Protein Chemistry Basics Protein function Protein structure Primary
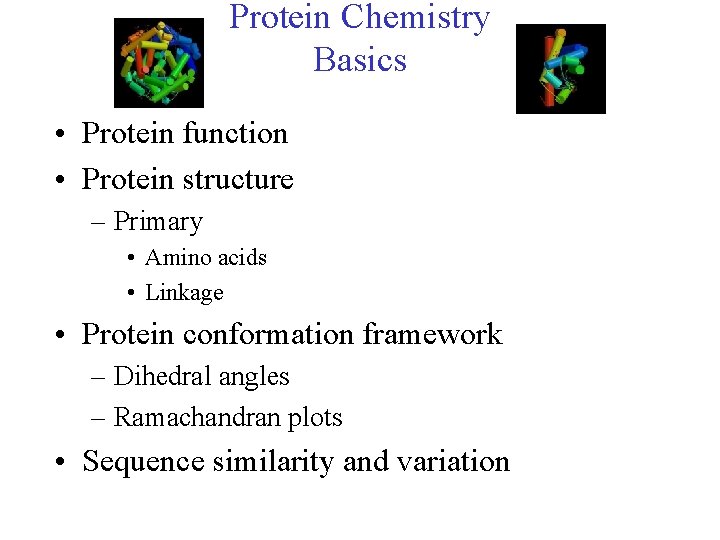
Protein Chemistry Basics • Protein function • Protein structure – Primary • Amino acids • Linkage • Protein conformation framework – Dihedral angles – Ramachandran plots • Sequence similarity and variation

Protein Function in Cell 1. Enzymes • Catalyze biological reactions 2. Structural role • • • Cell wall Cell membrane Cytoplasm
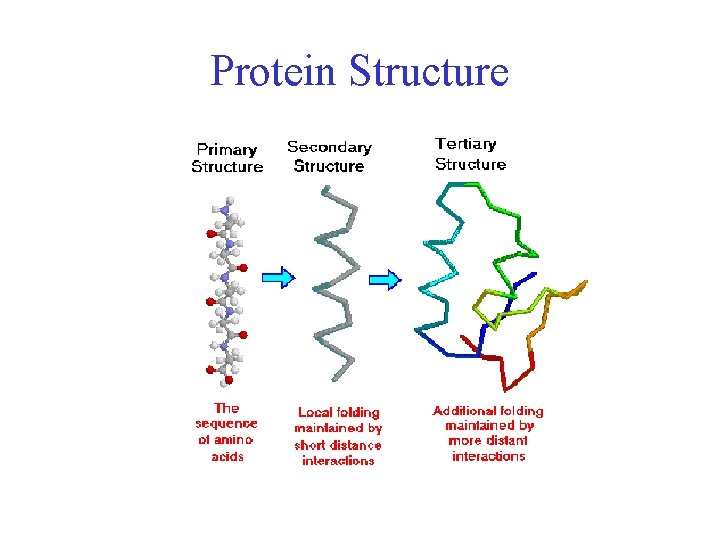
Protein Structure
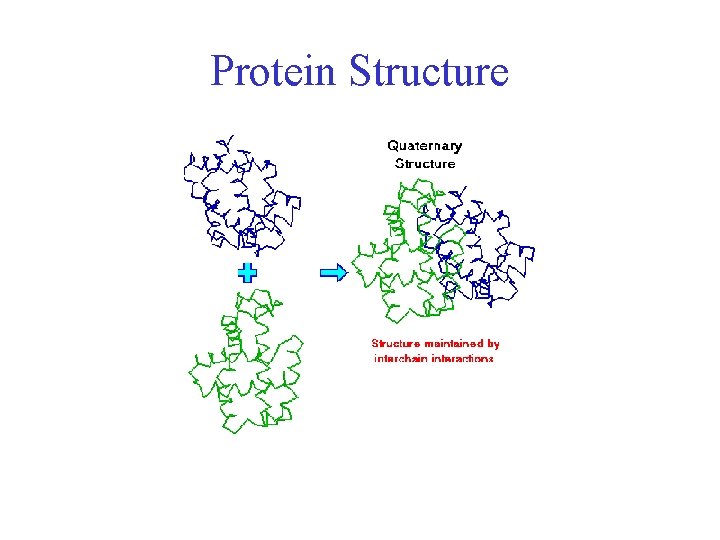
Protein Structure
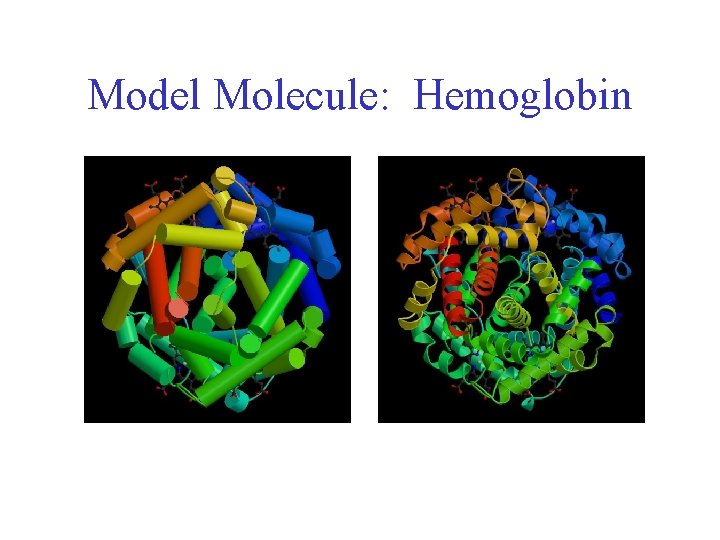
Model Molecule: Hemoglobin

Hemoglobin: Background • Protein in red blood cells
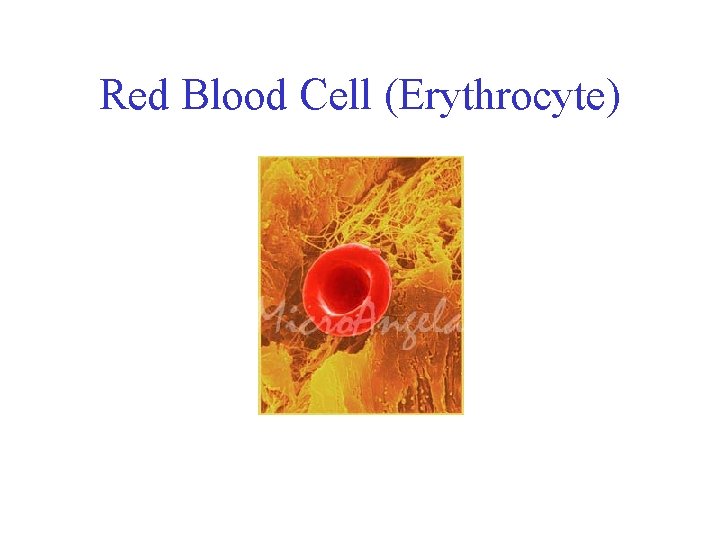
Red Blood Cell (Erythrocyte)

Hemoglobin: Background • Protein in red blood cells • Composed of four subunits, each containing a heme group: a ring-like structure with a central iron atom that binds oxygen

Heme Groups in Hemoglobin

Hemoglobin: Background • Protein in red blood cells • Composed of four subunits, each containing a heme group: a ring-like structure with a central iron atom that binds oxygen • Picks up oxygen in lungs, releases it in peripheral tissues (e. g. muscles)

Hemoglobin – Quaternary Structure Two alpha subunits and two beta subunits (141 AA per alpha, 146 AA per beta)
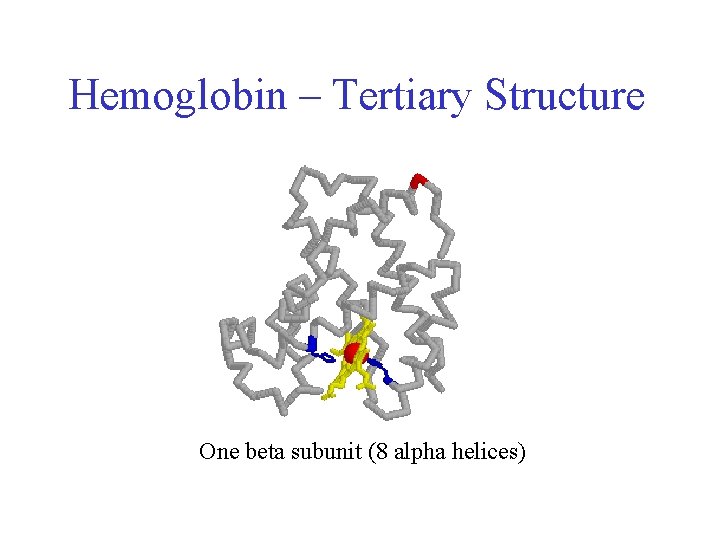
Hemoglobin – Tertiary Structure One beta subunit (8 alpha helices)
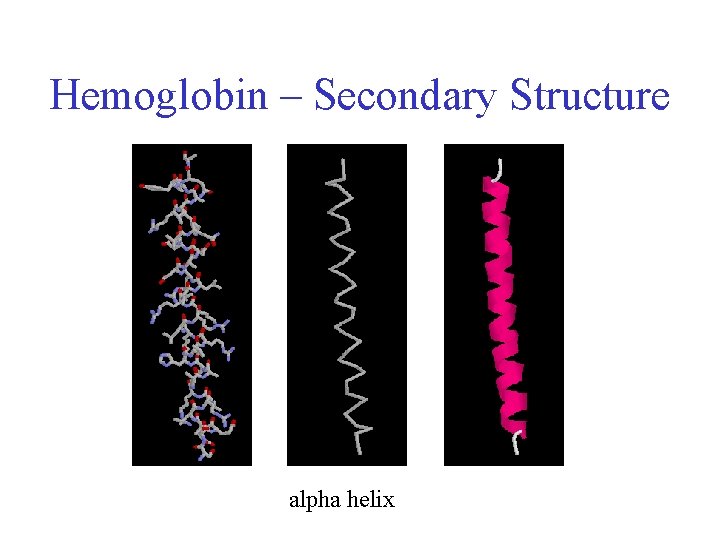
Hemoglobin – Secondary Structure alpha helix
![β-Hairpin Motif • Simplest protein motif involving two beta strands [from Wikipedia] – adjacent β-Hairpin Motif • Simplest protein motif involving two beta strands [from Wikipedia] – adjacent](http://slidetodoc.com/presentation_image/440744dd79e1258a2facfe962504209d/image-14.jpg)
β-Hairpin Motif • Simplest protein motif involving two beta strands [from Wikipedia] – adjacent in primary sequence – antiparallel – linked by a short loop • As isolated ribbon or part of beta sheet • a special case of a turn – direction of protein backbone reverses – flanking secondary structure elements interact (hydrogen bonds) Xin Zhan CS 882 course project 14
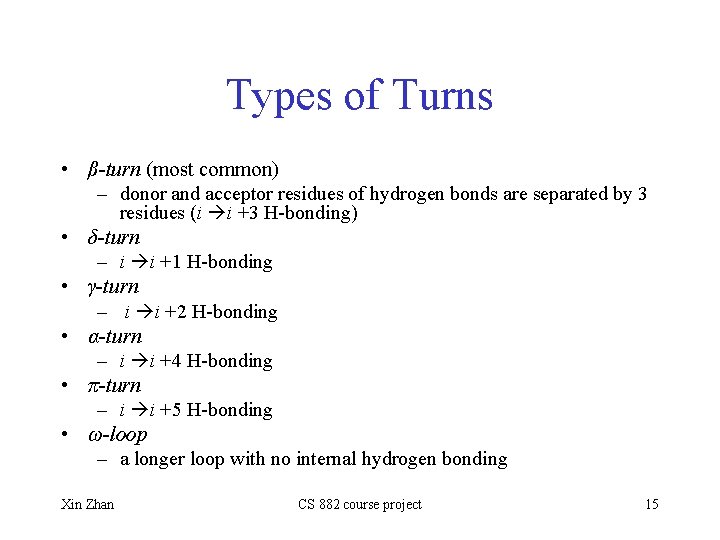
Types of Turns • β-turn (most common) – donor and acceptor residues of hydrogen bonds are separated by 3 residues (i i +3 H-bonding) • δ-turn – i i +1 H-bonding • γ-turn – i i +2 H-bonding • α-turn – i i +4 H-bonding • π-turn – i i +5 H-bonding • ω-loop – a longer loop with no internal hydrogen bonding Xin Zhan CS 882 course project 15

Structure Stabilizing Interactions • Noncovalent – Van der Waals forces (transient, weak electrical attraction of one atom for another) – Hydrophobic (clustering of nonpolar groups) – Hydrogen bonding

Hydrogen Bonding • Involves three atoms: – Donor electronegative atom (D) (Nitrogen or Oxygen in proteins) – Hydrogen bound to donor (H) – Acceptor electronegative atom (A) in close proximity D–H A
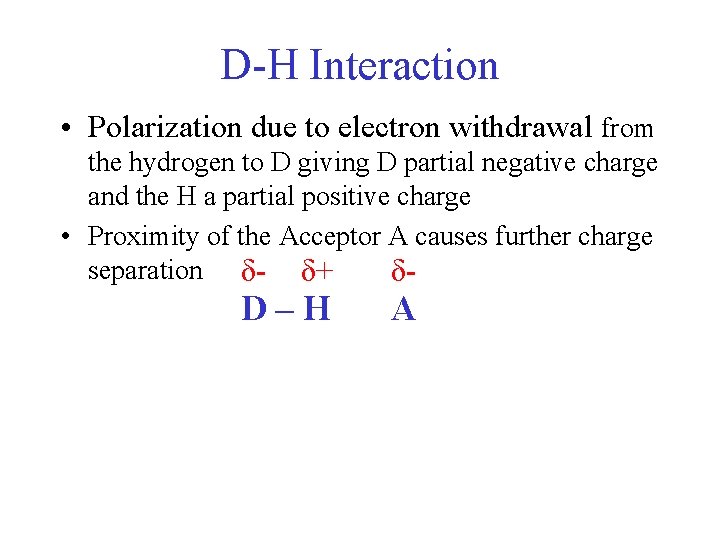
D-H Interaction • Polarization due to electron withdrawal from the hydrogen to D giving D partial negative charge and the H a partial positive charge • Proximity of the Acceptor A causes further charge separation δ- δ+ δ- D–H A

D-H Interaction • Polarization due to electron withdrawal from the hydrogen to D giving D partial negative charge and the H a partial positive charge • Proximity of the Acceptor A causes further charge separation δ- • Result: δ+ δ- D–H A – Closer approach of A to H – Higher interaction energy than a simple van der Waals interaction

Hydrogen Bonding And Secondary Structure alpha-helix beta-sheet

Structure Stabilizing Interactions • Noncovalent – Van der Waals forces (transient, weak electrical attraction of one atom for another) – Hydrophobic (clustering of nonpolar groups) – Hydrogen bonding • Covalent – Disulfide bonds

Disulfide Bonds • Side chain of cysteine contains highly reactive thiol group • Two thiol groups form a disulfide bond

Disulfide Bridge

Disulfide Bonds • Side chain of cysteine contains highly reactive thiol group • Two thiol groups form a disulfide bond • Contribute to the stability of the folded state by linking distant parts of the polypeptide chain
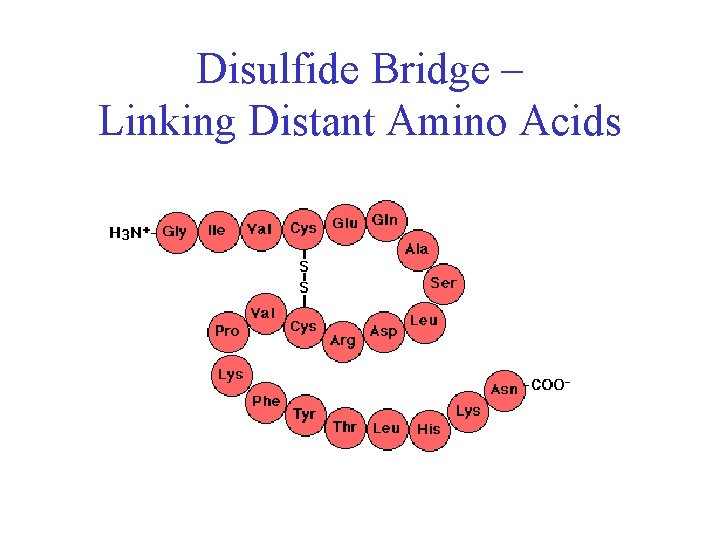
Disulfide Bridge – Linking Distant Amino Acids
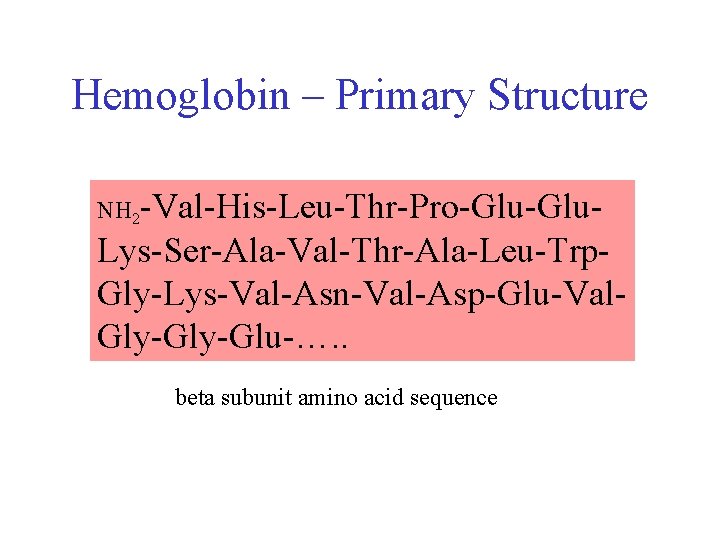
Hemoglobin – Primary Structure NH 2 -Val-His-Leu-Thr-Pro-Glu- Lys-Ser-Ala-Val-Thr-Ala-Leu-Trp. Gly-Lys-Val-Asn-Val-Asp-Glu-Val. Gly-Glu-…. . beta subunit amino acid sequence

Protein Structure - Primary • Protein: chain of amino acids joined by peptide bonds

Protein Structure - Primary • Protein: chain of amino acids joined by peptide bonds • Amino Acid – Central carbon (Cα) attached to: • • Hydrogen (H) Amino group (-NH 2) Carboxyl group (-COOH) Side chain (R)

General Amino Acid Structure H H 2 N α C R COOH
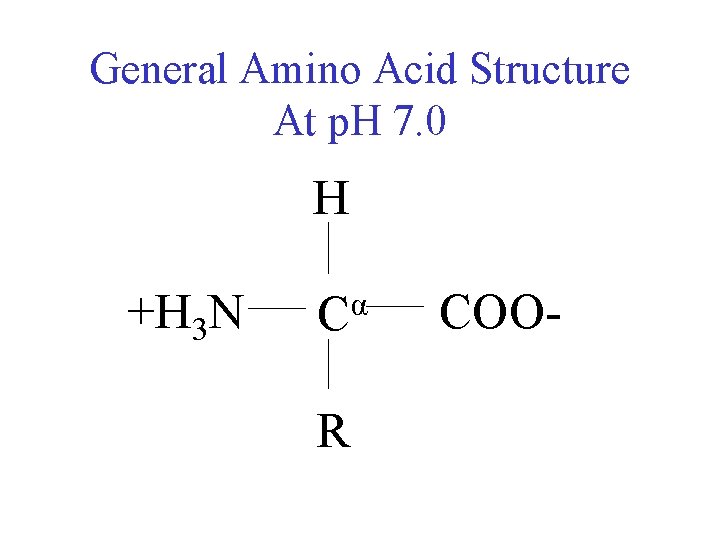
General Amino Acid Structure At p. H 7. 0 H +H 3 N α C R COO-

General Amino Acid Structure
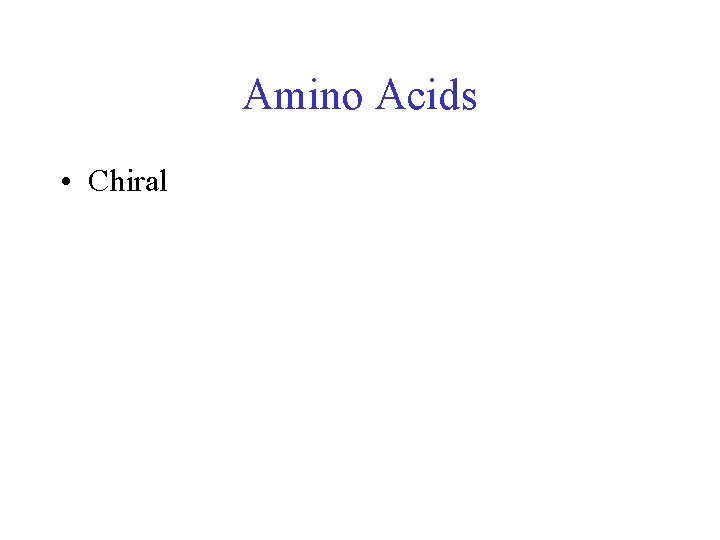
Amino Acids • Chiral

Chirality: Glyceraldehyde D-glyderaldehyde L-glyderaldehyde
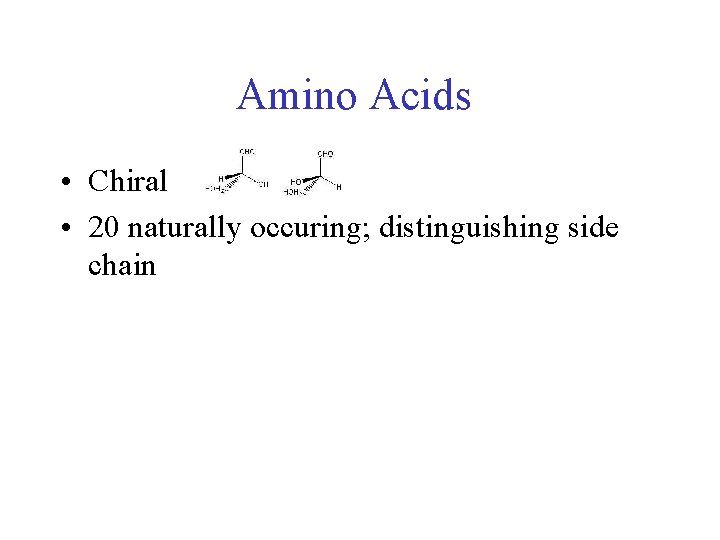
Amino Acids • Chiral • 20 naturally occuring; distinguishing side chain
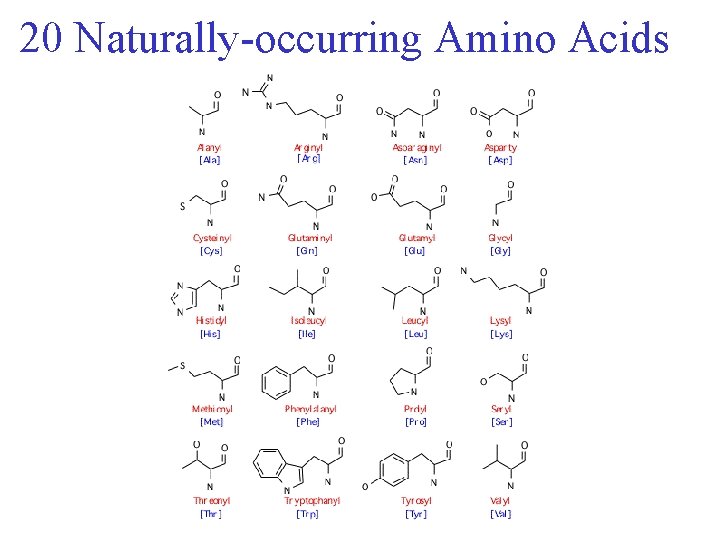
20 Naturally-occurring Amino Acids

Amino Acids • Chiral • 20 naturally occuring; distinguishing side chain • Classification: • Non-polar (hydrophobic) • Charged polar • Uncharged polar
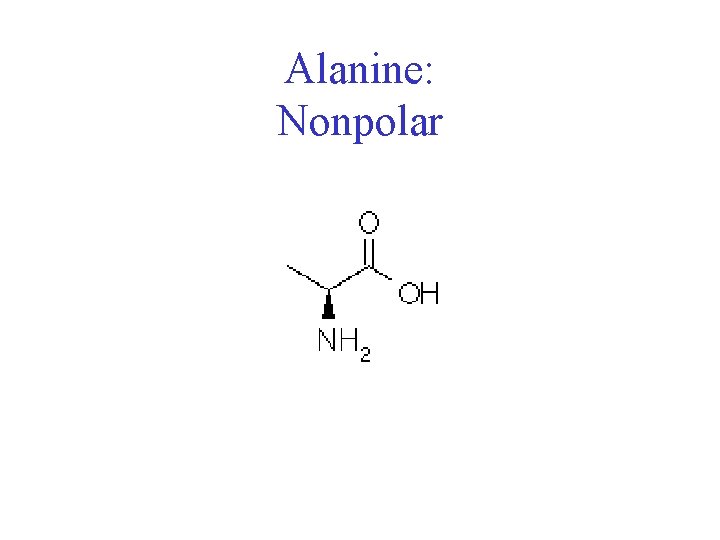
Alanine: Nonpolar
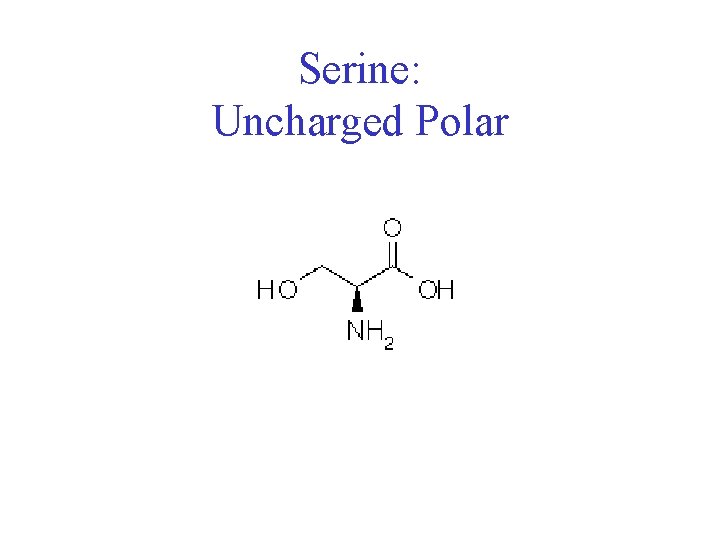
Serine: Uncharged Polar

Aspartic Acid Charged Polar

Glycine Nonpolar (special case)
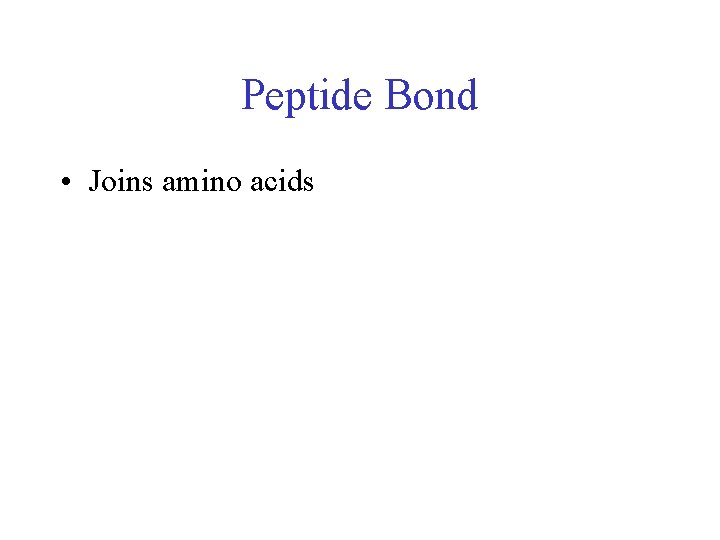
Peptide Bond • Joins amino acids

Peptide Bond Formation
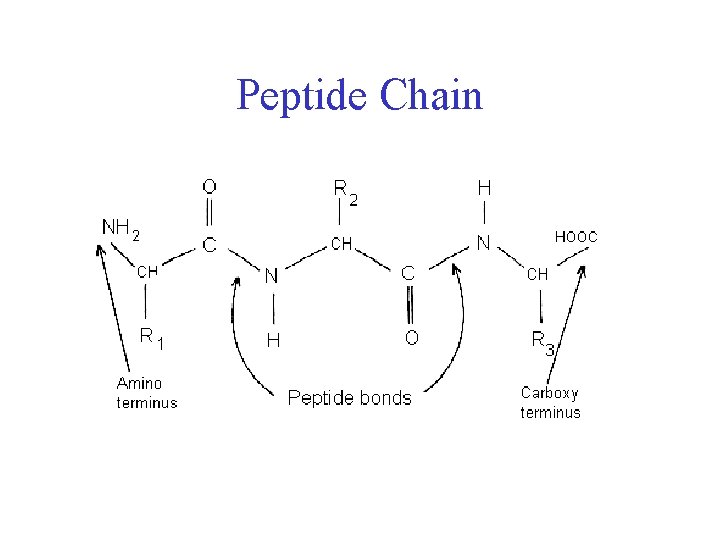
Peptide Chain

Peptide Bond • Joins amino acids • 40% double bond character – Caused by resonance

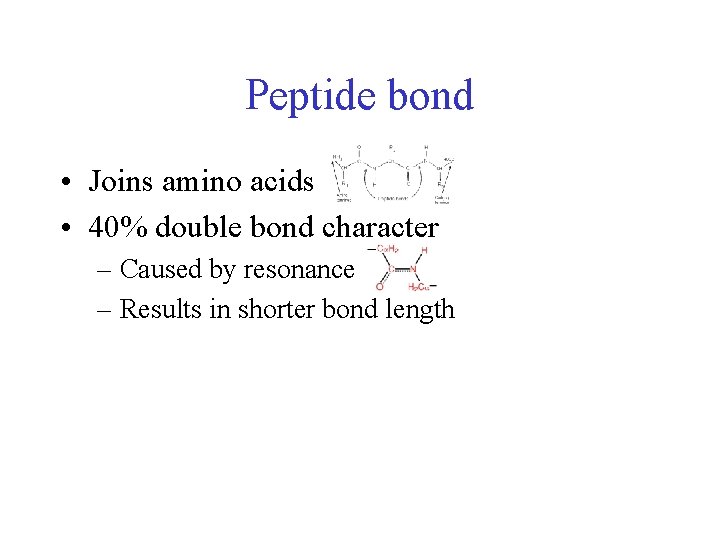
Peptide bond • Joins amino acids • 40% double bond character – Caused by resonance – Results in shorter bond length

Peptide Bond Lengths

Peptide bond • Joins amino acids • 40% double bond character – Caused by resonance – Results in shorter bond length – Double bond disallows rotation

Protein Conformation Framework • Bond rotation determines protein folding, 3 D structure
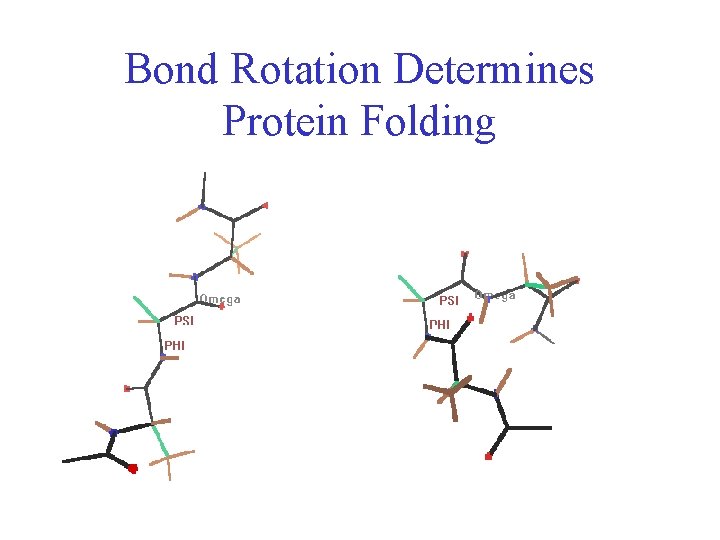
Bond Rotation Determines Protein Folding
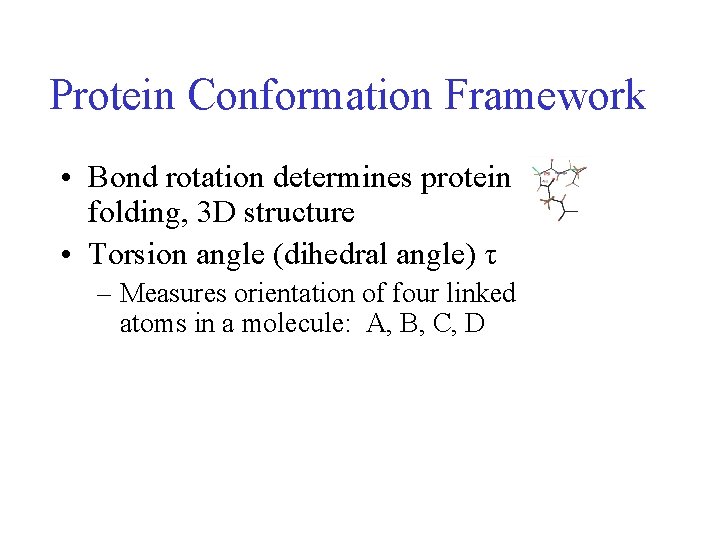
Protein Conformation Framework • Bond rotation determines protein folding, 3 D structure • Torsion angle (dihedral angle) τ – Measures orientation of four linked atoms in a molecule: A, B, C, D

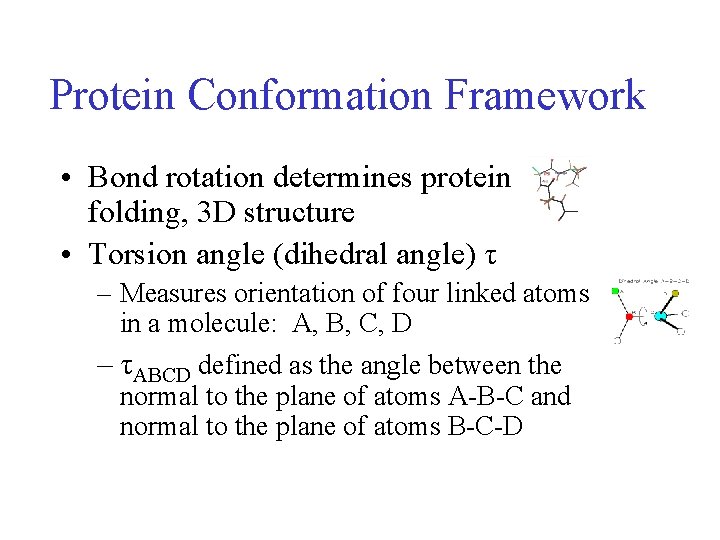
Protein Conformation Framework • Bond rotation determines protein folding, 3 D structure • Torsion angle (dihedral angle) τ – Measures orientation of four linked atoms in a molecule: A, B, C, D – τABCD defined as the angle between the normal to the plane of atoms A-B-C and normal to the plane of atoms B-C-D
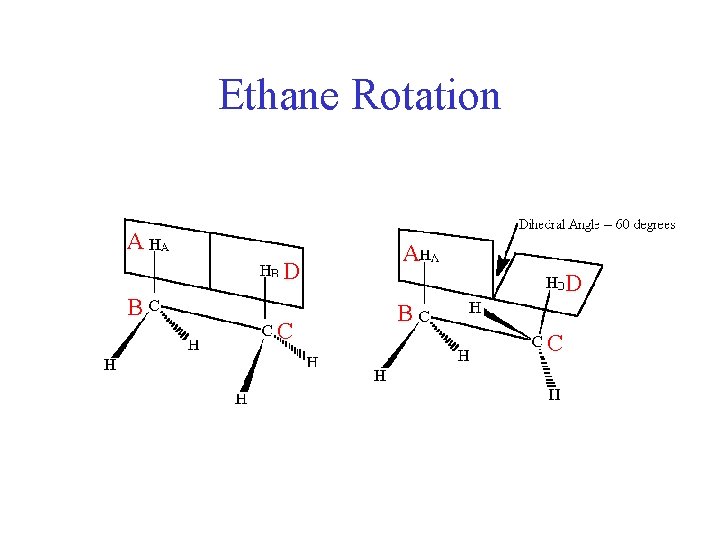
Ethane Rotation A D B C

Protein Conformation Framework • Bond rotation determines protein folding, 3 D structure • Torsion angle (dihedral angle) τ – Measures orientation of four linked atoms in a molecule: A, B, C, D – τABCD defined as the angle between the normal to the plane of atoms A-B-C and normal to the plane of atoms B-C-D – Three repeating torsion angles along protein backbone: ω, φ, ψ

Backbone Torsion Angles

Backbone Torsion Angles • Dihedral angle ω : rotation about the peptide bond, namely Cα 1 -{C-N}- Cα 2
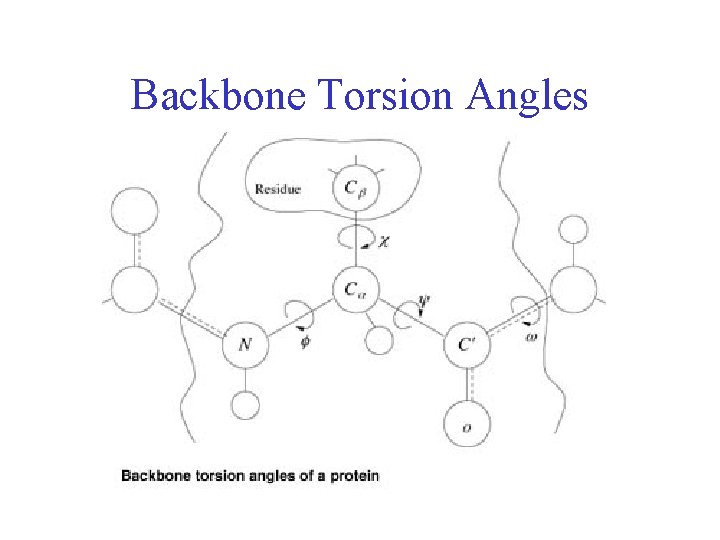
Backbone Torsion Angles

Backbone Torsion Angles • Dihedral angle ω : rotation about the peptide bond, namely Cα 1 -{C-N}- Cα 2 • Dihedral angle φ : rotation about the bond between N and Cα
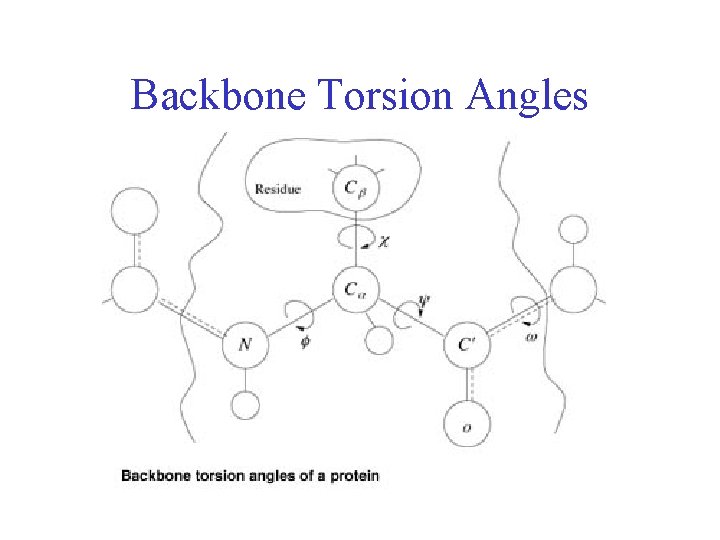
Backbone Torsion Angles
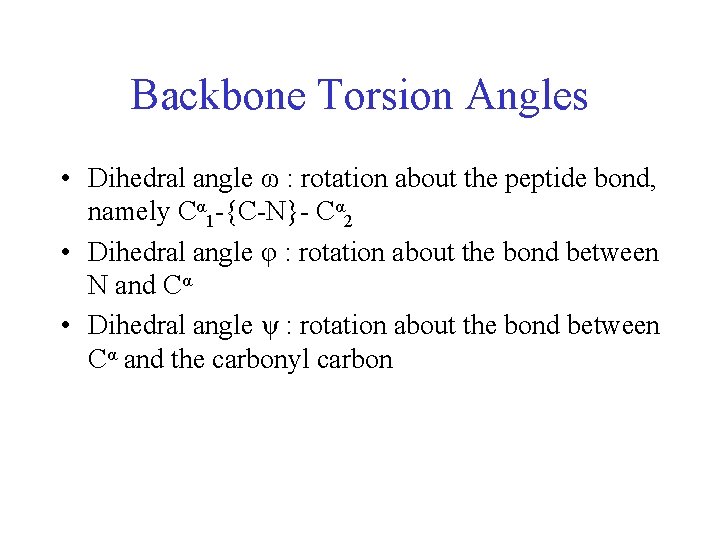
Backbone Torsion Angles • Dihedral angle ω : rotation about the peptide bond, namely Cα 1 -{C-N}- Cα 2 • Dihedral angle φ : rotation about the bond between N and Cα • Dihedral angle ψ : rotation about the bond between Cα and the carbonyl carbon

Backbone Torsion Angles

Backbone Torsion Angles • ω angle tends to be planar (0º - cis, or 180 º - trans) due to delocalization of carbonyl π electrons and nitrogen lone pair
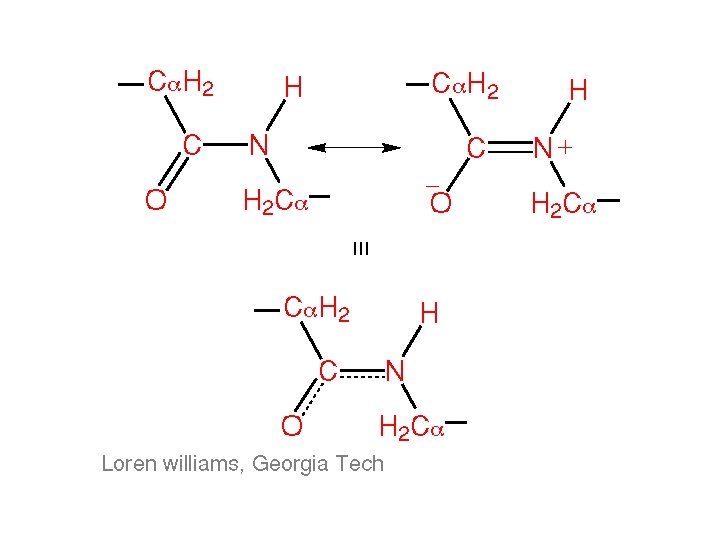
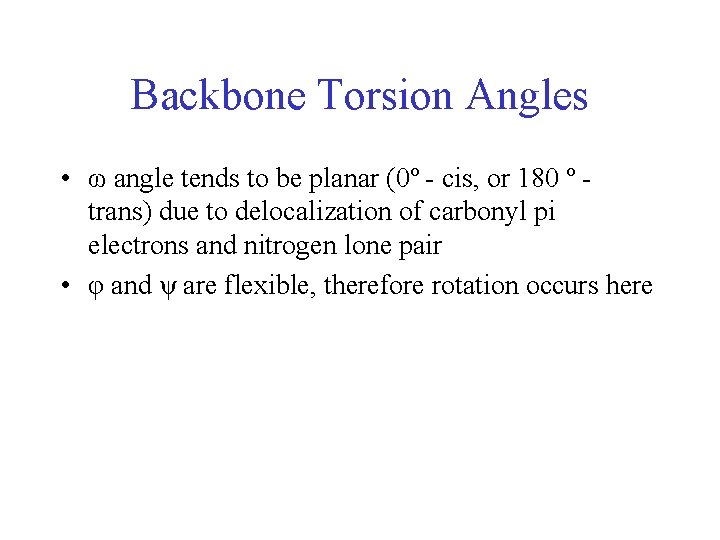
Backbone Torsion Angles • ω angle tends to be planar (0º - cis, or 180 º - trans) due to delocalization of carbonyl pi electrons and nitrogen lone pair • φ and ψ are flexible, therefore rotation occurs here

Backbone Torsion Angles
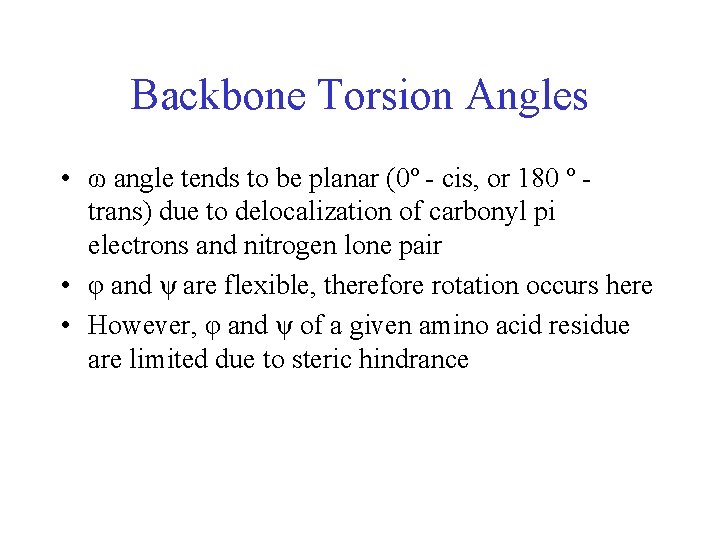
Backbone Torsion Angles • ω angle tends to be planar (0º - cis, or 180 º - trans) due to delocalization of carbonyl pi electrons and nitrogen lone pair • φ and ψ are flexible, therefore rotation occurs here • However, φ and ψ of a given amino acid residue are limited due to steric hindrance
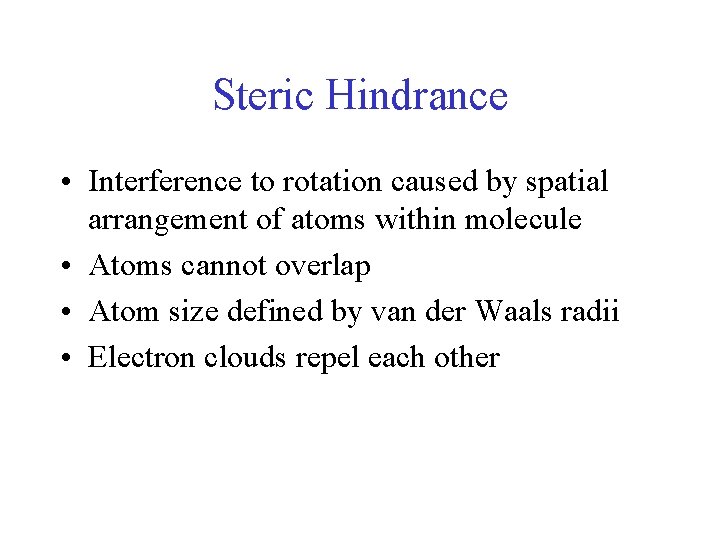
Steric Hindrance • Interference to rotation caused by spatial arrangement of atoms within molecule • Atoms cannot overlap • Atom size defined by van der Waals radii • Electron clouds repel each other
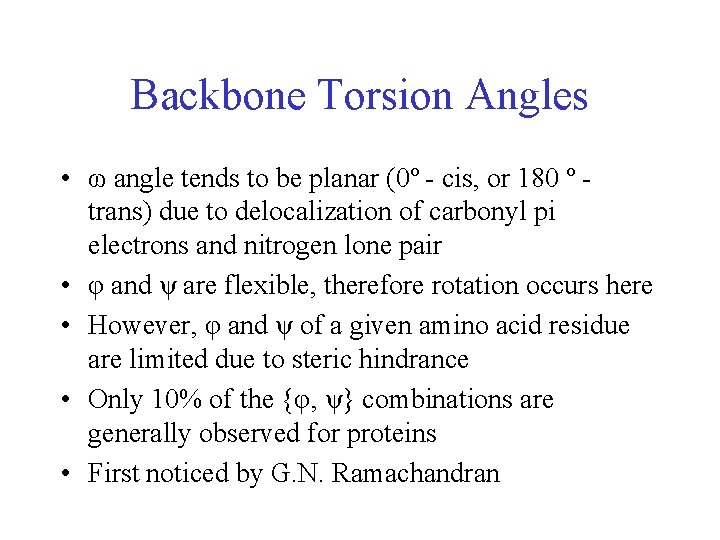
Backbone Torsion Angles • ω angle tends to be planar (0º - cis, or 180 º - trans) due to delocalization of carbonyl pi electrons and nitrogen lone pair • φ and ψ are flexible, therefore rotation occurs here • However, φ and ψ of a given amino acid residue are limited due to steric hindrance • Only 10% of the {φ, ψ} combinations are generally observed for proteins • First noticed by G. N. Ramachandran

G. N. Ramachandran • Used computer models of small polypeptides to systematically vary φ and ψ with the objective of finding stable conformations • For each conformation, the structure was examined for close contacts between atoms • Atoms were treated as hard spheres with dimensions corresponding to their van der Waals radii • Therefore, φ and ψ angles which cause spheres to collide correspond to sterically disallowed conformations of the polypeptide backbone
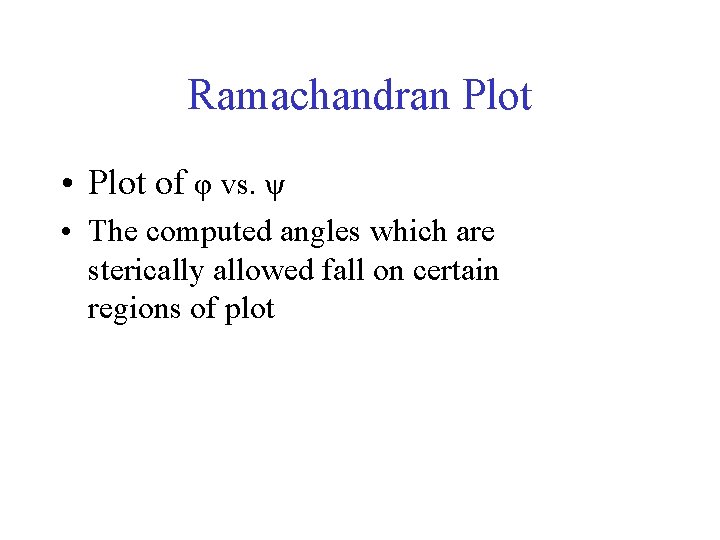
Ramachandran Plot • Plot of φ vs. ψ • The computed angles which are sterically allowed fall on certain regions of plot

Computed Ramachandran Plot White = sterically disallowed conformations (atoms come closer than sum of van der Waals radii) Blue = sterically allowed conformations

Ramachandran Plot • Plot of φ vs. ψ • Computed sterically allowed angles fall on certain regions of plot • Experimentally determined angles fall on same regions

Experimental Ramachandran Plot φ, ψ distribution in 42 high-resolution protein structures (x-ray crystallography)

Ramachandran Plot And Secondary Structure • Repeating values of φ and ψ along the chain result in regular structure • For example, repeating values of φ ~ -57° and ψ ~ -47° give a right-handed helical fold (the alphahelix)

The structure of cytochrome C shows many segments of helix and the Ramachandran plot shows a tight grouping of φ, ψ angles near -50, -50 alpha-helix cytochrome C Ramachandran plot

Similarly, repetitive values in the region of φ = -110 to – 140 and ψ = +110 to +135 give beta sheets. The structure of plastocyanin is composed mostly of beta sheets; the Ramachandran plot shows values in the – 110, +130 region: beta-sheet plastocyanin Ramachandran plot


Ramachandran Plot And Secondary Structure • White = sterically disallowed conformations • Red = sterically allowed regions if strict (greater) radii are used (namely righthanded alpha helix and beta sheet) • Yellow = sterically allowed if shorter radii are used (i. e. atoms allowed closer together; brings out left-handed helix)
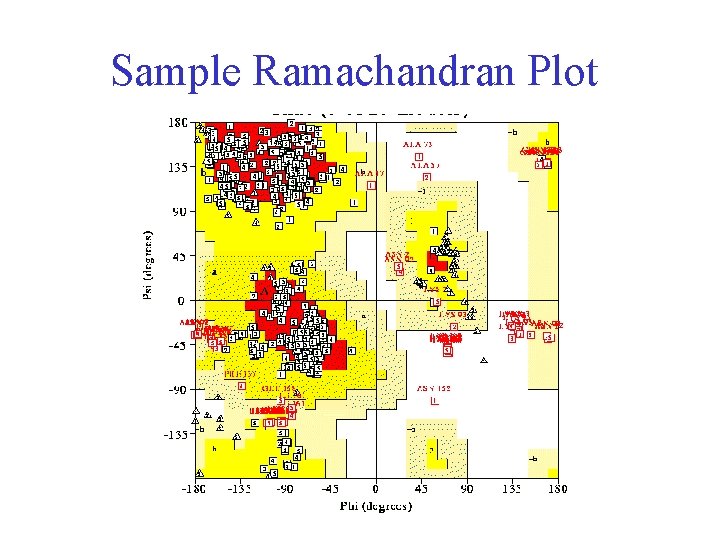
Sample Ramachandran Plot
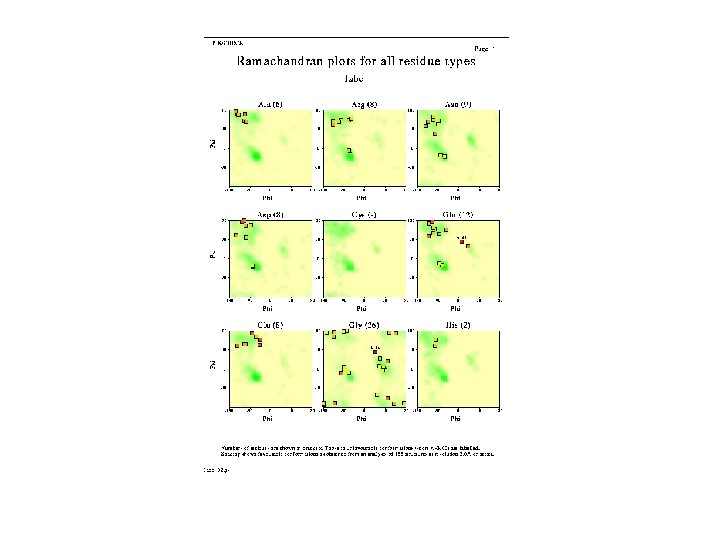
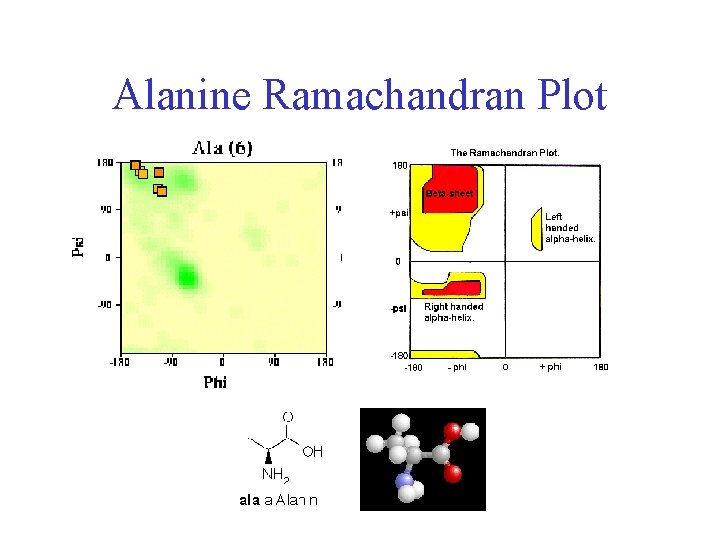
Alanine Ramachandran Plot

Arginine Ramachandran Plot
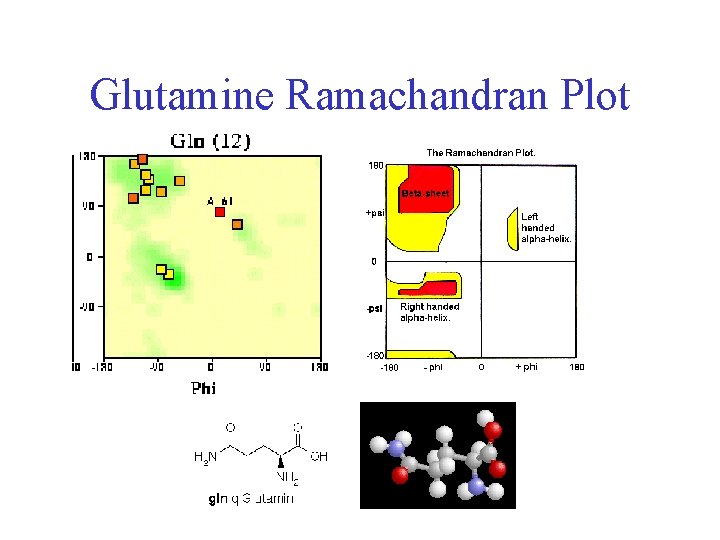
Glutamine Ramachandran Plot
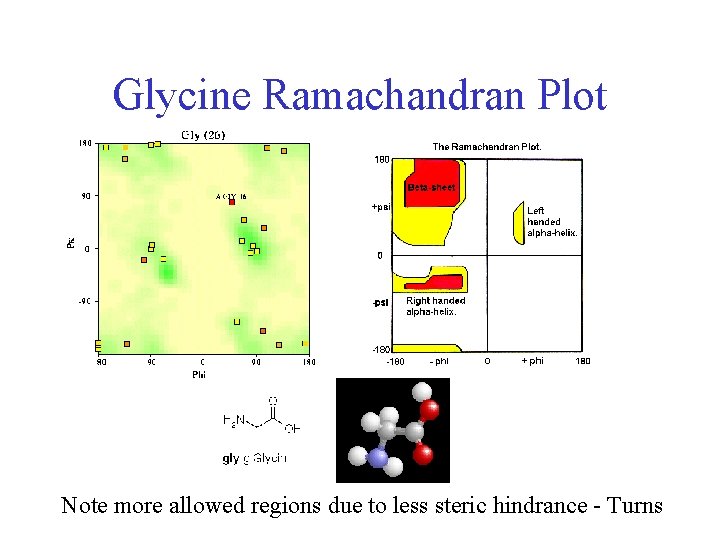
Glycine Ramachandran Plot Note more allowed regions due to less steric hindrance - Turns

Proline Ramachandran Plot Note less allowed regions due to structure rigidity
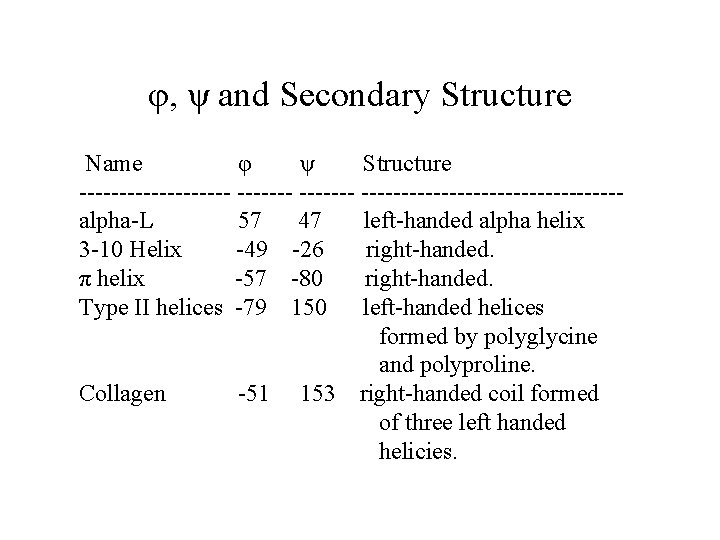
φ, ψ and Secondary Structure Name φ ψ Structure ---------- ----------------alpha-L 57 47 left-handed alpha helix 3 -10 Helix -49 -26 right-handed. π helix -57 -80 right-handed. Type II helices -79 150 left-handed helices formed by polyglycine and polyproline. Collagen -51 153 right-handed coil formed of three left handed helicies.
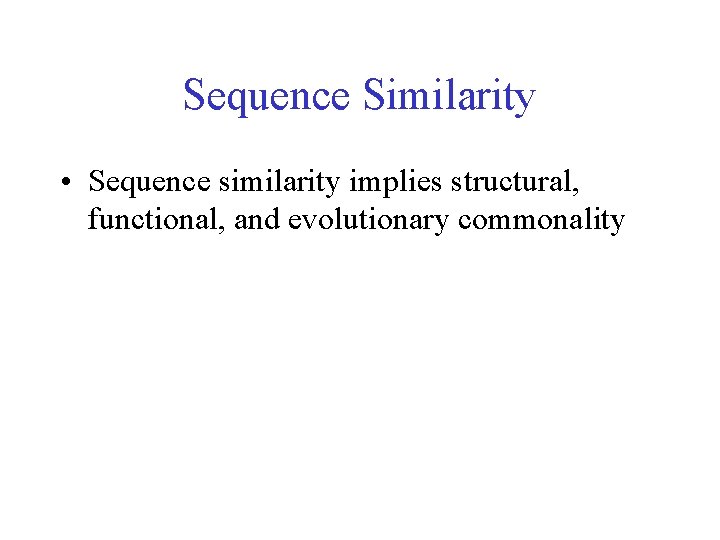
Sequence Similarity • Sequence similarity implies structural, functional, and evolutionary commonality

Homologous Proteins: Enterotoxin and Cholera toxin Enterotoxin Cholera toxin 80% homology
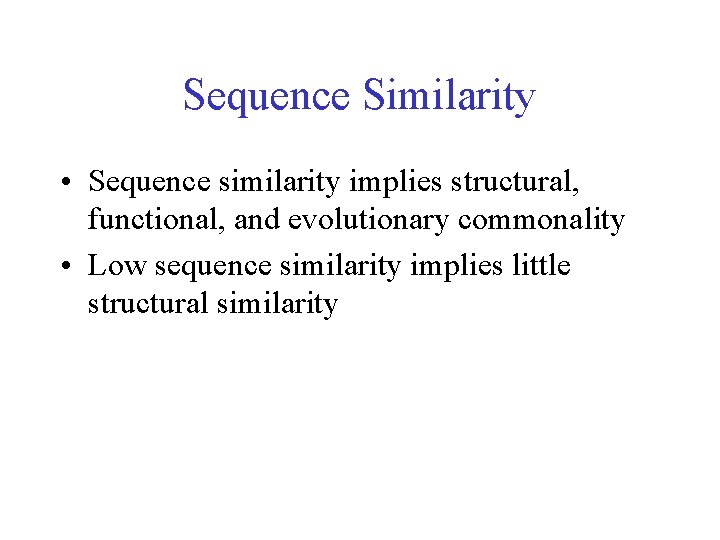
Sequence Similarity • Sequence similarity implies structural, functional, and evolutionary commonality • Low sequence similarity implies little structural similarity

Nonhomologous Proteins: Cytochrome and Barstar Cytochrome Barstar Less than 20% homology
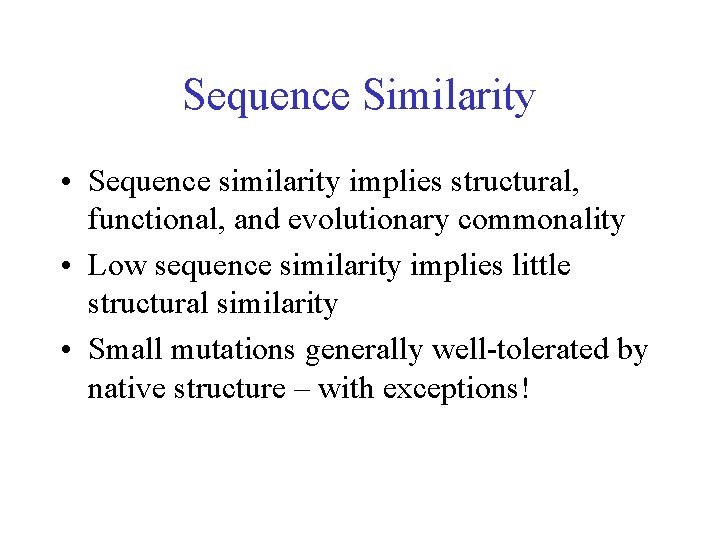
Sequence Similarity • Sequence similarity implies structural, functional, and evolutionary commonality • Low sequence similarity implies little structural similarity • Small mutations generally well-tolerated by native structure – with exceptions!
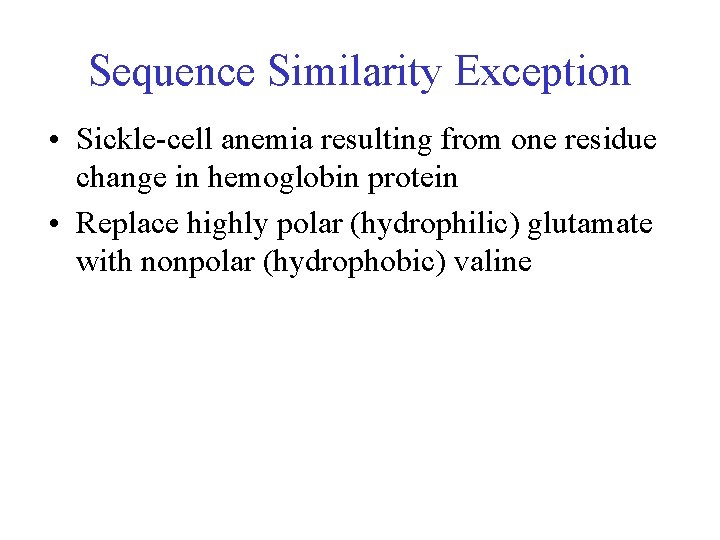
Sequence Similarity Exception • Sickle-cell anemia resulting from one residue change in hemoglobin protein • Replace highly polar (hydrophilic) glutamate with nonpolar (hydrophobic) valine

Sickle-cell mutation in hemoglobin sequence

Normal Trait • Hemoglobin molecules exist as single, isolated units in RBC, whether oxygen bound or not • Cells maintain basic disc shape, whether transporting oxygen or not
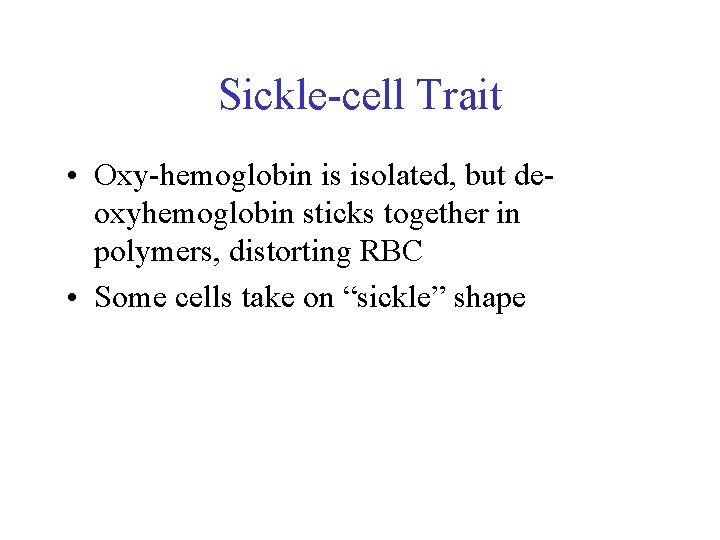
Sickle-cell Trait • Oxy-hemoglobin is isolated, but deoxyhemoglobin sticks together in polymers, distorting RBC • Some cells take on “sickle” shape
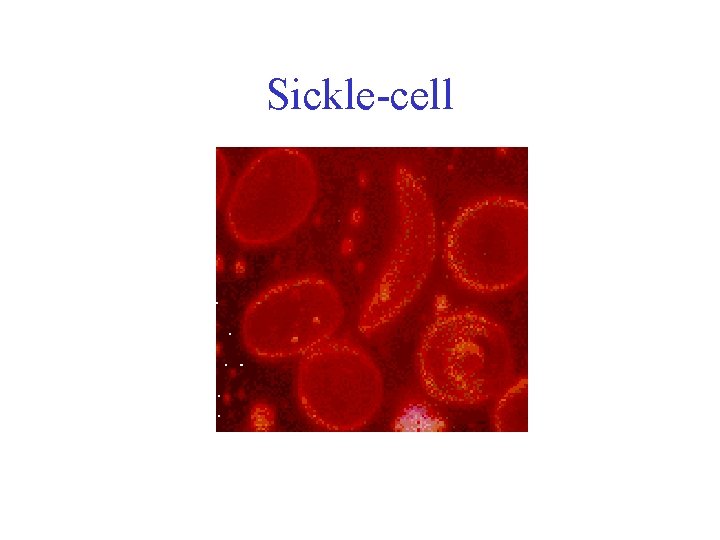
Sickle-cell

RBC Distortion • Hydrophobic valine replaces hydrophilic glutamate • Causes hemoglobin molecules to repel water and be attracted to one another • Leads to the formation of long hemoglobin filaments

Hemoglobin Polymerization Normal Mutant

RBC Distortion • Hydrophobic valine replaces hydrophilic glutamate • Causes hemoglobin molecules to repel water and be attracted to one another • Leads to the formation of long hemoglobin filaments • Filaments distort the shape of red blood cells (analogy: icicle in a water balloon) • Rigid structure of sickle cells blocks capillaries and prevents red blood cells from delivering oxygen
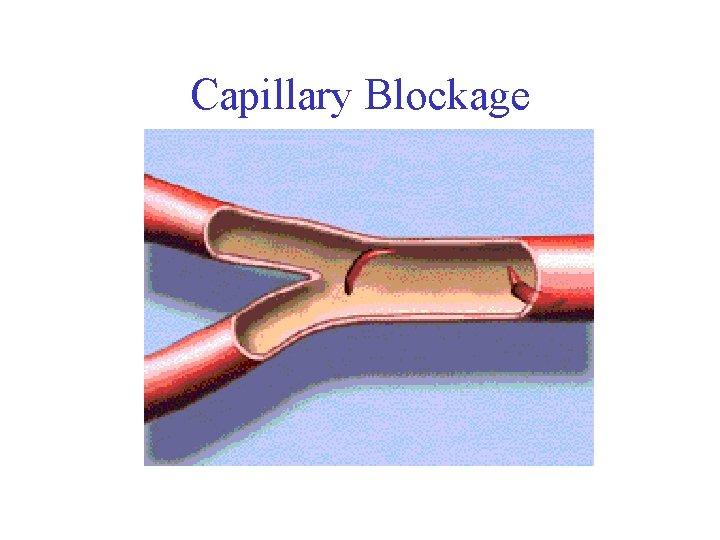
Capillary Blockage
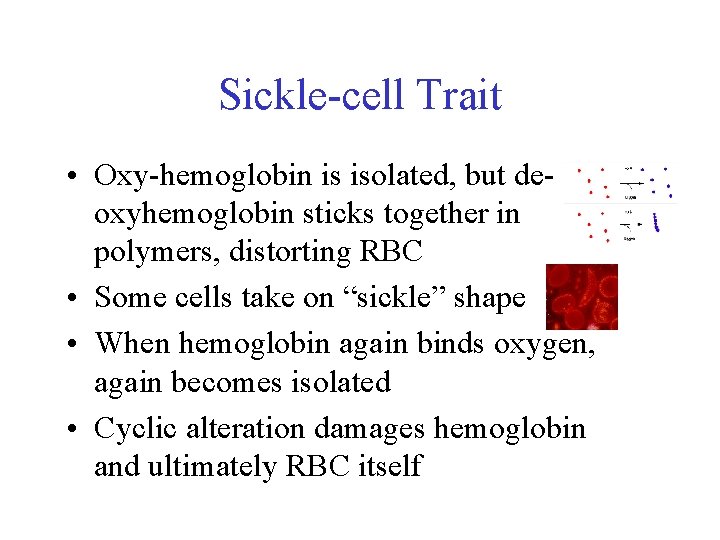
Sickle-cell Trait • Oxy-hemoglobin is isolated, but deoxyhemoglobin sticks together in polymers, distorting RBC • Some cells take on “sickle” shape • When hemoglobin again binds oxygen, again becomes isolated • Cyclic alteration damages hemoglobin and ultimately RBC itself

Protein: The Machinery of Life NH 2 -Val-His-Leu-Thr-Pro-Glu. Lys-Ser-Ala-Val-Thr-Ala-Leu-Trp. Gly-Lys-Val-Asn-Val-Asp-Glu-Val. Gly-Glu-…. . “Life is the mode of existence of proteins, and this mode of existence essentially consists in the constant selfrenewal of the chemical constituents of these substances. ” Friedrich Engles, 1878
- Slides: 103