensemble of electrons is linear, perpendicular to the magnetic field and the fractional polarization is given by: 0. 7 - 0. 8 for 2<p<4 never! Typical polarization from few to ~20% Tangled magnetic field
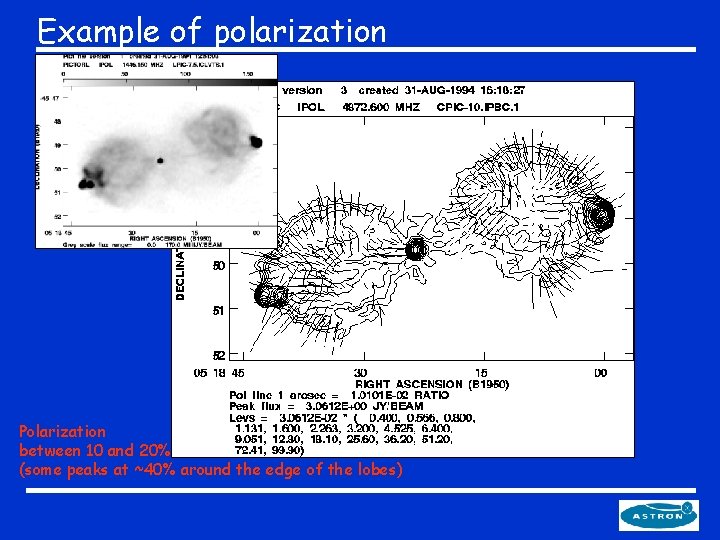
Example of polarization Polarization between 10 and 20% (some peaks at ~40% around the edge of the lobes)
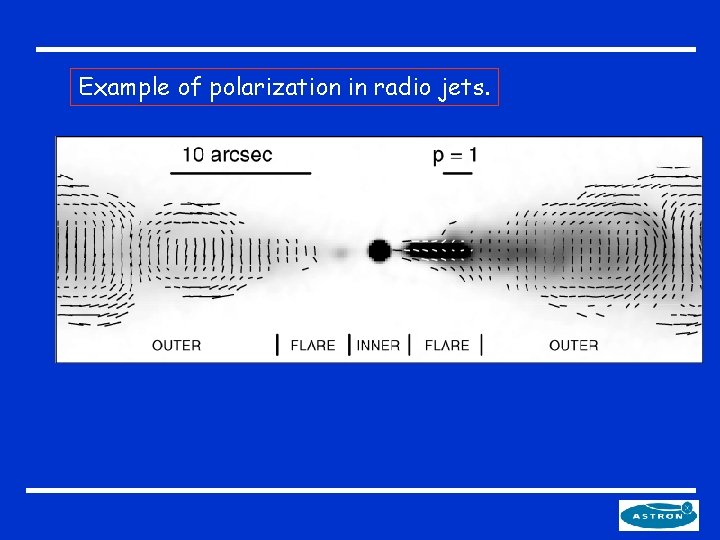
Example of polarization in radio jets.
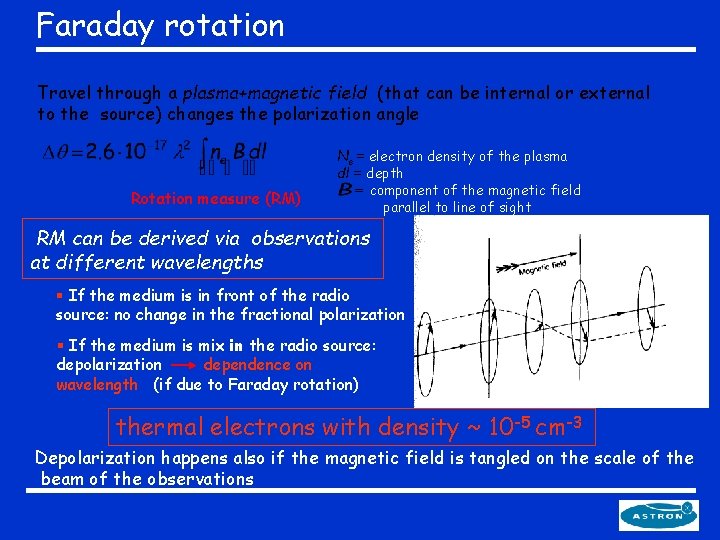
Faraday rotation Travel through a plasma+magnetic field (that can be internal or external to the source) changes the polarization angle Rotation measure (RM) Ne = electron density of the plasma dl = depth = component of the magnetic field parallel to line of sight RM can be derived via observations at different wavelengths § If the medium is in front of the radio source: no change in the fractional polarization § If the medium is mix in the radio source: depolarization dependence on wavelength (if due to Faraday rotation) thermal electrons with density ~ 10 -5 cm-3 Depolarization happens also if the magnetic field is tangled on the scale of the beam of the observations
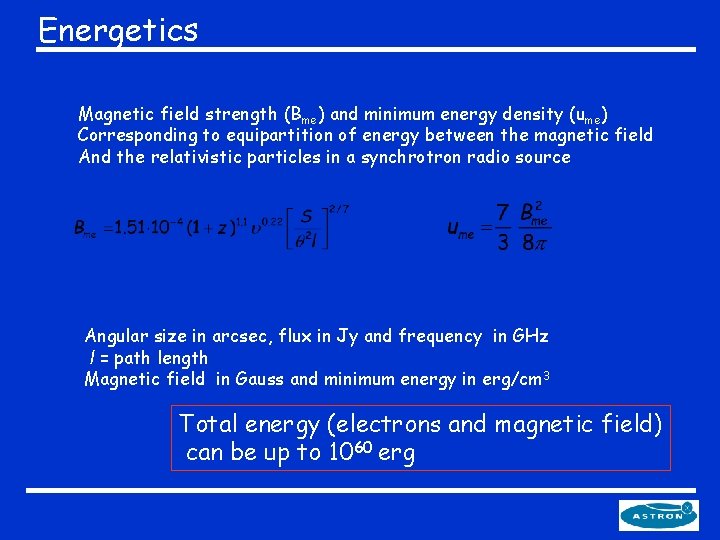
Energetics Magnetic field strength (Bme) and minimum energy density (ume) Corresponding to equipartition of energy between the magnetic field And the relativistic particles in a synchrotron radio source Angular size in arcsec, flux in Jy and frequency in GHz l = path length Magnetic field in Gauss and minimum energy in erg/cm 3 Total energy (electrons and magnetic field) can be up to 1060 erg
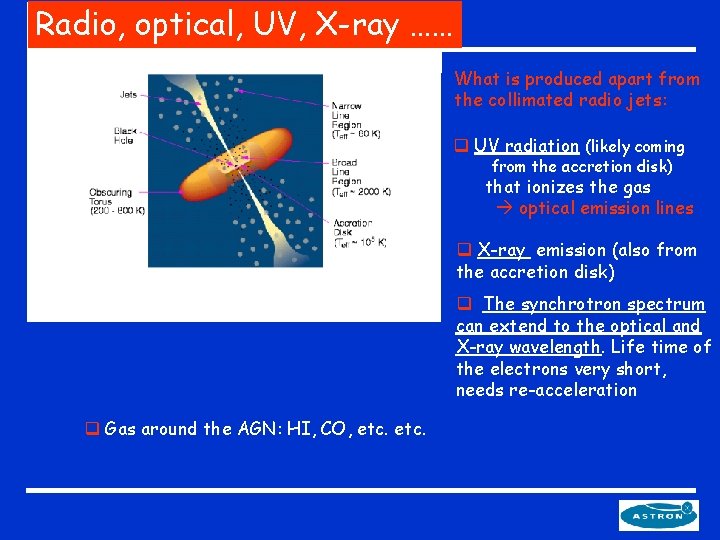
Radio, optical, UV, X-ray …… What is produced apart from the collimated radio jets: q UV radiation (likely coming from the accretion disk) that ionizes the gas optical emission lines q X-ray emission (also from the accretion disk) q The synchrotron spectrum can extend to the optical and X-ray wavelength. Life time of the electrons very short, needs re-acceleration q Gas around the AGN: HI, CO, etc.
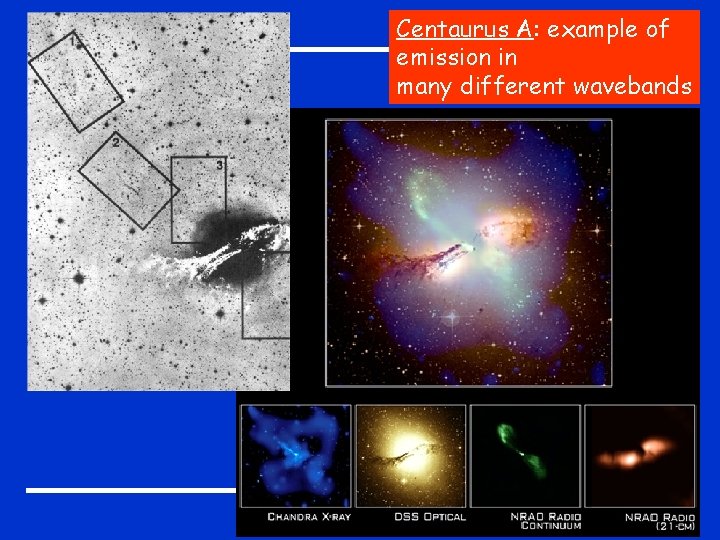
Centaurus A: example of emission in many different wavebands
Raffaella assetta
Enrico stefani
Raffaella nori
Raffaella geometrante
Raffaella niro
Raffaella niro
Raffaella pocobello
Fuciarelli raffaella pescara
Raffaella geometrante
Raffaella punzo
Life cycle of a galaxy
Properties of elliptical galaxies
Chapter 30 galaxies and the universe
How are active galaxies classified?
Elliptical galaxies facts
Evolution of galaxies
Types of galaxies
Classification
E irregulars
Billions of galaxies
Brainpop galaxies quiz answers
Electromagnetic star
Tipus de galaxies
Critical density
Era of galaxies
Galaxies lesson plan
4 types of galaxies
Conventional radio system
Cách giải mật thư tọa độ
Tư thế worm breton
ưu thế lai là gì
Thẻ vin
Thể thơ truyền thống
Cái miệng nó xinh thế
Các châu lục và đại dương trên thế giới