PHSIOLOGY OF THE CARDIOVASCULAR SYSTEM Introduction Properties of
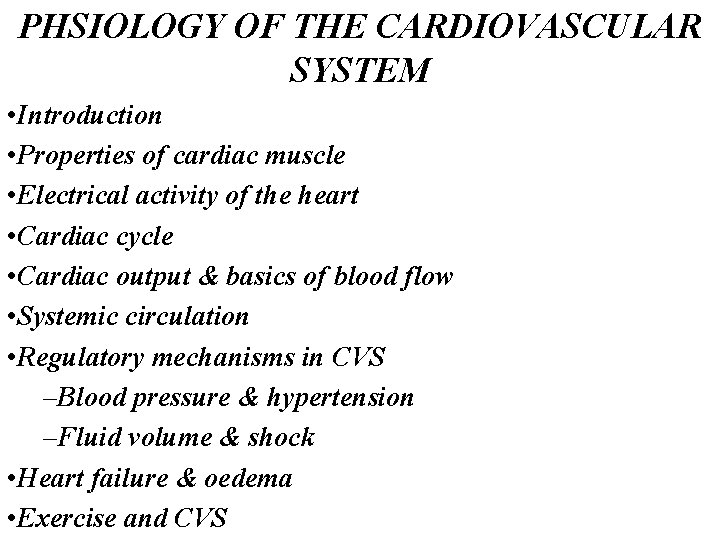
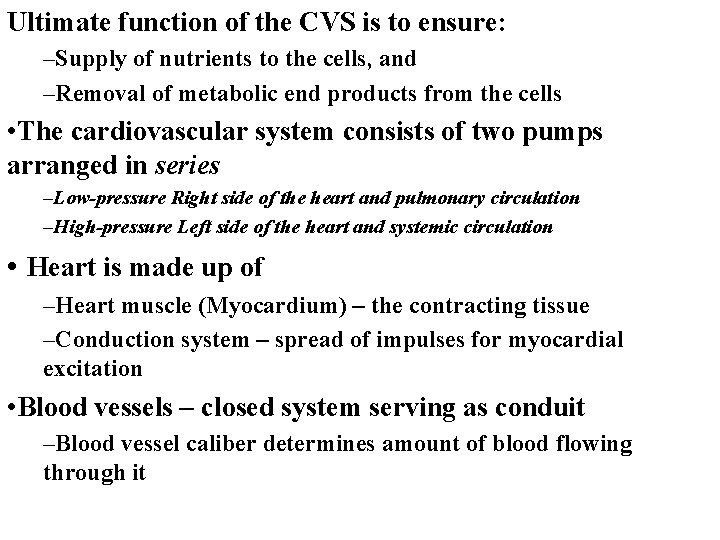
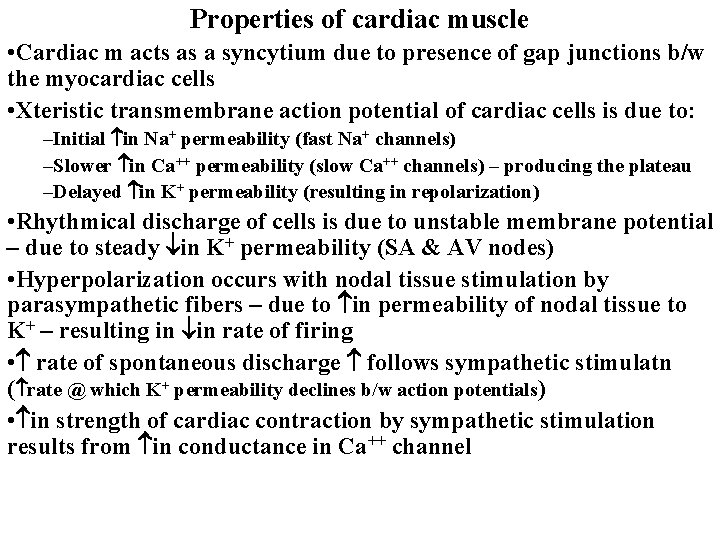
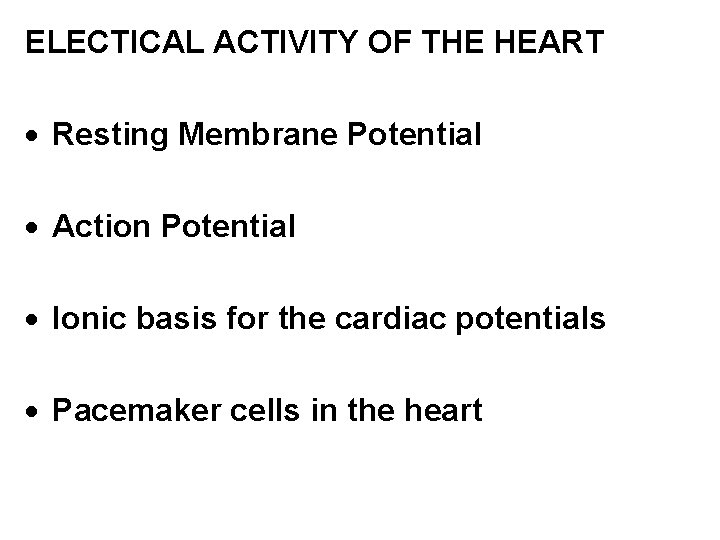
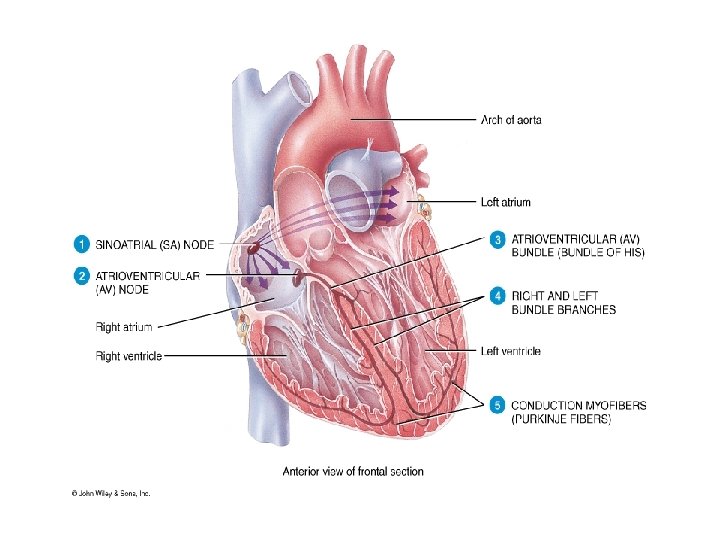
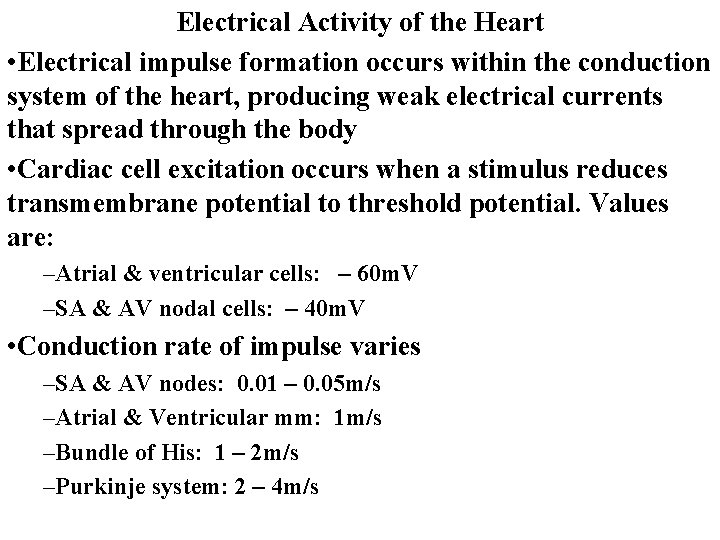
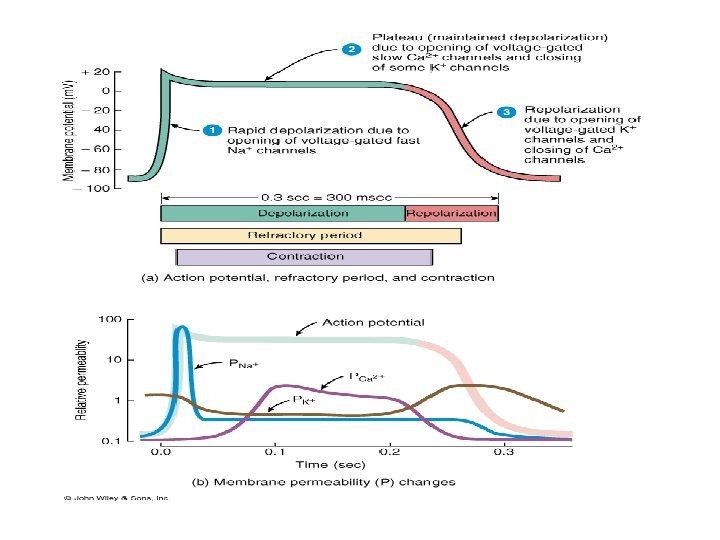
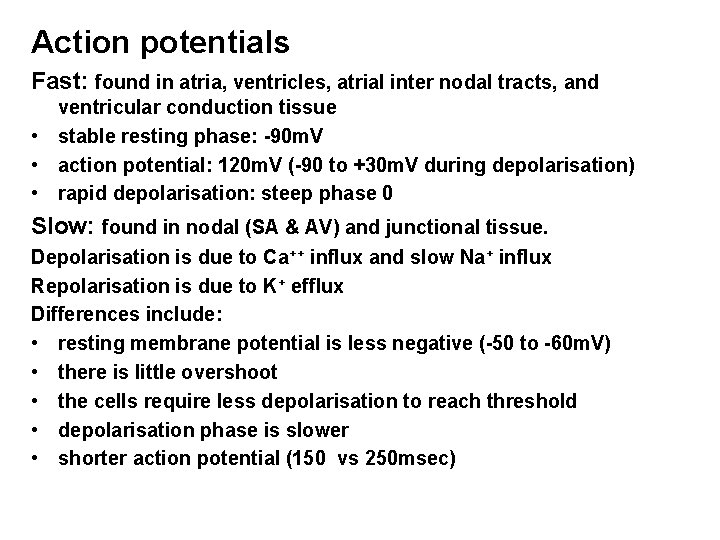
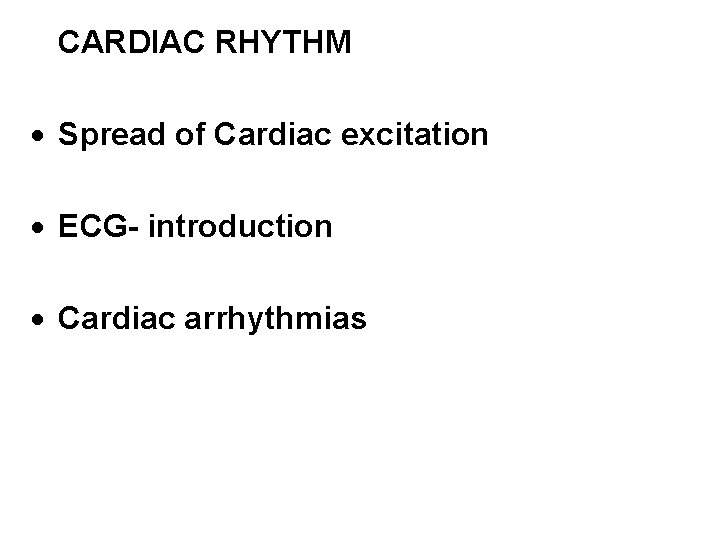
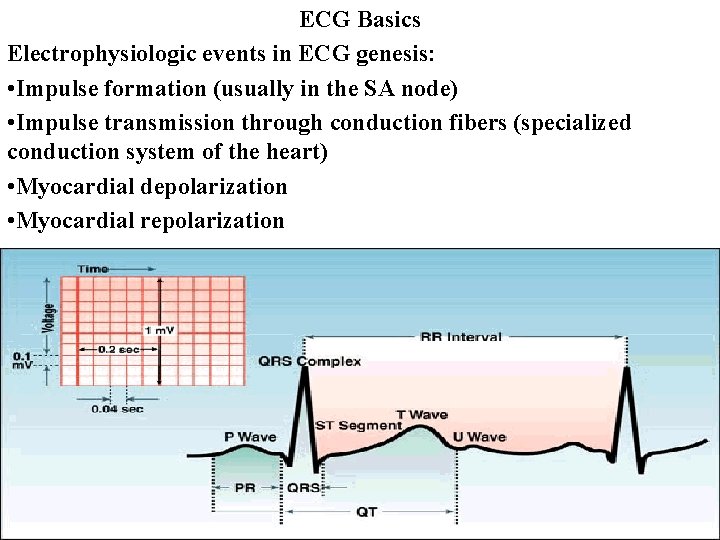
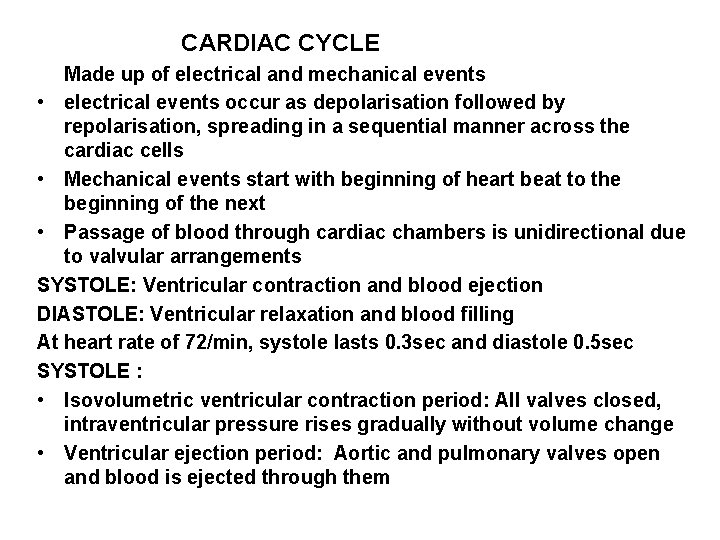
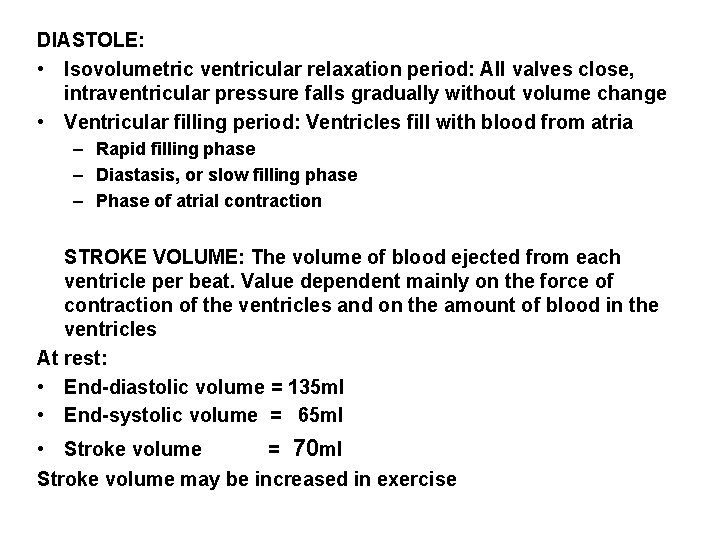
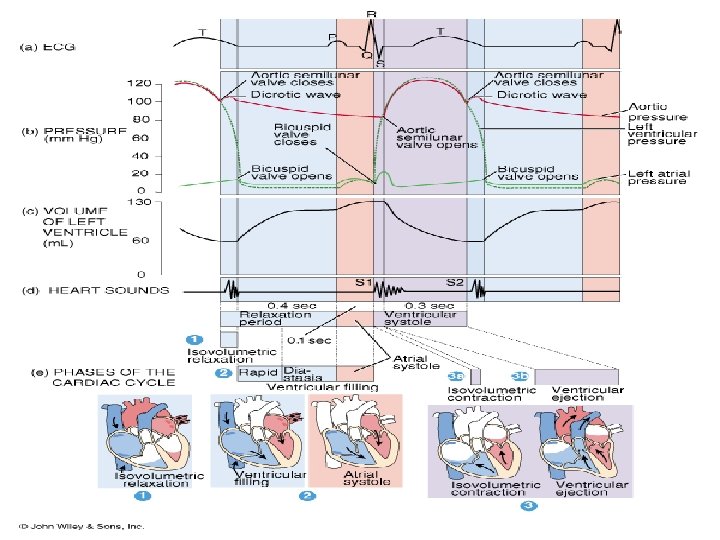
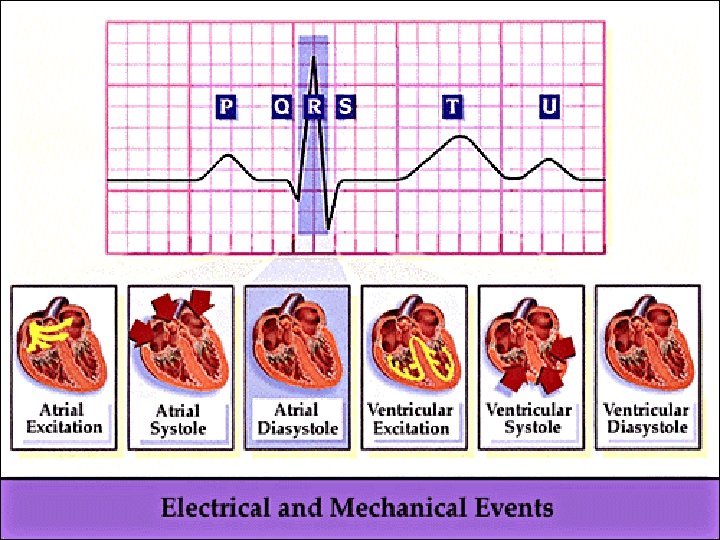
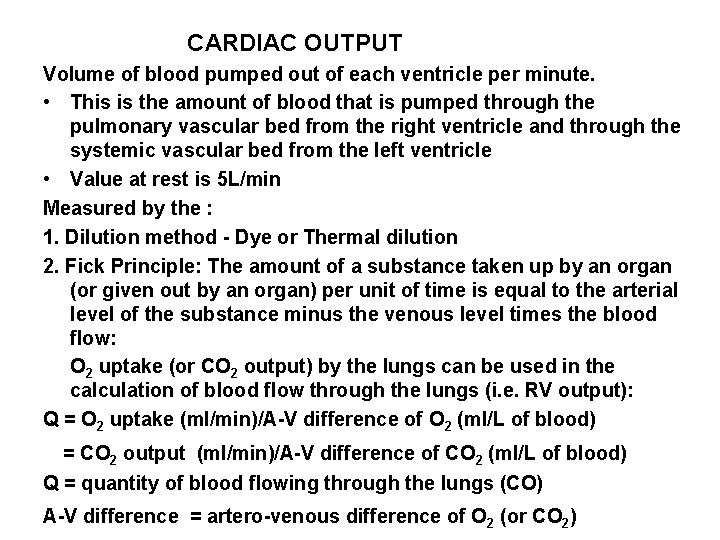
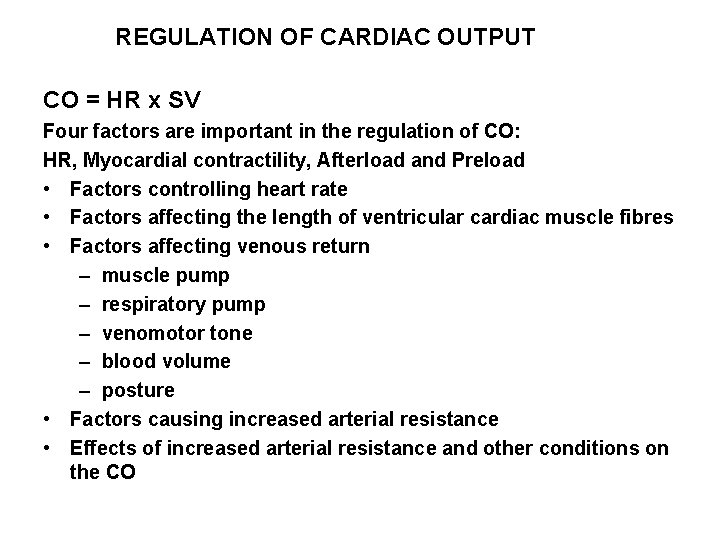
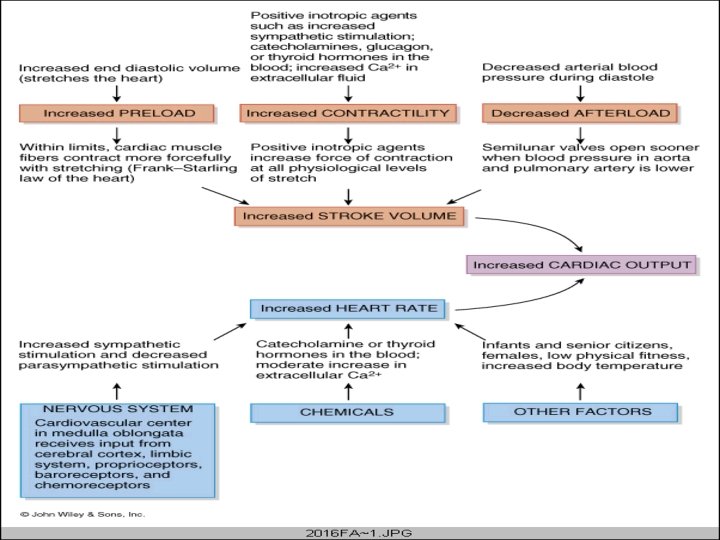
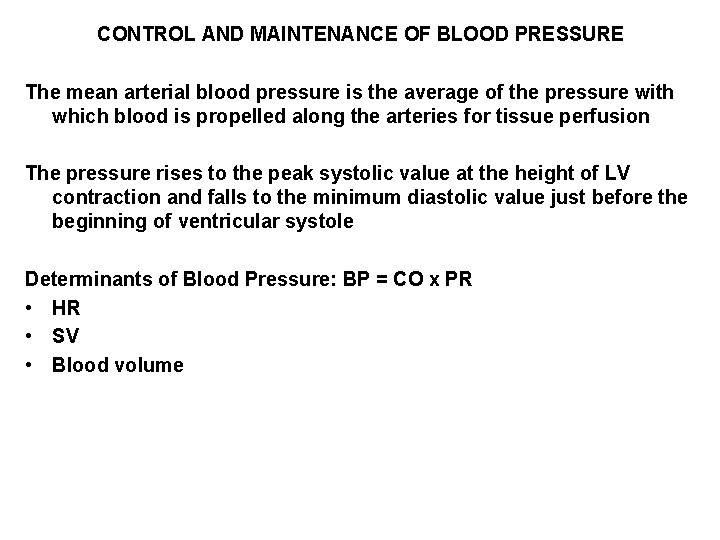
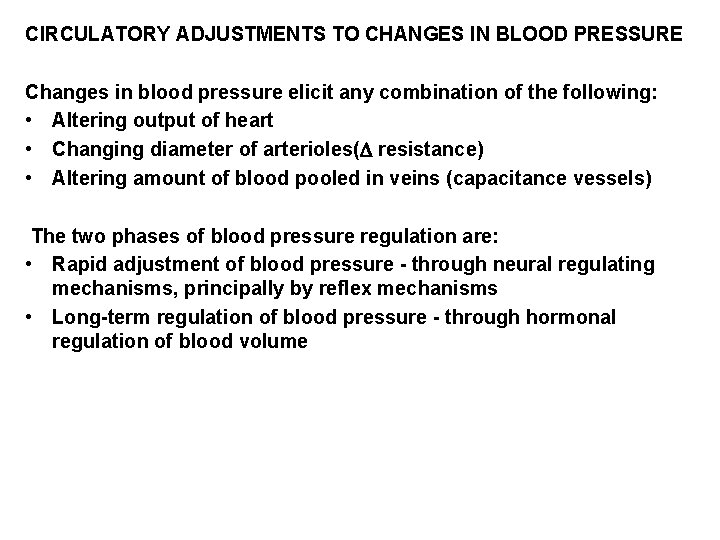
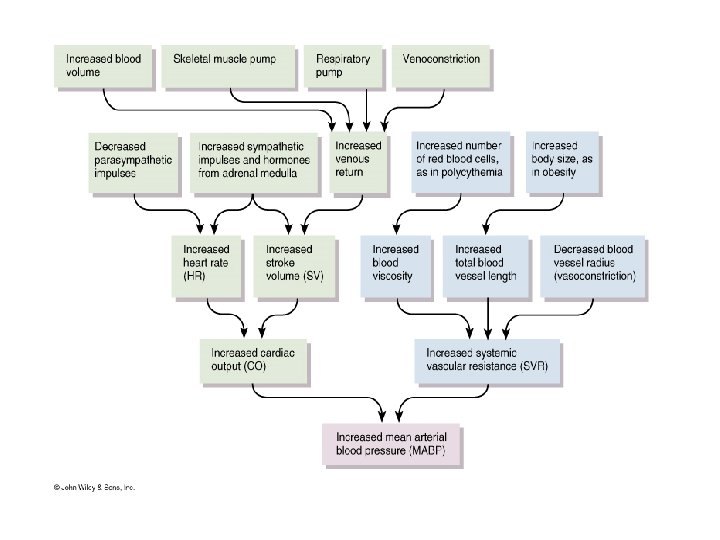
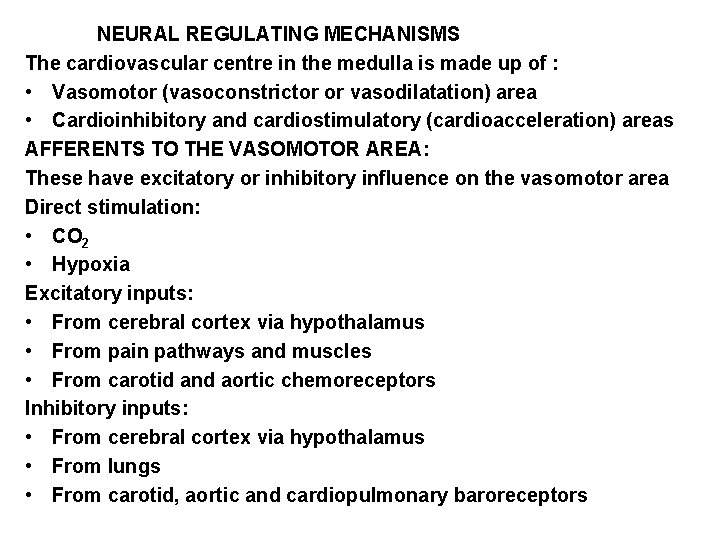
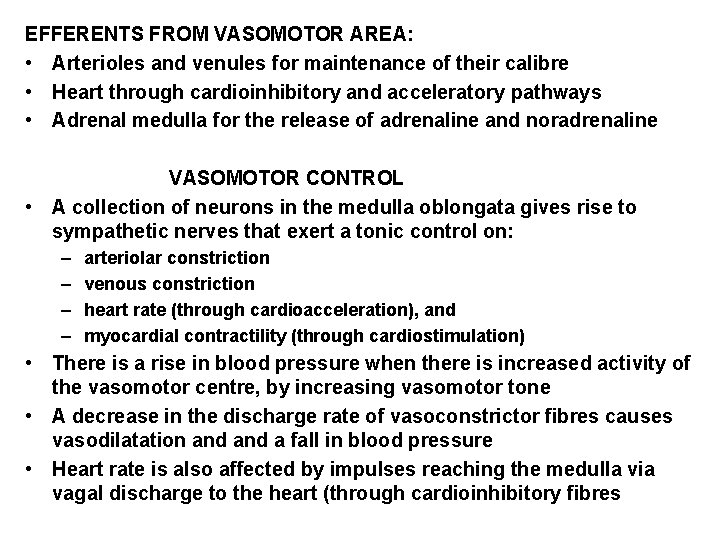
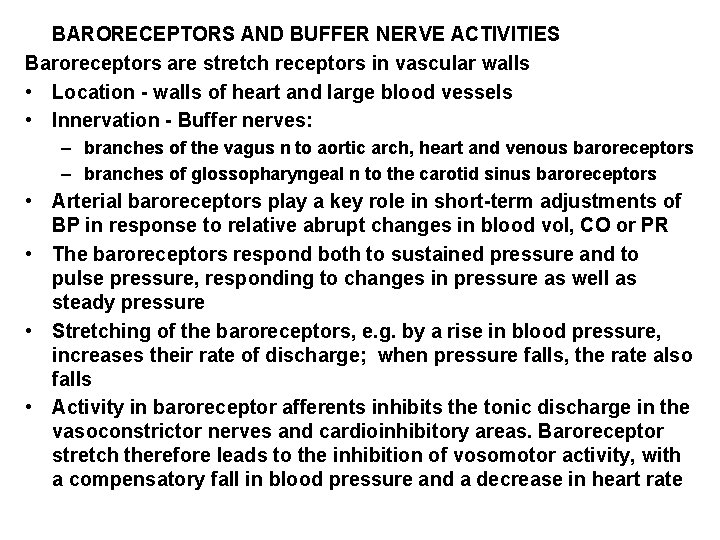
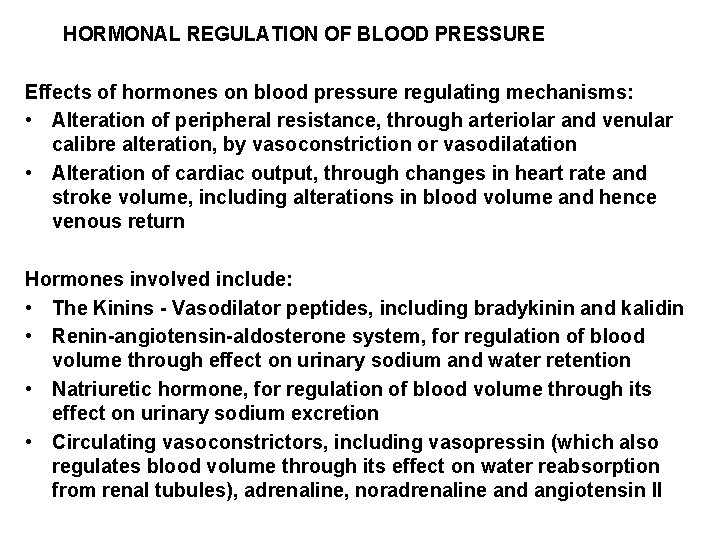
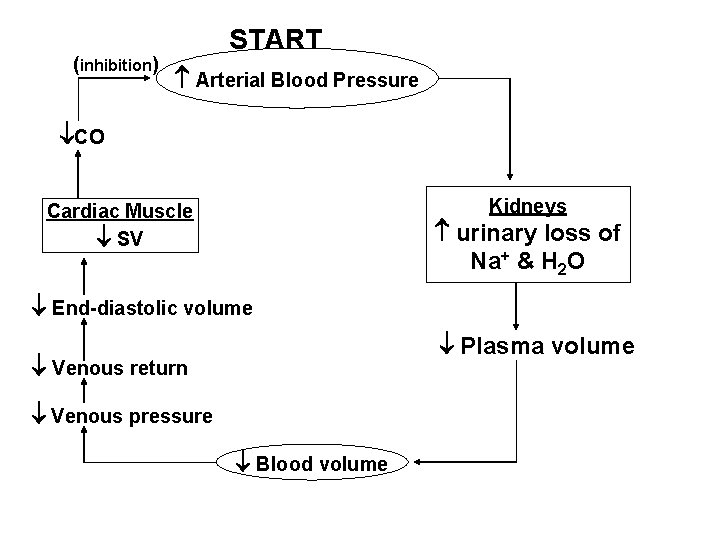
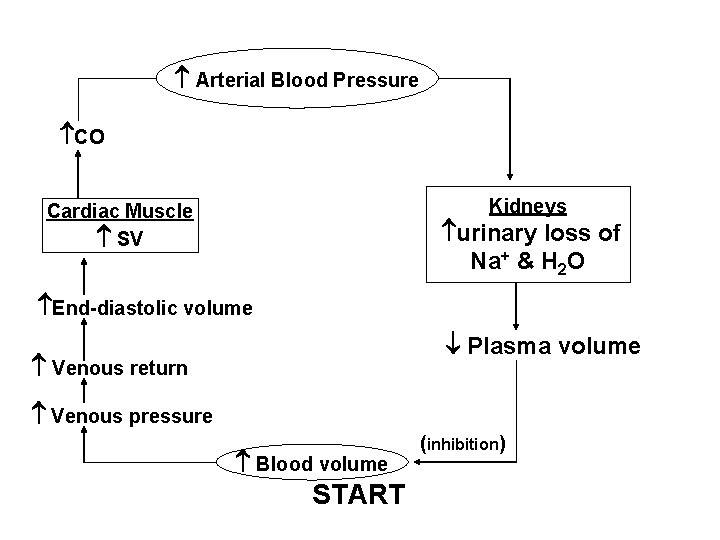
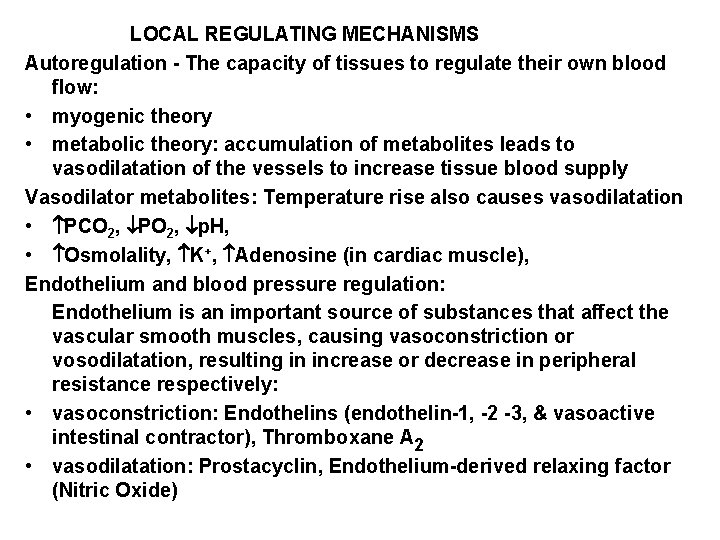
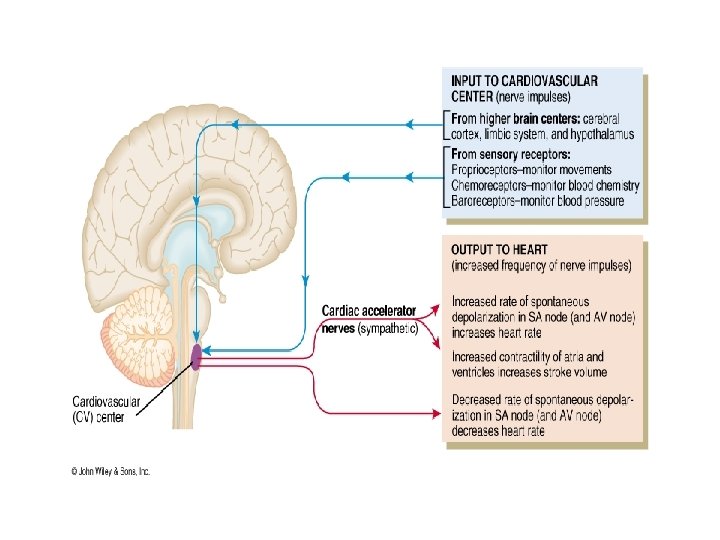
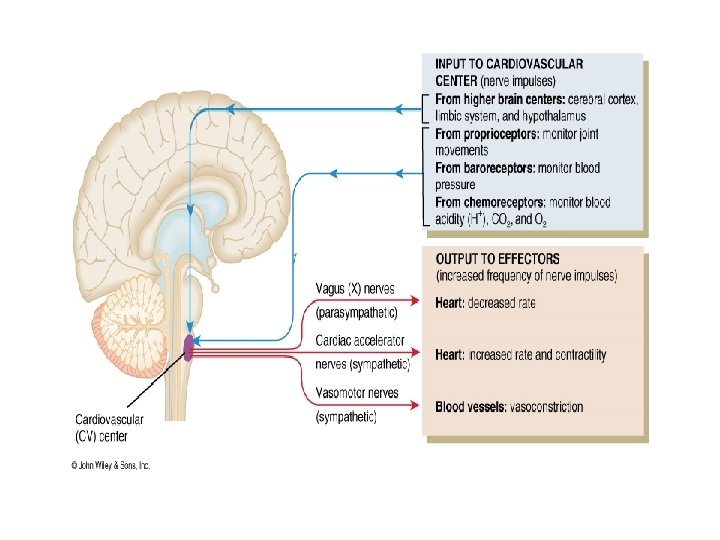
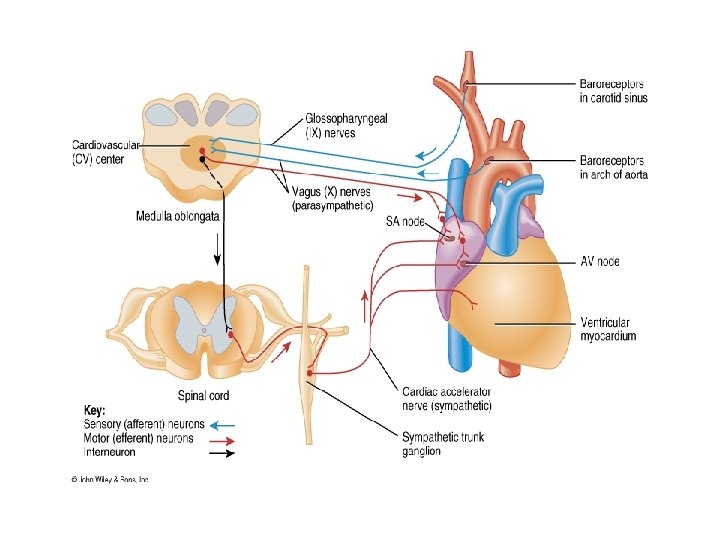
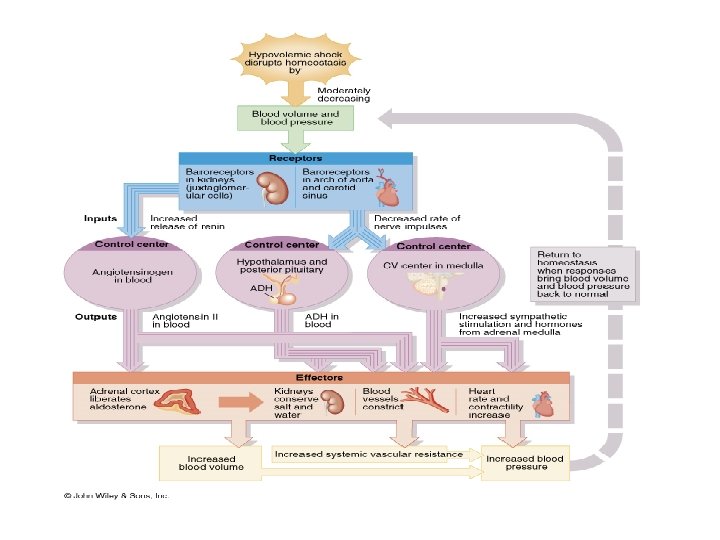
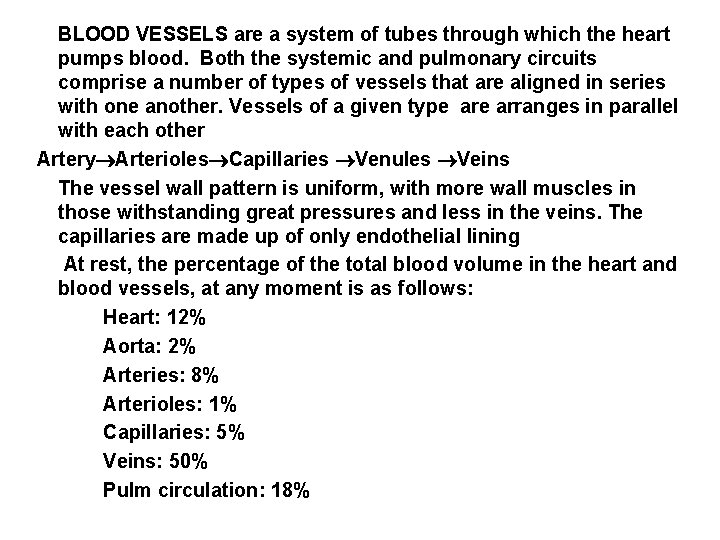
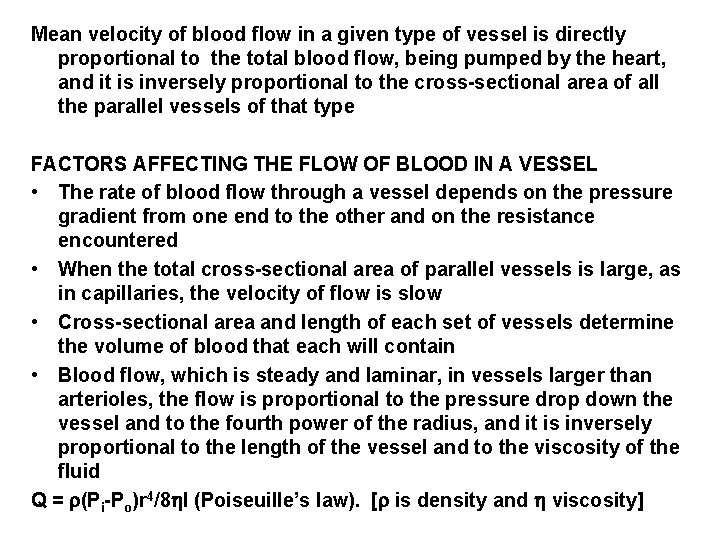
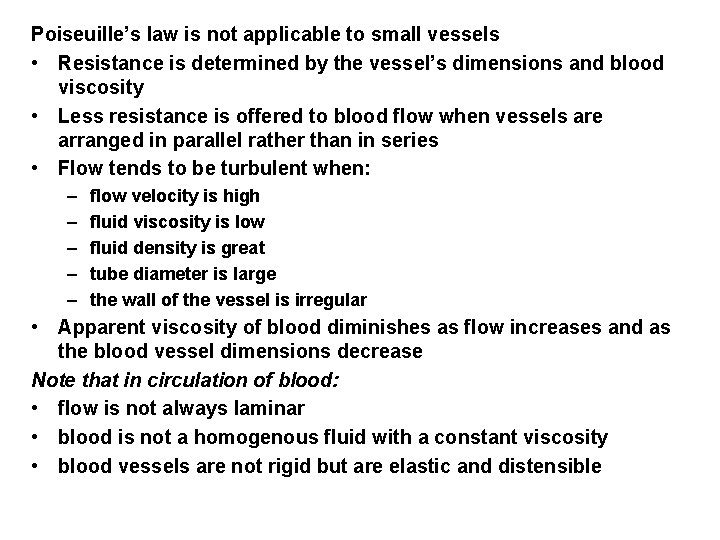
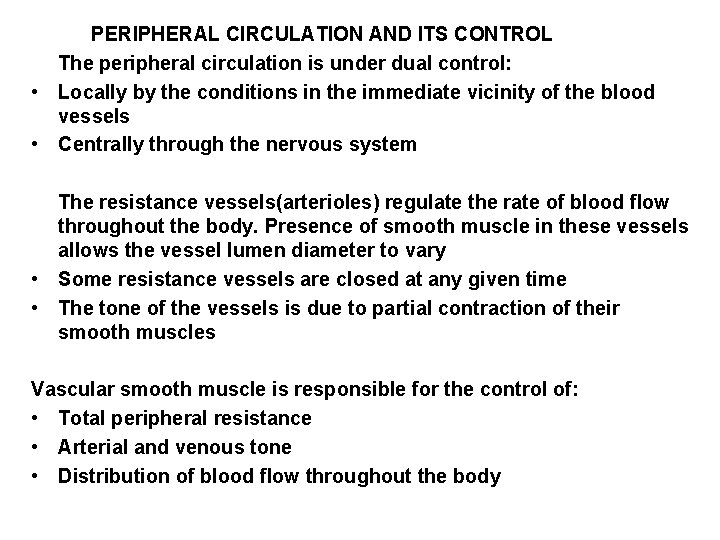
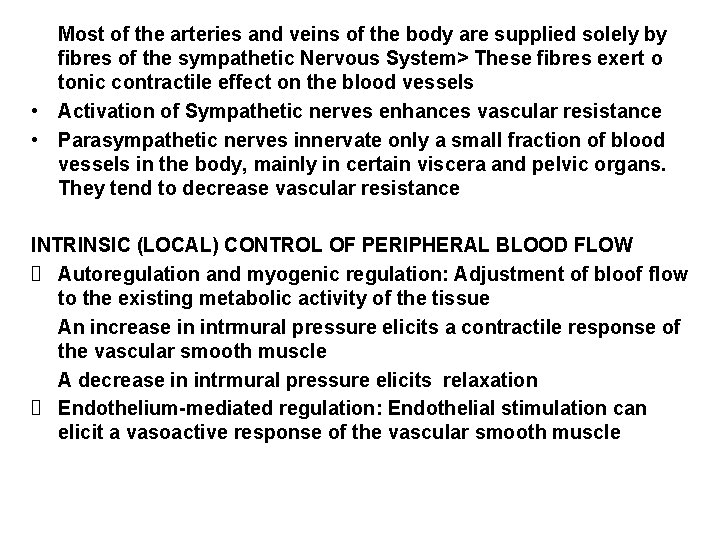
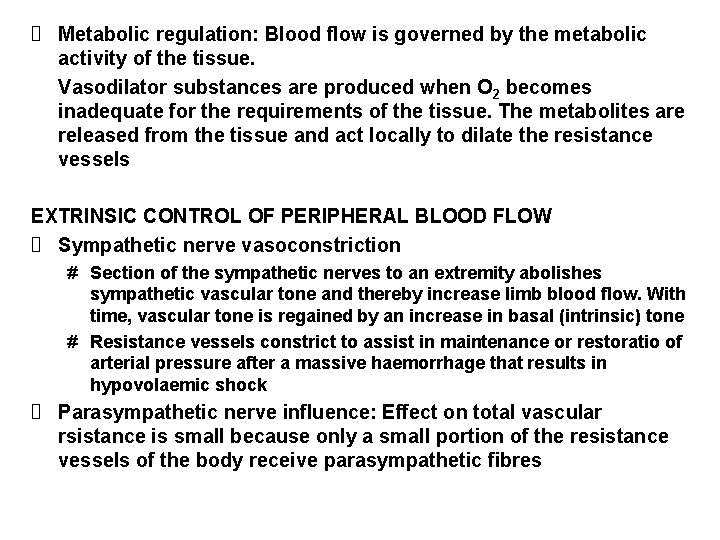
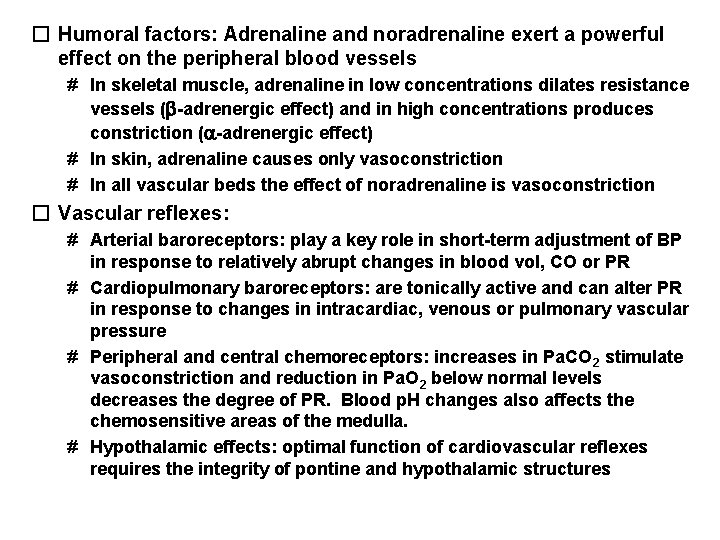
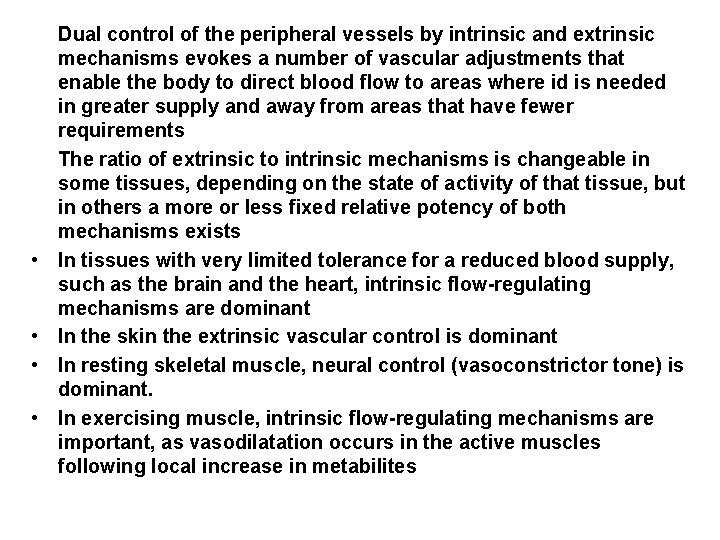
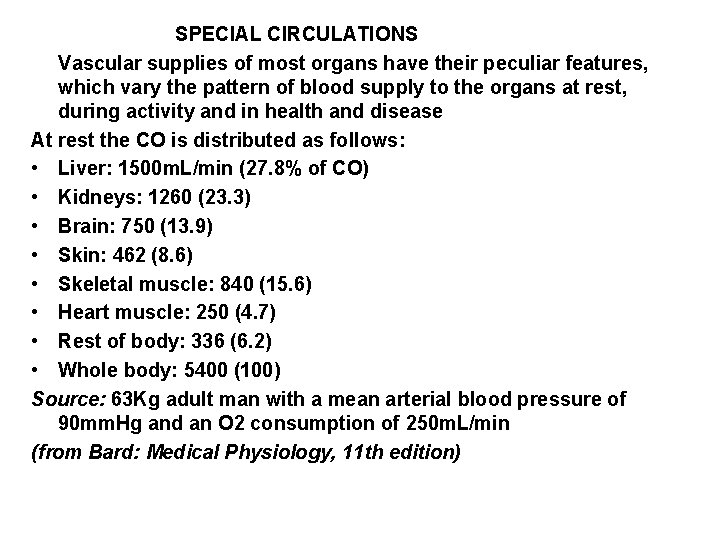
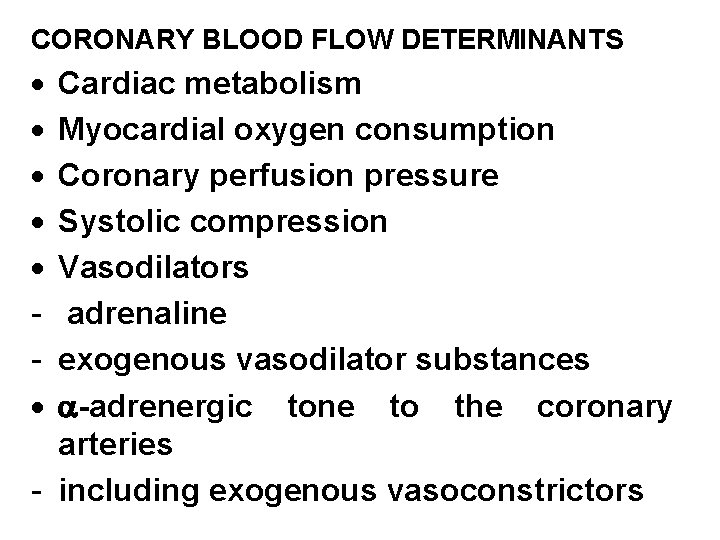
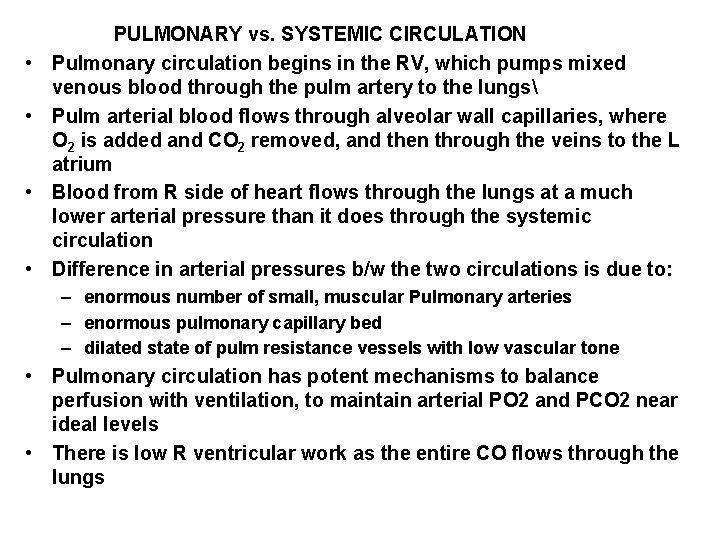
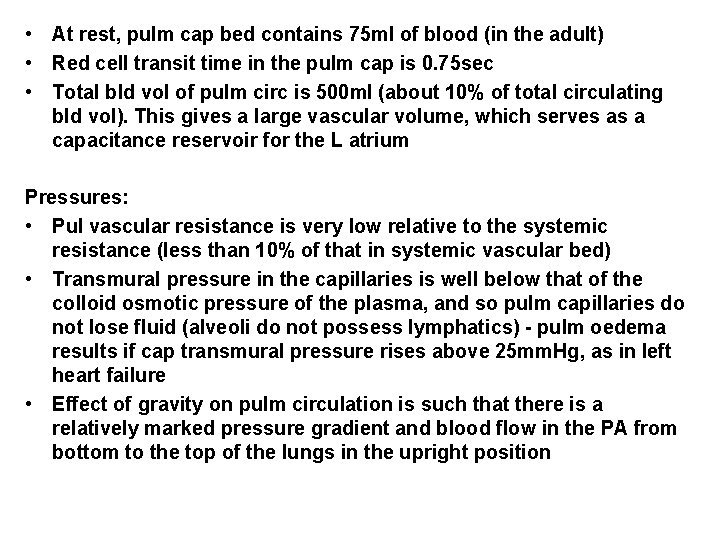
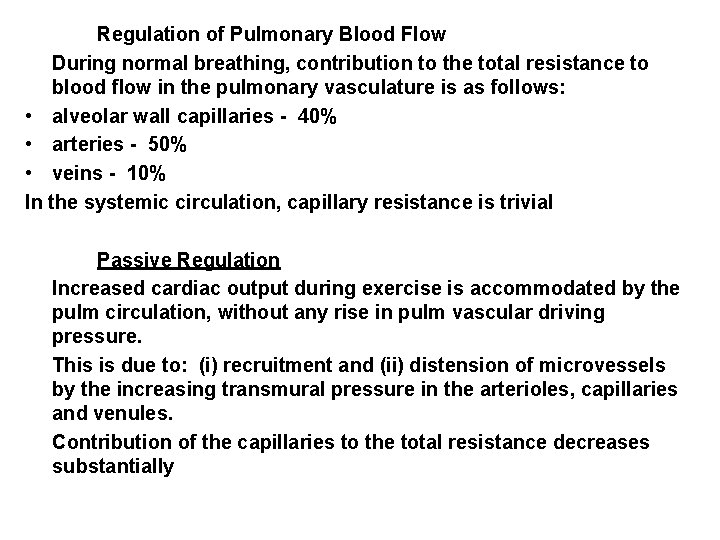
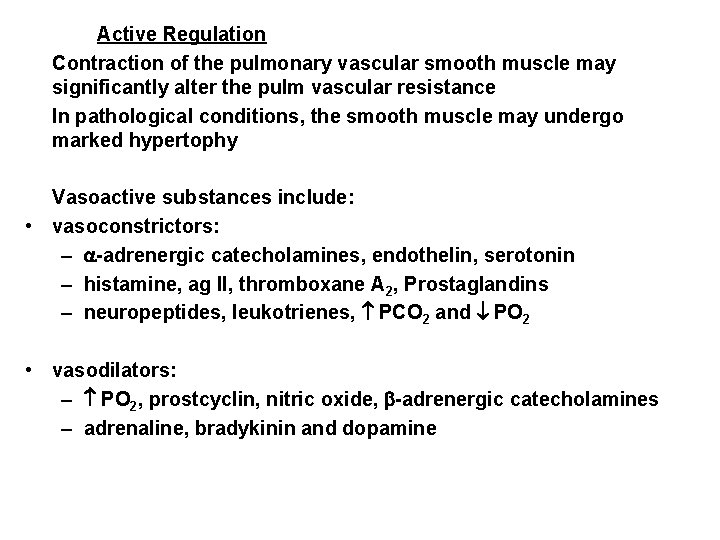
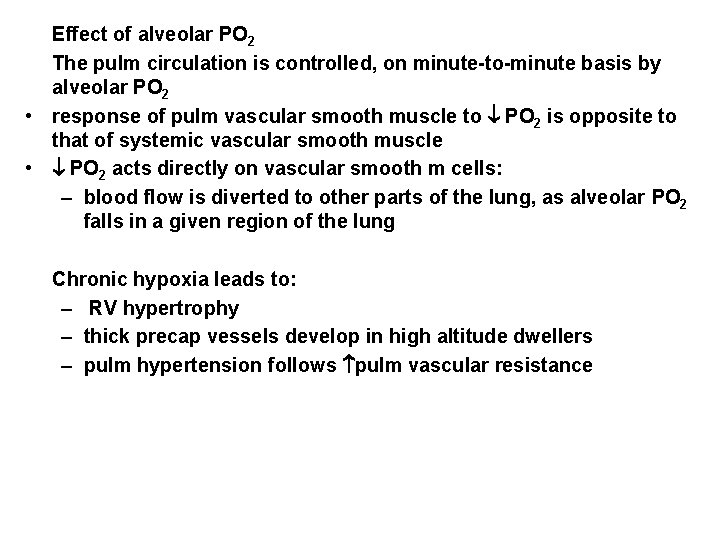
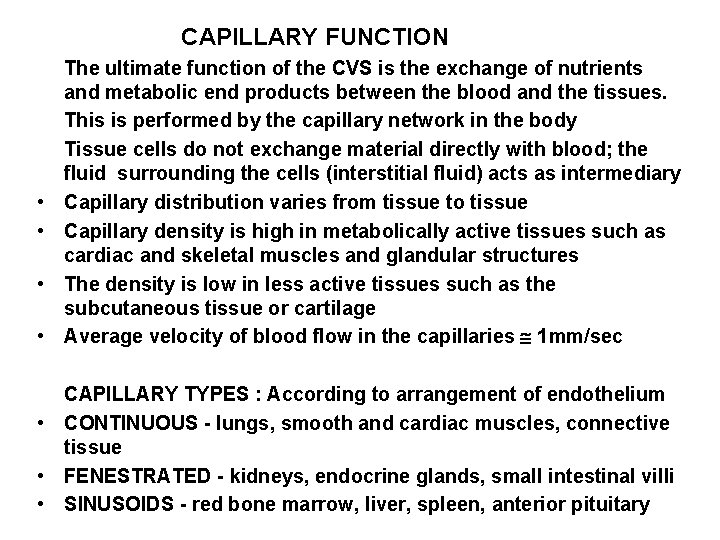
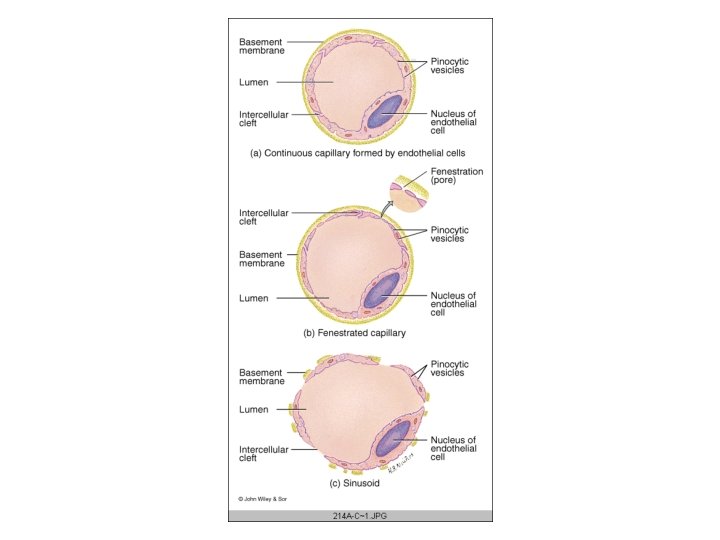
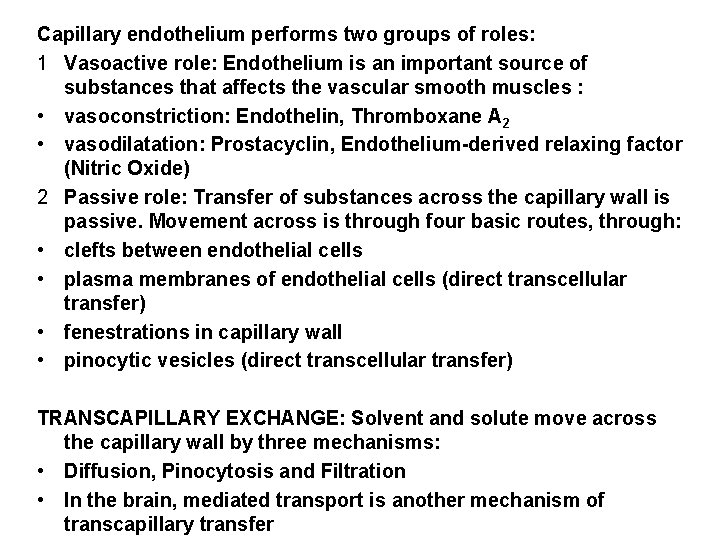
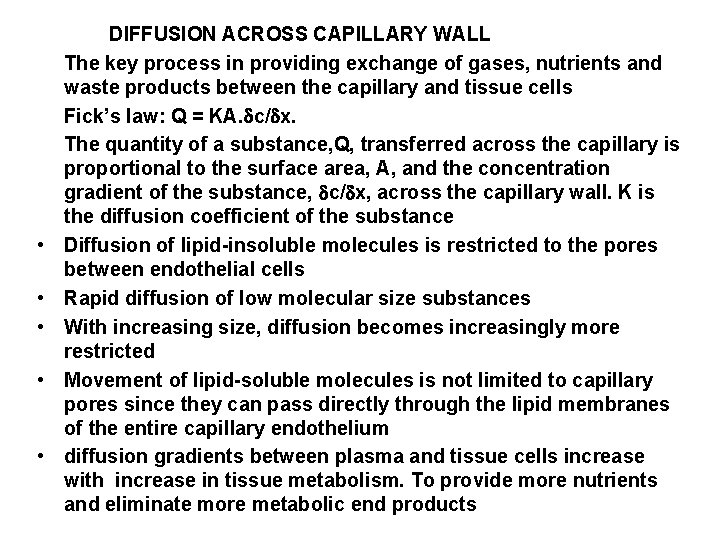
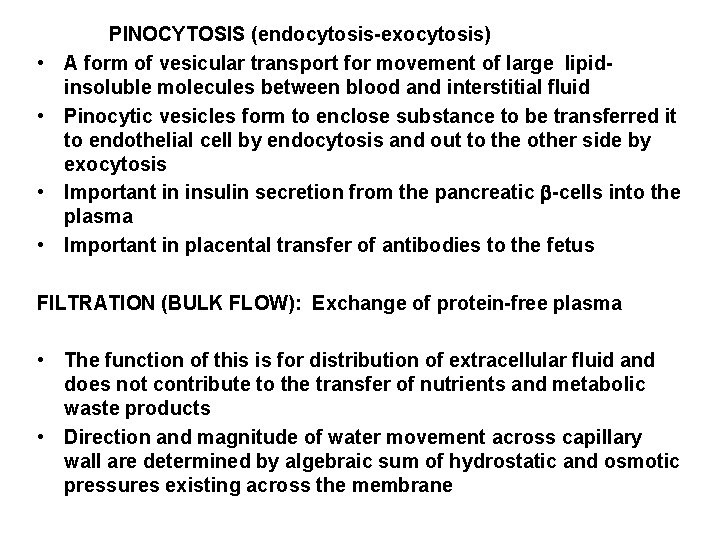
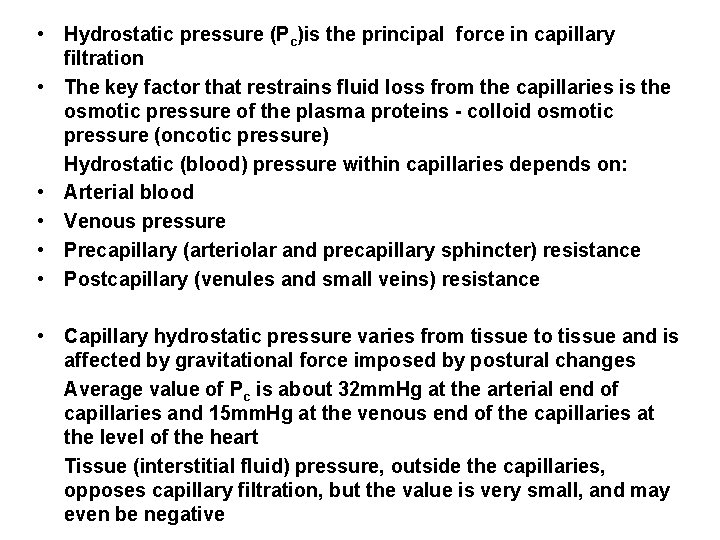
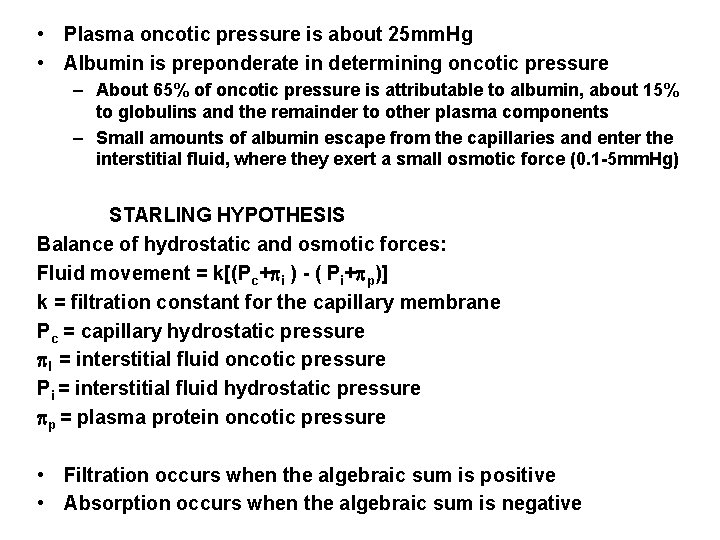
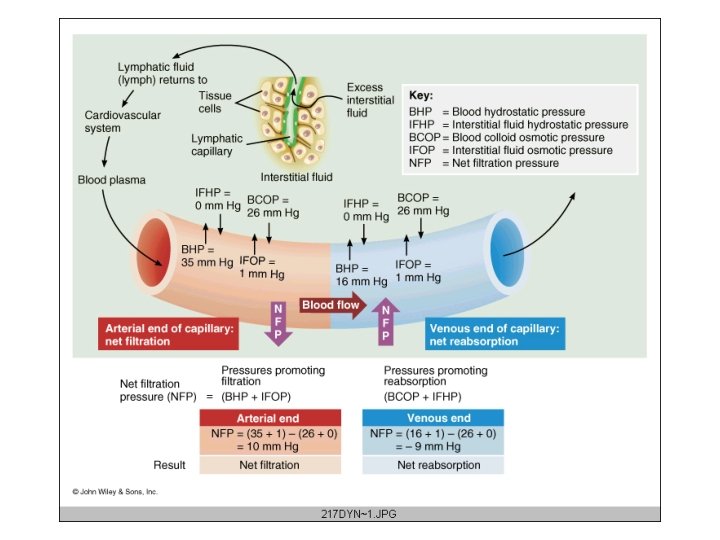
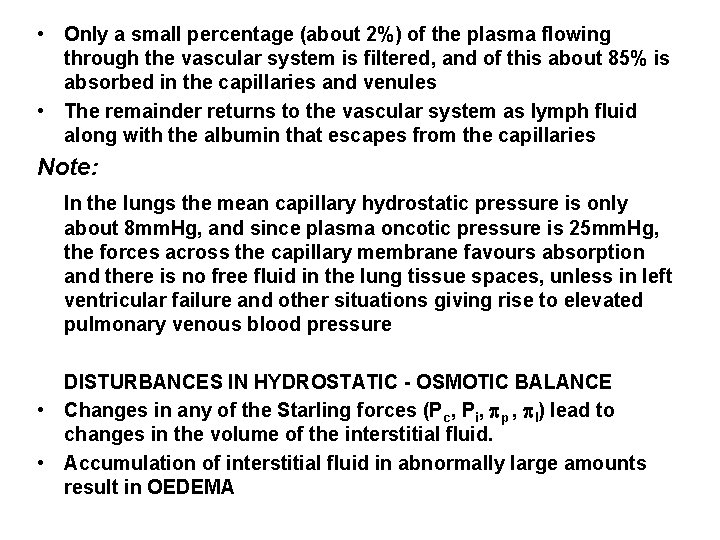
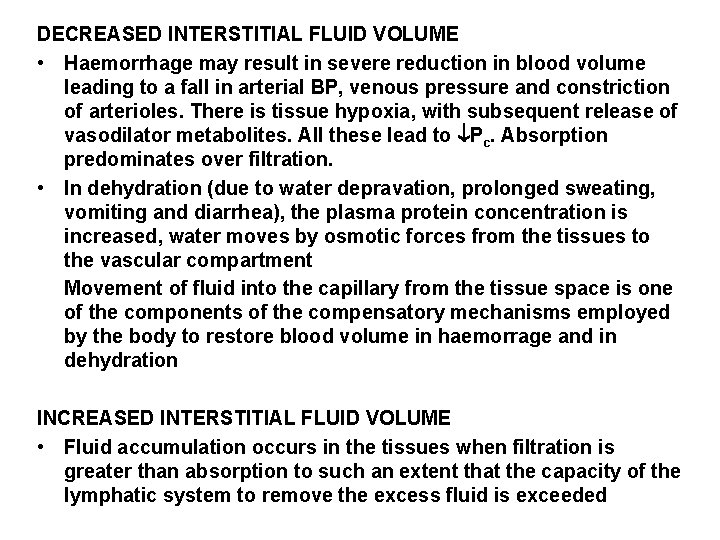
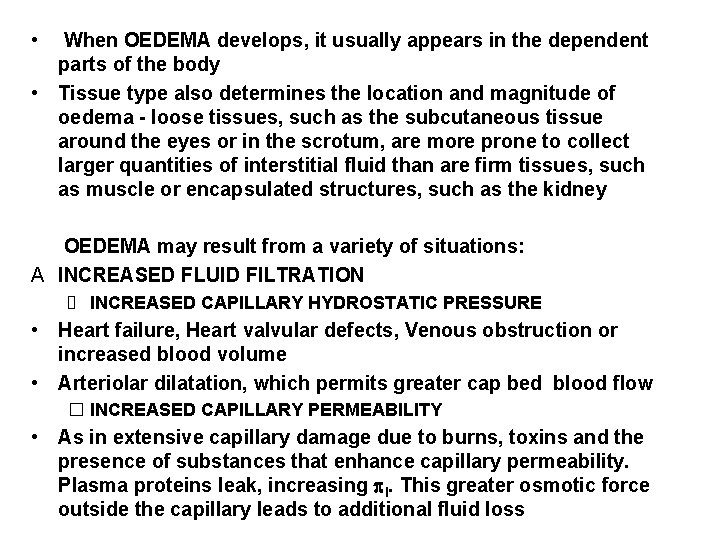
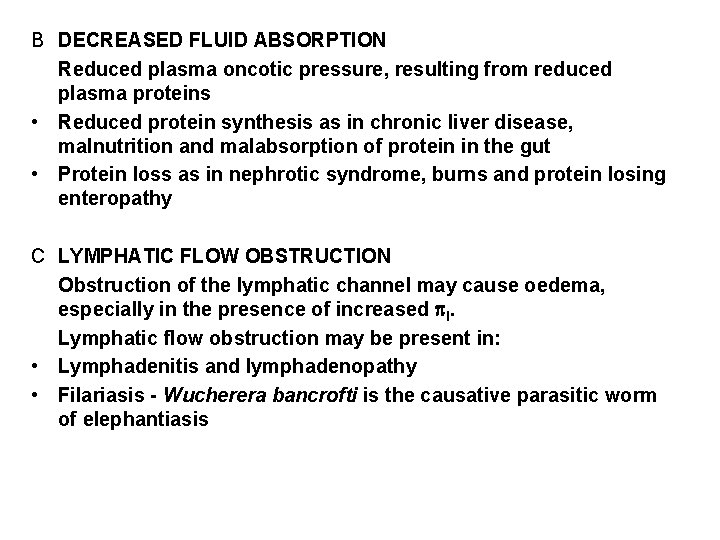
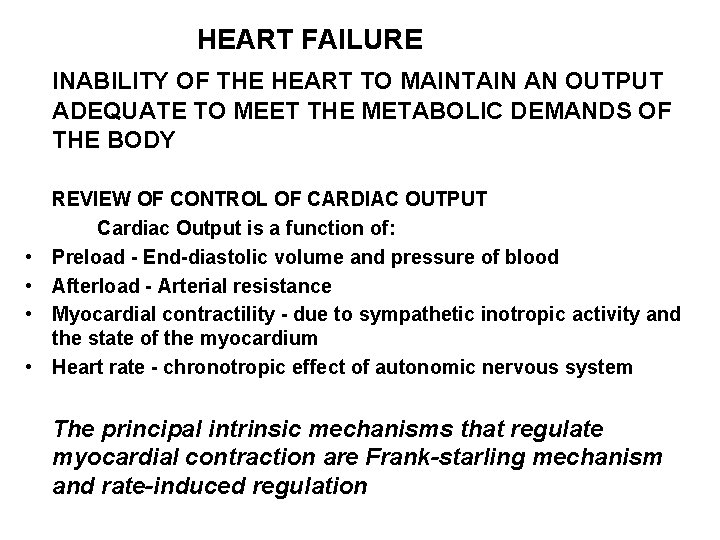
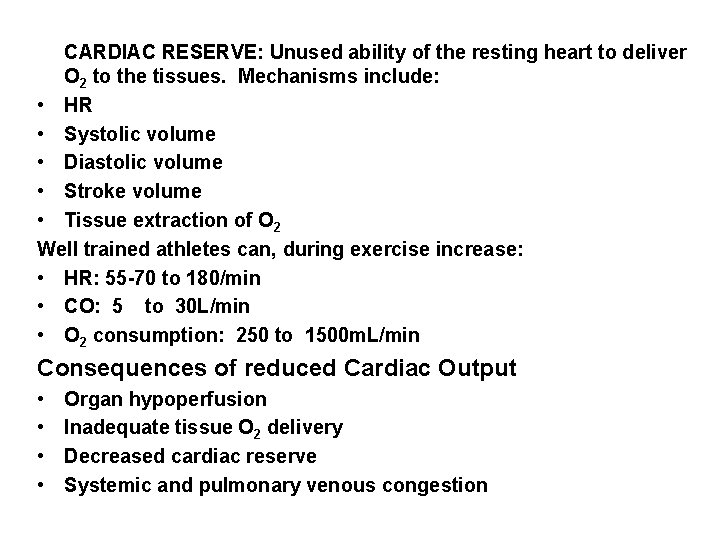
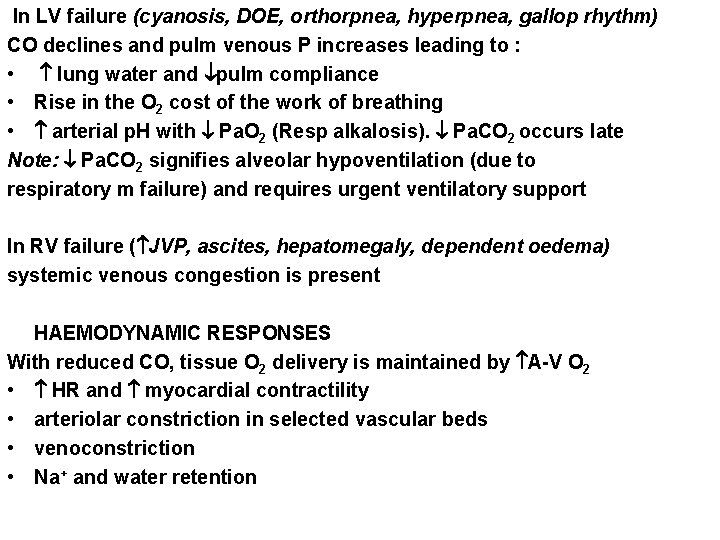
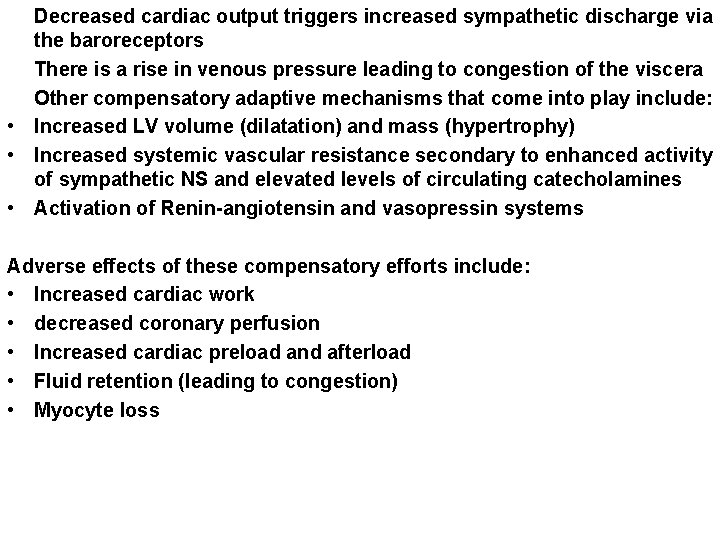
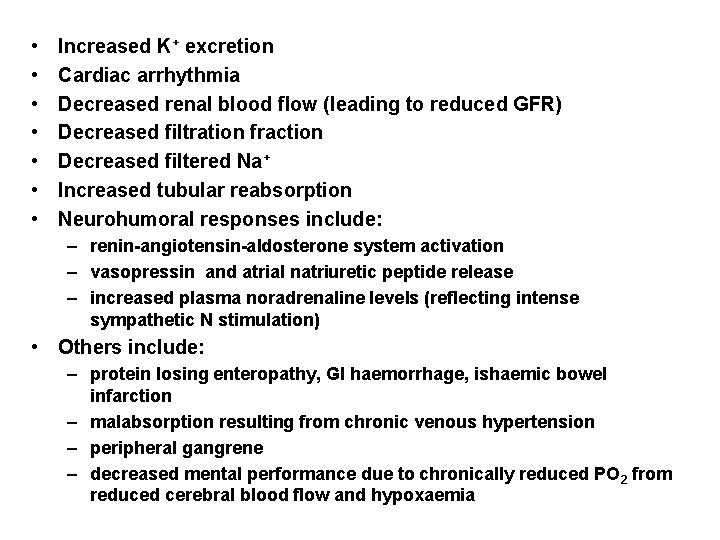
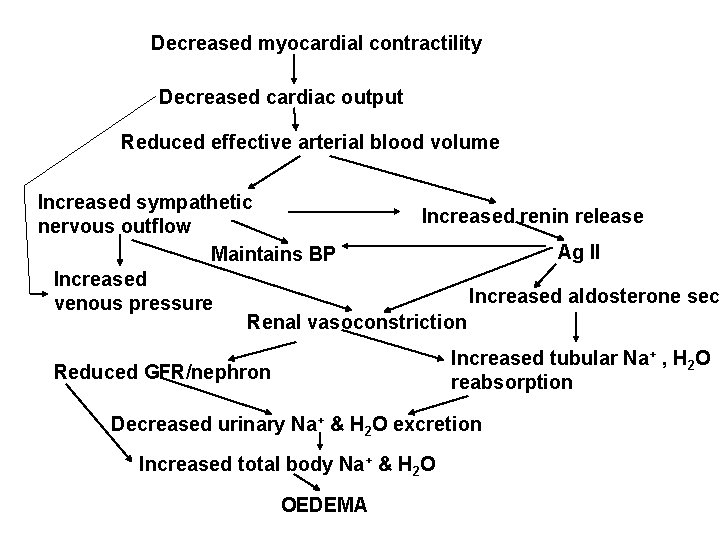
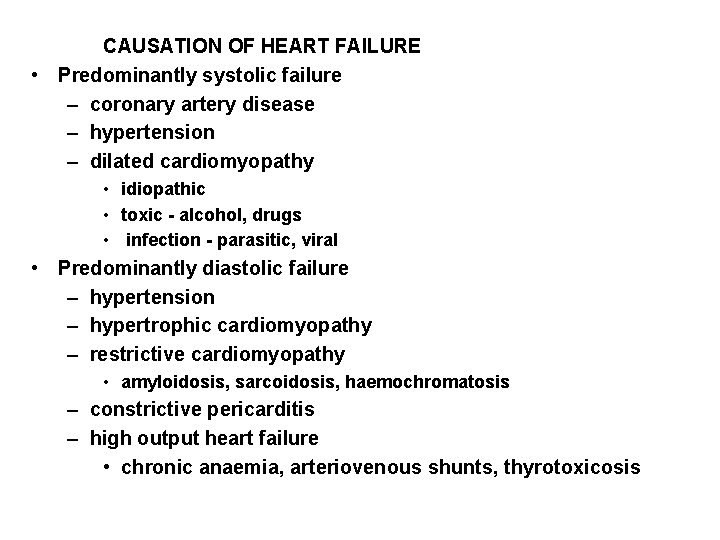
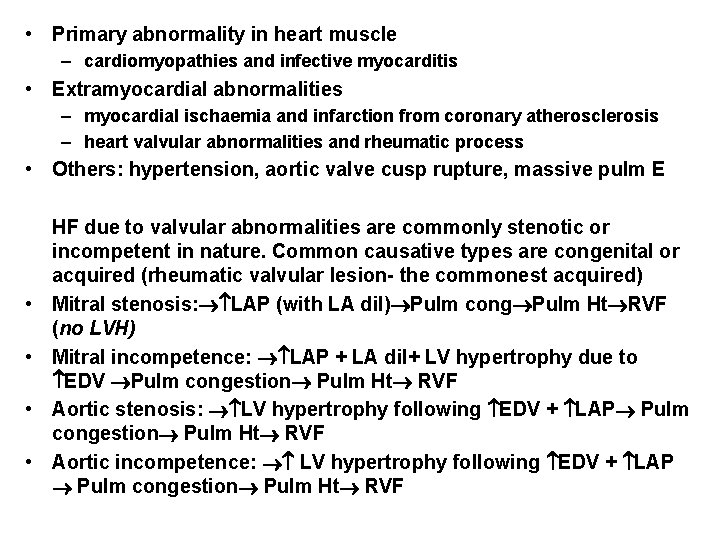
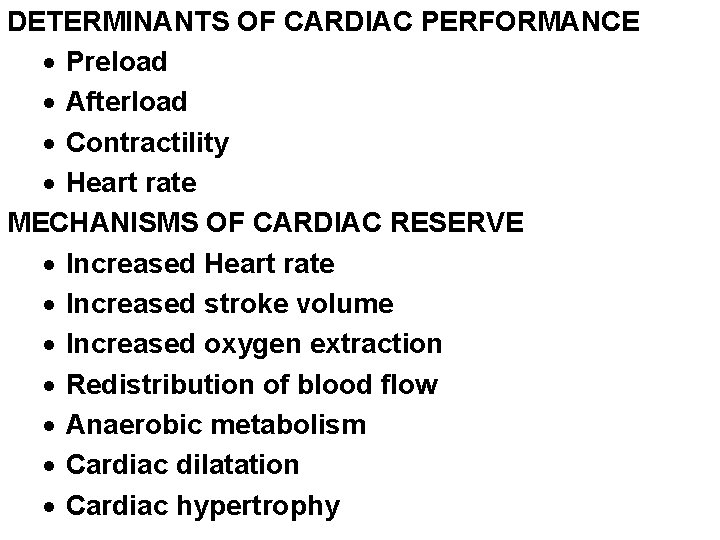
- Slides: 67
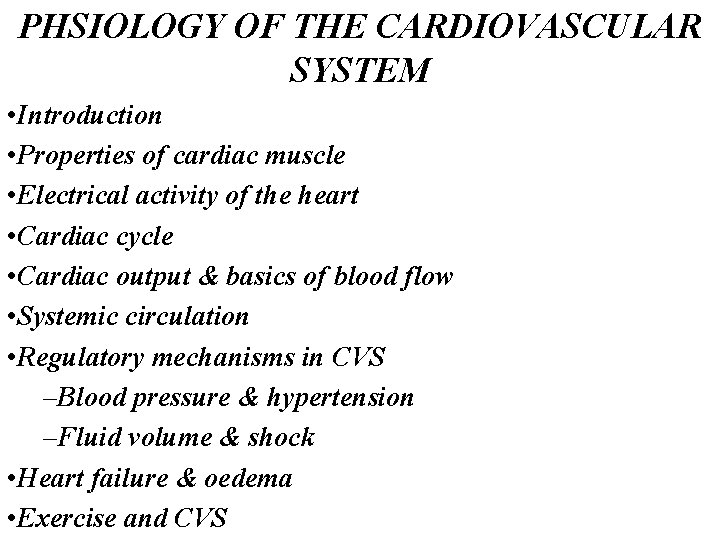
PHSIOLOGY OF THE CARDIOVASCULAR SYSTEM • Introduction • Properties of cardiac muscle • Electrical activity of the heart • Cardiac cycle • Cardiac output & basics of blood flow • Systemic circulation • Regulatory mechanisms in CVS –Blood pressure & hypertension –Fluid volume & shock • Heart failure & oedema • Exercise and CVS
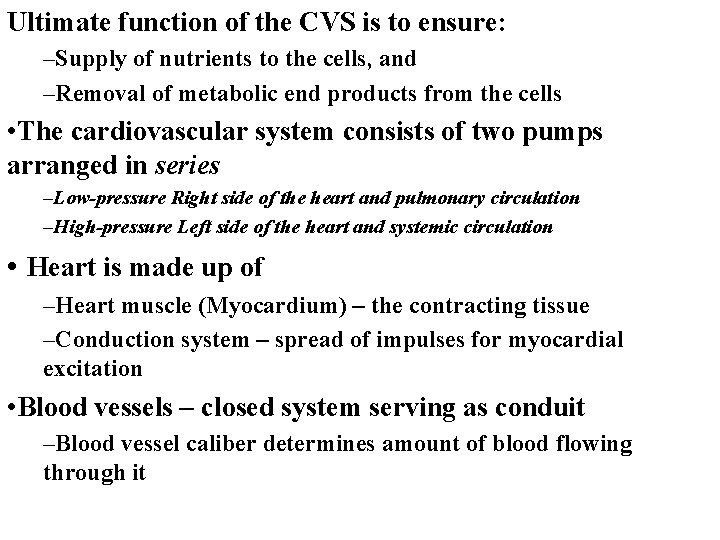
Ultimate function of the CVS is to ensure: –Supply of nutrients to the cells, and –Removal of metabolic end products from the cells • The cardiovascular system consists of two pumps arranged in series –Low-pressure Right side of the heart and pulmonary circulation –High-pressure Left side of the heart and systemic circulation • Heart is made up of –Heart muscle (Myocardium) – the contracting tissue –Conduction system – spread of impulses for myocardial excitation • Blood vessels – closed system serving as conduit –Blood vessel caliber determines amount of blood flowing through it
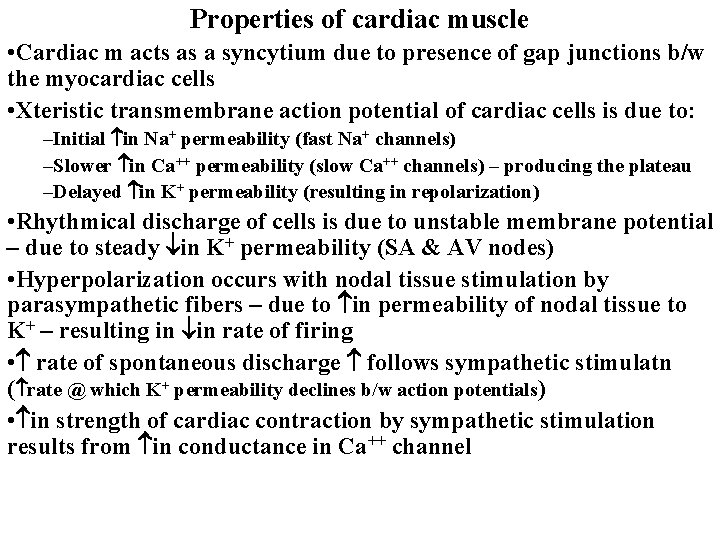
Properties of cardiac muscle • Cardiac m acts as a syncytium due to presence of gap junctions b/w the myocardiac cells • Xteristic transmembrane action potential of cardiac cells is due to: –Initial in Na+ permeability (fast Na+ channels) –Slower in Ca++ permeability (slow Ca++ channels) – producing the plateau –Delayed in K+ permeability (resulting in repolarization) • Rhythmical discharge of cells is due to unstable membrane potential – due to steady in K+ permeability (SA & AV nodes) • Hyperpolarization occurs with nodal tissue stimulation by parasympathetic fibers – due to in permeability of nodal tissue to K+ – resulting in in rate of firing • rate of spontaneous discharge follows sympathetic stimulatn ( rate @ which K+ permeability declines b/w action potentials) • in strength of cardiac contraction by sympathetic stimulation results from in conductance in Ca++ channel
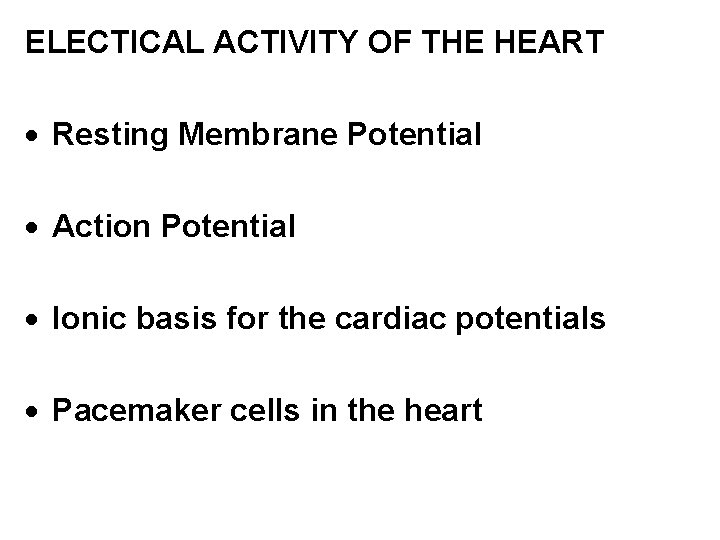
ELECTICAL ACTIVITY OF THE HEART · Resting Membrane Potential · Action Potential · Ionic basis for the cardiac potentials · Pacemaker cells in the heart
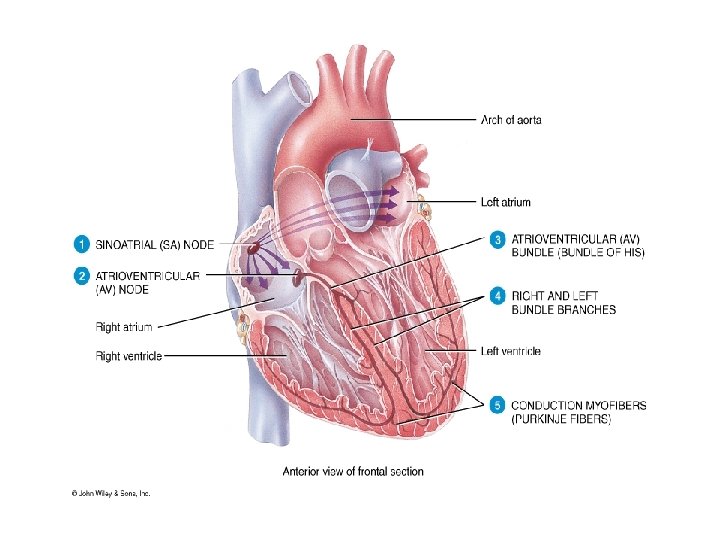
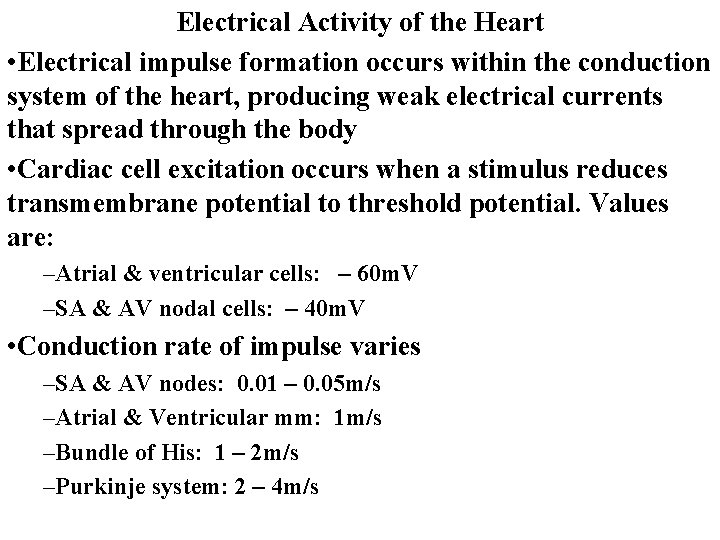
Electrical Activity of the Heart • Electrical impulse formation occurs within the conduction system of the heart, producing weak electrical currents that spread through the body • Cardiac cell excitation occurs when a stimulus reduces transmembrane potential to threshold potential. Values are: –Atrial & ventricular cells: – 60 m. V –SA & AV nodal cells: – 40 m. V • Conduction rate of impulse varies –SA & AV nodes: 0. 01 – 0. 05 m/s –Atrial & Ventricular mm: 1 m/s –Bundle of His: 1 – 2 m/s –Purkinje system: 2 – 4 m/s
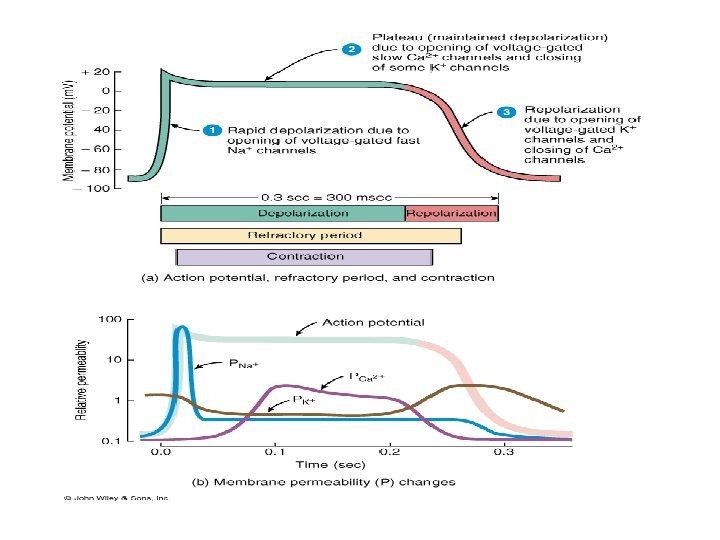
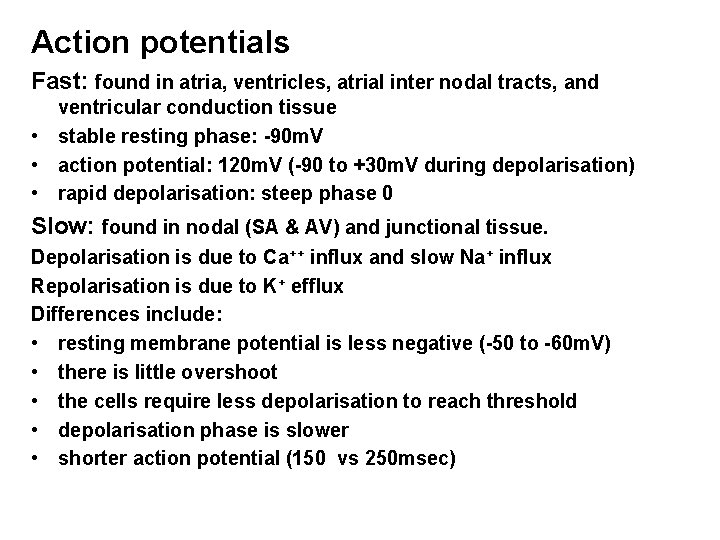
Action potentials Fast: found in atria, ventricles, atrial inter nodal tracts, and ventricular conduction tissue • stable resting phase: -90 m. V • action potential: 120 m. V (-90 to +30 m. V during depolarisation) • rapid depolarisation: steep phase 0 Slow: found in nodal (SA & AV) and junctional tissue. Depolarisation is due to Ca++ influx and slow Na+ influx Repolarisation is due to K+ efflux Differences include: • resting membrane potential is less negative (-50 to -60 m. V) • there is little overshoot • the cells require less depolarisation to reach threshold • depolarisation phase is slower • shorter action potential (150 vs 250 msec)
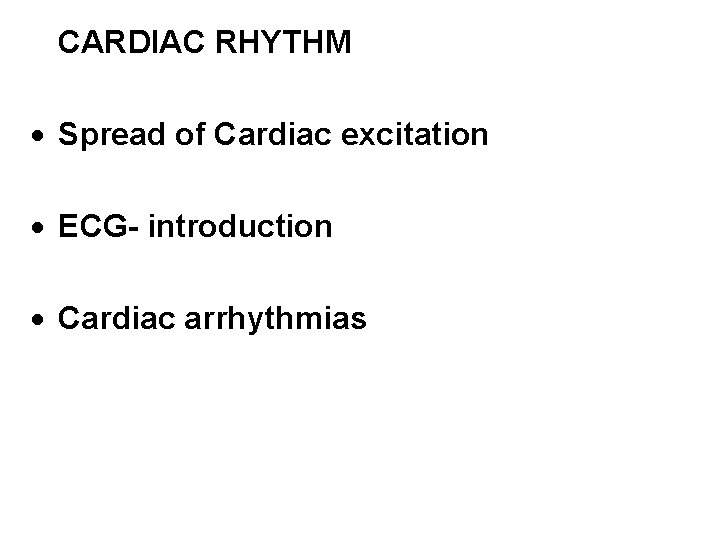
CARDIAC RHYTHM · Spread of Cardiac excitation · ECG- introduction · Cardiac arrhythmias
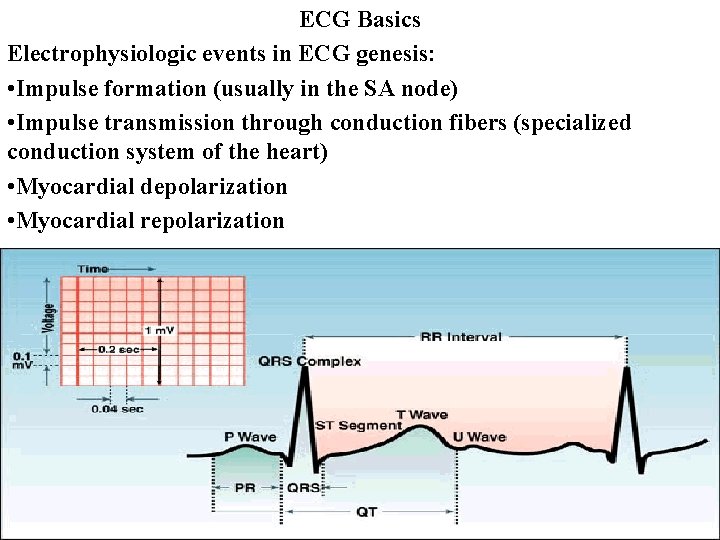
ECG Basics Electrophysiologic events in ECG genesis: • Impulse formation (usually in the SA node) • Impulse transmission through conduction fibers (specialized conduction system of the heart) • Myocardial depolarization • Myocardial repolarization
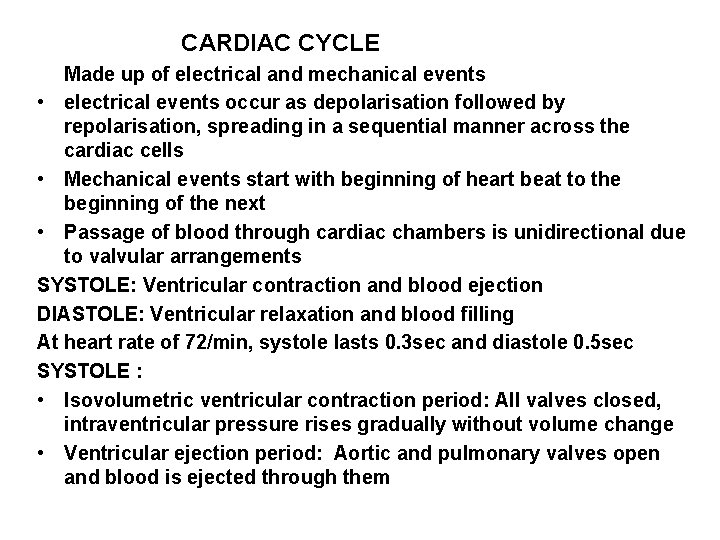
CARDIAC CYCLE Made up of electrical and mechanical events • electrical events occur as depolarisation followed by repolarisation, spreading in a sequential manner across the cardiac cells • Mechanical events start with beginning of heart beat to the beginning of the next • Passage of blood through cardiac chambers is unidirectional due to valvular arrangements SYSTOLE: Ventricular contraction and blood ejection DIASTOLE: Ventricular relaxation and blood filling At heart rate of 72/min, systole lasts 0. 3 sec and diastole 0. 5 sec SYSTOLE : • Isovolumetric ventricular contraction period: All valves closed, intraventricular pressure rises gradually without volume change • Ventricular ejection period: Aortic and pulmonary valves open and blood is ejected through them
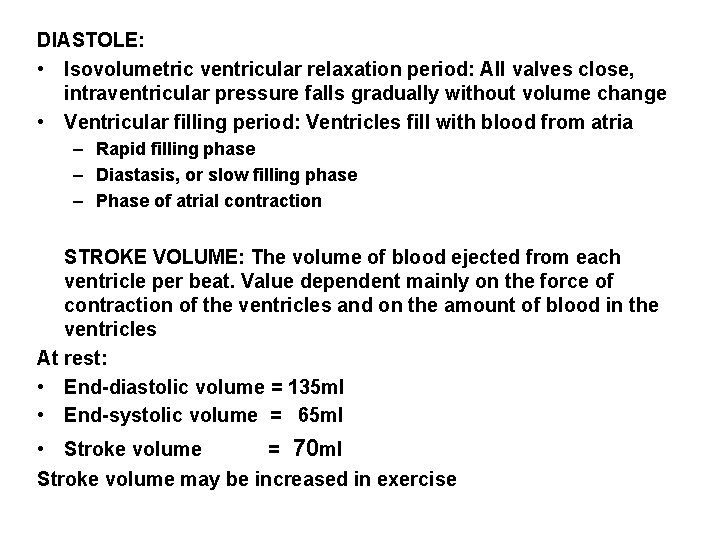
DIASTOLE: • Isovolumetric ventricular relaxation period: All valves close, intraventricular pressure falls gradually without volume change • Ventricular filling period: Ventricles fill with blood from atria – Rapid filling phase – Diastasis, or slow filling phase – Phase of atrial contraction STROKE VOLUME: The volume of blood ejected from each ventricle per beat. Value dependent mainly on the force of contraction of the ventricles and on the amount of blood in the ventricles At rest: • End-diastolic volume = 135 ml • End-systolic volume = 65 ml • Stroke volume = 70 ml Stroke volume may be increased in exercise
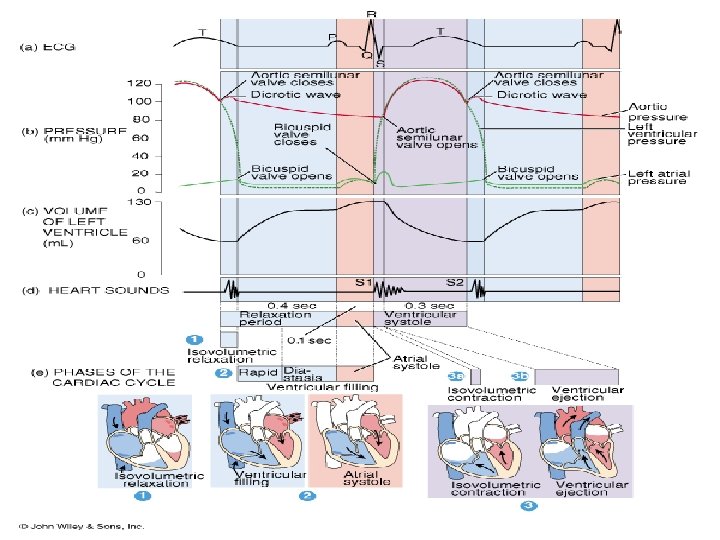
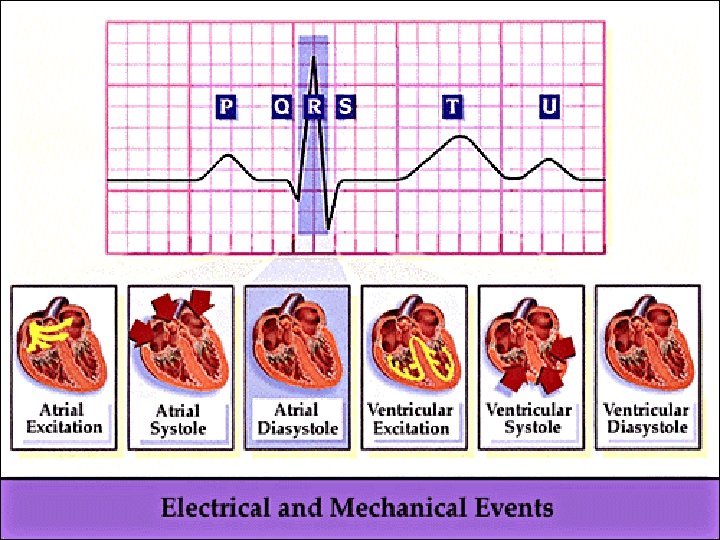
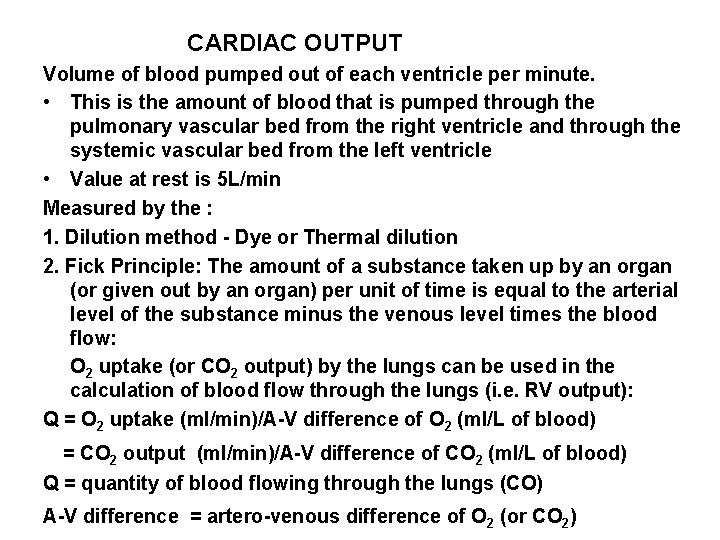
CARDIAC OUTPUT Volume of blood pumped out of each ventricle per minute. • This is the amount of blood that is pumped through the pulmonary vascular bed from the right ventricle and through the systemic vascular bed from the left ventricle • Value at rest is 5 L/min Measured by the : 1. Dilution method - Dye or Thermal dilution 2. Fick Principle: The amount of a substance taken up by an organ (or given out by an organ) per unit of time is equal to the arterial level of the substance minus the venous level times the blood flow: O 2 uptake (or CO 2 output) by the lungs can be used in the calculation of blood flow through the lungs (i. e. RV output): Q = O 2 uptake (ml/min)/A-V difference of O 2 (ml/L of blood) = CO 2 output (ml/min)/A-V difference of CO 2 (ml/L of blood) Q = quantity of blood flowing through the lungs (CO) A-V difference = artero-venous difference of O 2 (or CO 2)
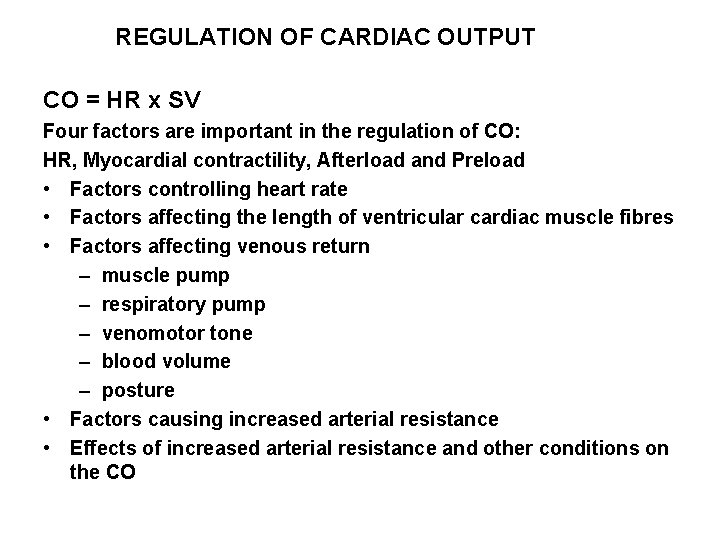
REGULATION OF CARDIAC OUTPUT CO = HR x SV Four factors are important in the regulation of CO: HR, Myocardial contractility, Afterload and Preload • Factors controlling heart rate • Factors affecting the length of ventricular cardiac muscle fibres • Factors affecting venous return – muscle pump – respiratory pump – venomotor tone – blood volume – posture • Factors causing increased arterial resistance • Effects of increased arterial resistance and other conditions on the CO
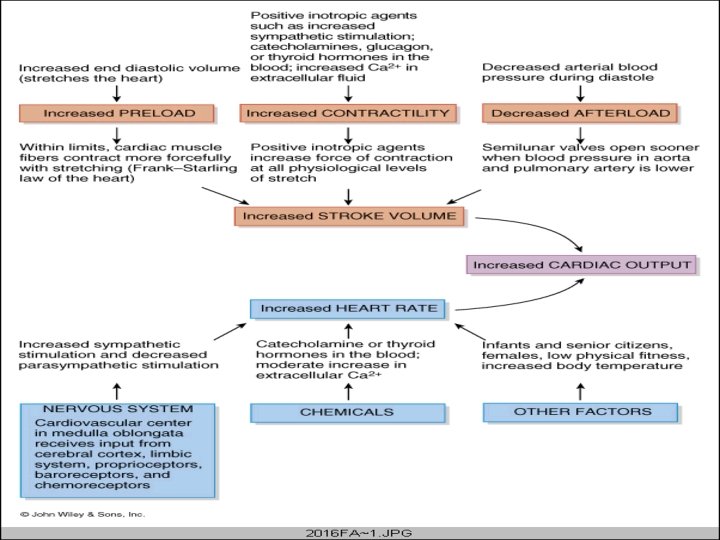
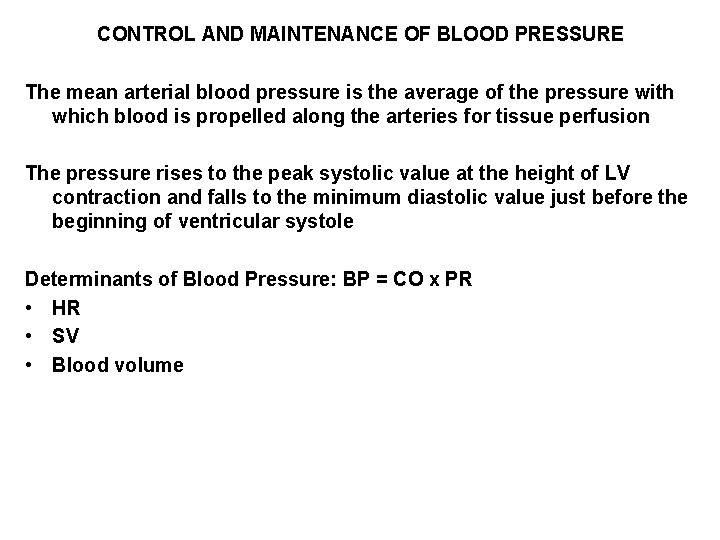
CONTROL AND MAINTENANCE OF BLOOD PRESSURE The mean arterial blood pressure is the average of the pressure with which blood is propelled along the arteries for tissue perfusion The pressure rises to the peak systolic value at the height of LV contraction and falls to the minimum diastolic value just before the beginning of ventricular systole Determinants of Blood Pressure: BP = CO x PR • HR • SV • Blood volume
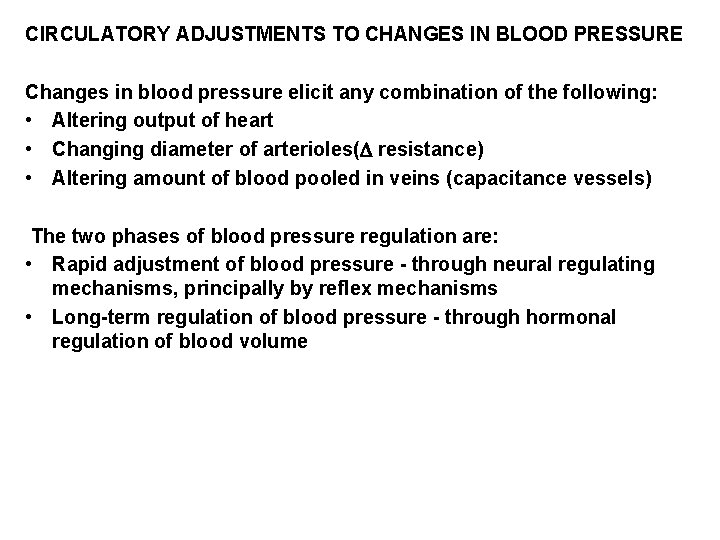
CIRCULATORY ADJUSTMENTS TO CHANGES IN BLOOD PRESSURE Changes in blood pressure elicit any combination of the following: • Altering output of heart • Changing diameter of arterioles( resistance) • Altering amount of blood pooled in veins (capacitance vessels) The two phases of blood pressure regulation are: • Rapid adjustment of blood pressure - through neural regulating mechanisms, principally by reflex mechanisms • Long-term regulation of blood pressure - through hormonal regulation of blood volume
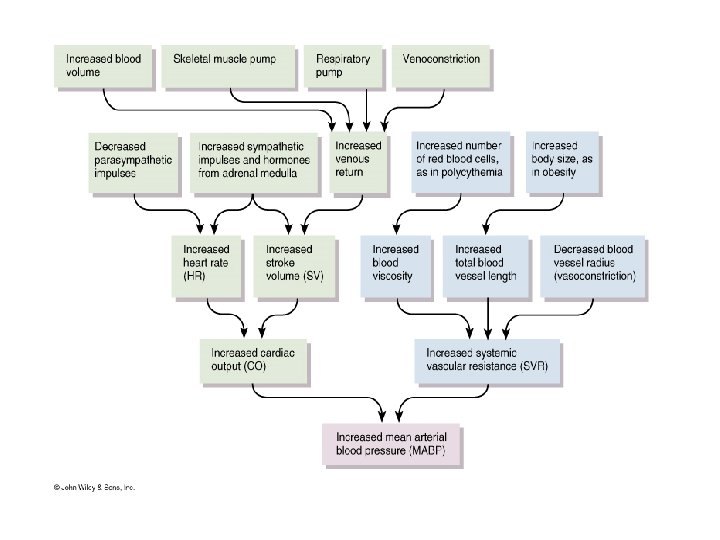
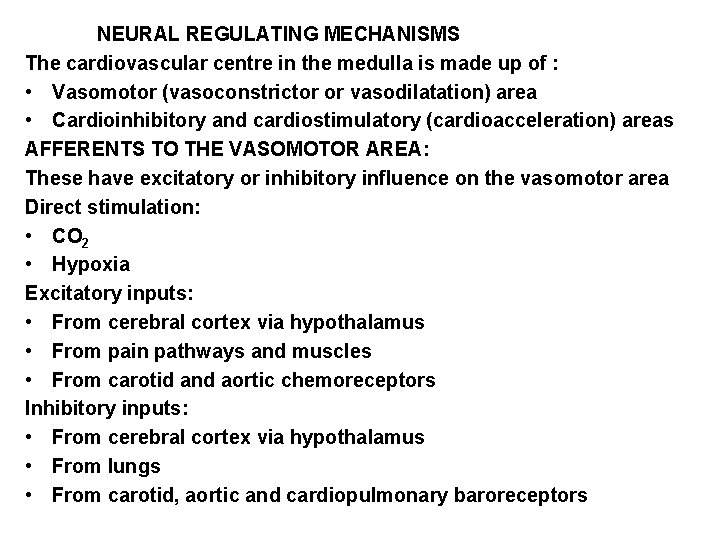
NEURAL REGULATING MECHANISMS The cardiovascular centre in the medulla is made up of : • Vasomotor (vasoconstrictor or vasodilatation) area • Cardioinhibitory and cardiostimulatory (cardioacceleration) areas AFFERENTS TO THE VASOMOTOR AREA: These have excitatory or inhibitory influence on the vasomotor area Direct stimulation: • CO 2 • Hypoxia Excitatory inputs: • From cerebral cortex via hypothalamus • From pain pathways and muscles • From carotid and aortic chemoreceptors Inhibitory inputs: • From cerebral cortex via hypothalamus • From lungs • From carotid, aortic and cardiopulmonary baroreceptors
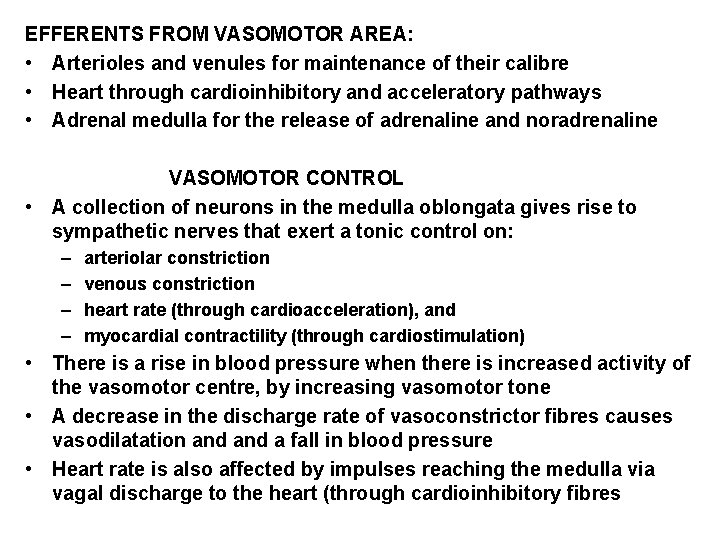
EFFERENTS FROM VASOMOTOR AREA: • Arterioles and venules for maintenance of their calibre • Heart through cardioinhibitory and acceleratory pathways • Adrenal medulla for the release of adrenaline and noradrenaline VASOMOTOR CONTROL • A collection of neurons in the medulla oblongata gives rise to sympathetic nerves that exert a tonic control on: – – arteriolar constriction venous constriction heart rate (through cardioacceleration), and myocardial contractility (through cardiostimulation) • There is a rise in blood pressure when there is increased activity of the vasomotor centre, by increasing vasomotor tone • A decrease in the discharge rate of vasoconstrictor fibres causes vasodilatation and a fall in blood pressure • Heart rate is also affected by impulses reaching the medulla via vagal discharge to the heart (through cardioinhibitory fibres
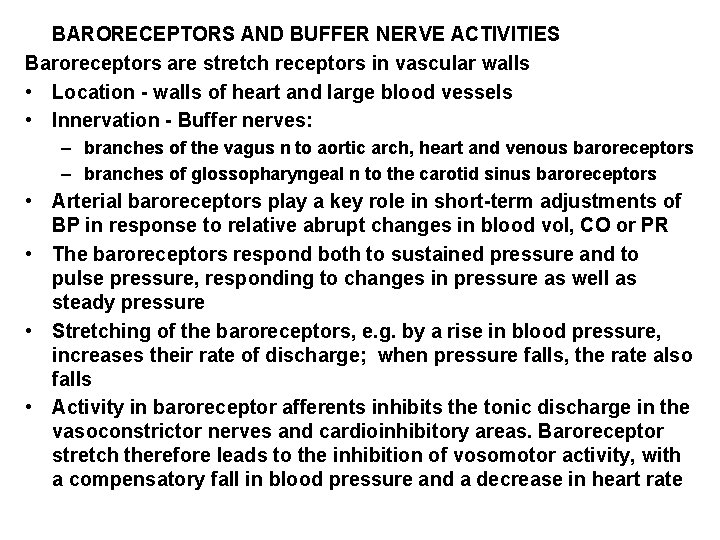
BARORECEPTORS AND BUFFER NERVE ACTIVITIES Baroreceptors are stretch receptors in vascular walls • Location - walls of heart and large blood vessels • Innervation - Buffer nerves: – branches of the vagus n to aortic arch, heart and venous baroreceptors – branches of glossopharyngeal n to the carotid sinus baroreceptors • Arterial baroreceptors play a key role in short-term adjustments of BP in response to relative abrupt changes in blood vol, CO or PR • The baroreceptors respond both to sustained pressure and to pulse pressure, responding to changes in pressure as well as steady pressure • Stretching of the baroreceptors, e. g. by a rise in blood pressure, increases their rate of discharge; when pressure falls, the rate also falls • Activity in baroreceptor afferents inhibits the tonic discharge in the vasoconstrictor nerves and cardioinhibitory areas. Baroreceptor stretch therefore leads to the inhibition of vosomotor activity, with a compensatory fall in blood pressure and a decrease in heart rate
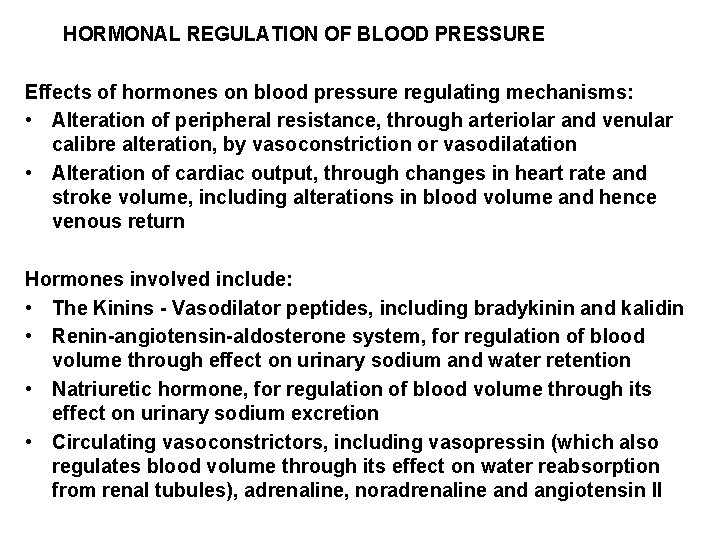
HORMONAL REGULATION OF BLOOD PRESSURE Effects of hormones on blood pressure regulating mechanisms: • Alteration of peripheral resistance, through arteriolar and venular calibre alteration, by vasoconstriction or vasodilatation • Alteration of cardiac output, through changes in heart rate and stroke volume, including alterations in blood volume and hence venous return Hormones involved include: • The Kinins - Vasodilator peptides, including bradykinin and kalidin • Renin-angiotensin-aldosterone system, for regulation of blood volume through effect on urinary sodium and water retention • Natriuretic hormone, for regulation of blood volume through its effect on urinary sodium excretion • Circulating vasoconstrictors, including vasopressin (which also regulates blood volume through its effect on water reabsorption from renal tubules), adrenaline, noradrenaline and angiotensin II
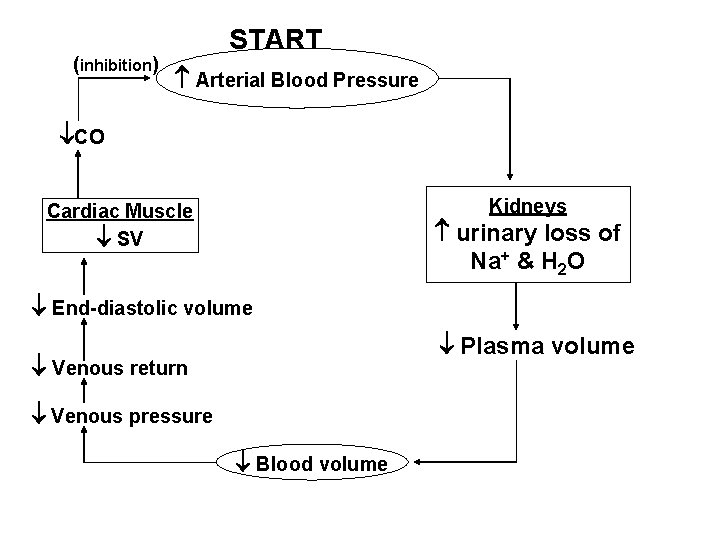
(inhibition) START Arterial Blood Pressure CO Kidneys Cardiac Muscle SV urinary loss of Na+ & H 2 O End-diastolic volume Plasma volume Venous return Venous pressure Blood volume
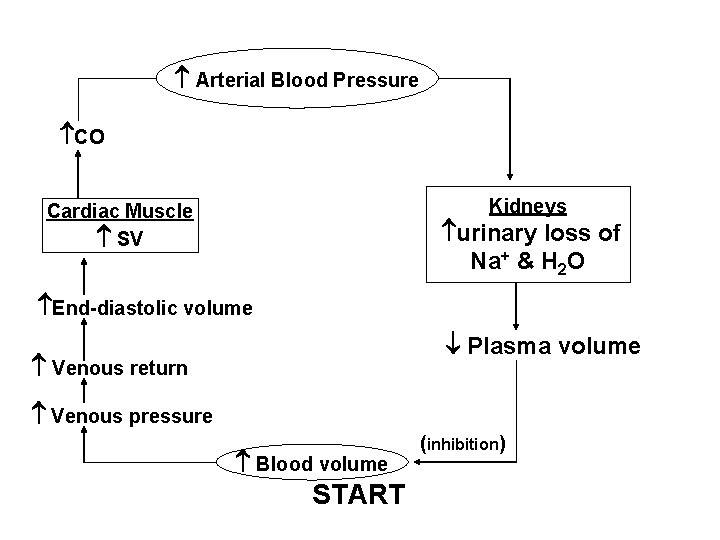
Arterial Blood Pressure CO Kidneys Cardiac Muscle SV urinary loss of Na+ & H 2 O End-diastolic volume Plasma volume Venous return Venous pressure Blood volume START (inhibition)
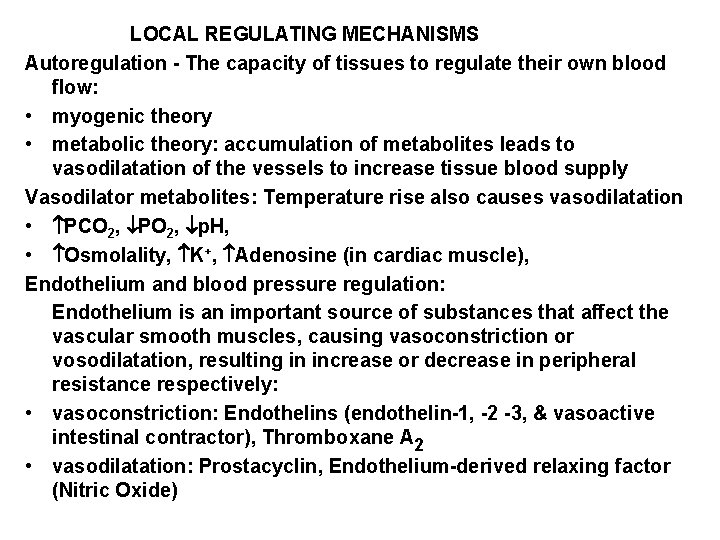
LOCAL REGULATING MECHANISMS Autoregulation - The capacity of tissues to regulate their own blood flow: • myogenic theory • metabolic theory: accumulation of metabolites leads to vasodilatation of the vessels to increase tissue blood supply Vasodilator metabolites: Temperature rise also causes vasodilatation • PCO 2, PO 2, p. H, • Osmolality, K+, Adenosine (in cardiac muscle), Endothelium and blood pressure regulation: Endothelium is an important source of substances that affect the vascular smooth muscles, causing vasoconstriction or vosodilatation, resulting in increase or decrease in peripheral resistance respectively: • vasoconstriction: Endothelins (endothelin-1, -2 -3, & vasoactive intestinal contractor), Thromboxane A 2 • vasodilatation: Prostacyclin, Endothelium-derived relaxing factor (Nitric Oxide)
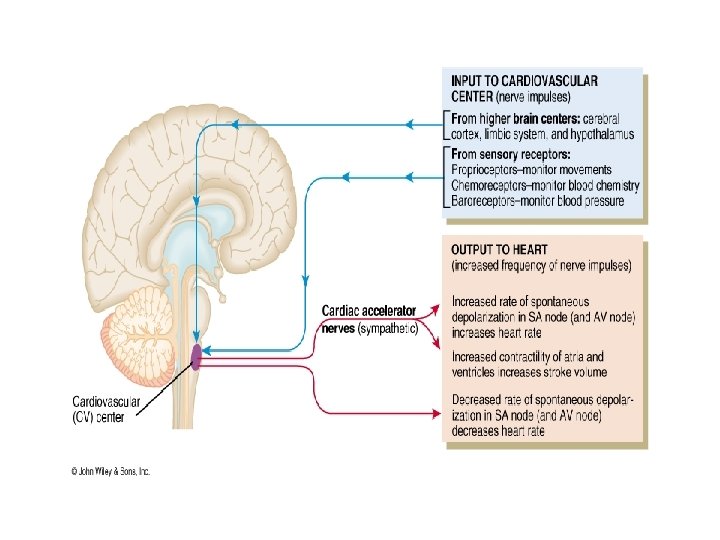
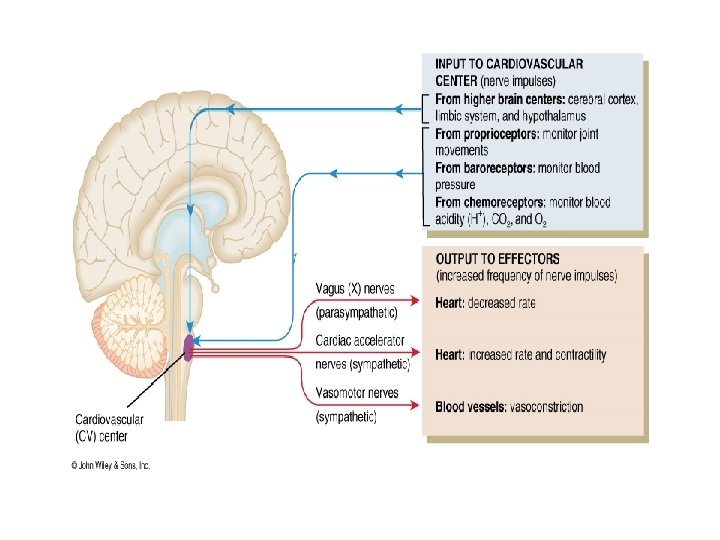
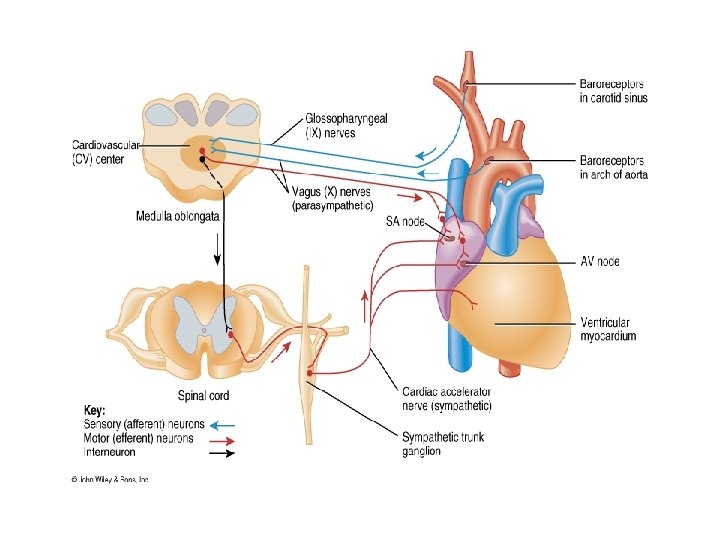
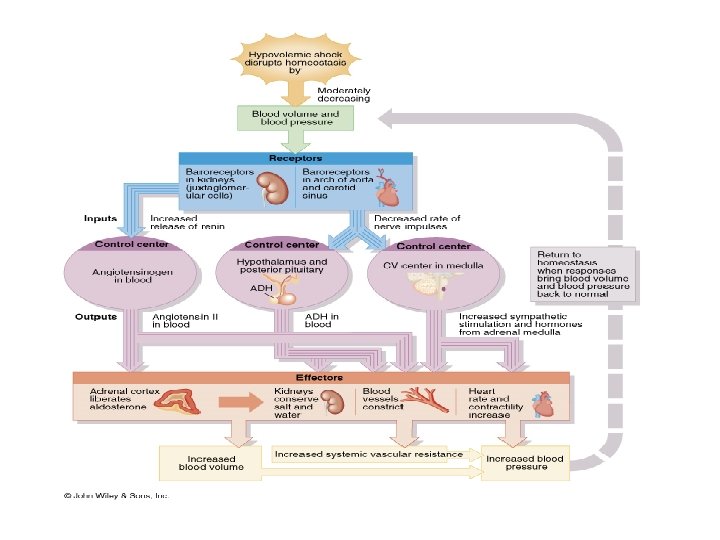
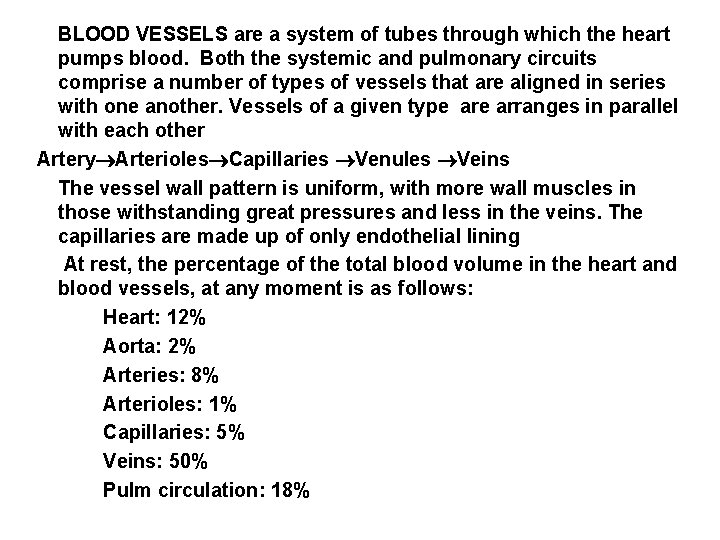
BLOOD VESSELS are a system of tubes through which the heart pumps blood. Both the systemic and pulmonary circuits comprise a number of types of vessels that are aligned in series with one another. Vessels of a given type arranges in parallel with each other Artery Arterioles Capillaries Venules Veins The vessel wall pattern is uniform, with more wall muscles in those withstanding great pressures and less in the veins. The capillaries are made up of only endothelial lining At rest, the percentage of the total blood volume in the heart and blood vessels, at any moment is as follows: Heart: 12% Aorta: 2% Arteries: 8% Arterioles: 1% Capillaries: 5% Veins: 50% Pulm circulation: 18%
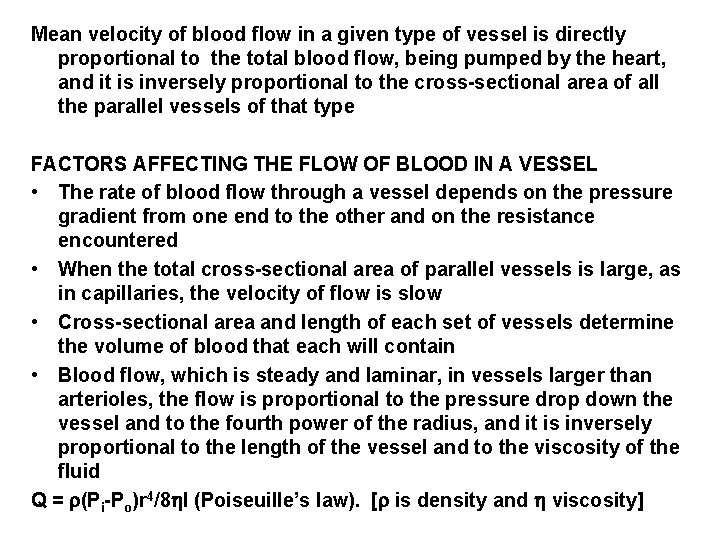
Mean velocity of blood flow in a given type of vessel is directly proportional to the total blood flow, being pumped by the heart, and it is inversely proportional to the cross-sectional area of all the parallel vessels of that type FACTORS AFFECTING THE FLOW OF BLOOD IN A VESSEL • The rate of blood flow through a vessel depends on the pressure gradient from one end to the other and on the resistance encountered • When the total cross-sectional area of parallel vessels is large, as in capillaries, the velocity of flow is slow • Cross-sectional area and length of each set of vessels determine the volume of blood that each will contain • Blood flow, which is steady and laminar, in vessels larger than arterioles, the flow is proportional to the pressure drop down the vessel and to the fourth power of the radius, and it is inversely proportional to the length of the vessel and to the viscosity of the fluid Q = (Pi-Po)r 4/8 l (Poiseuille’s law). [ is density and viscosity]
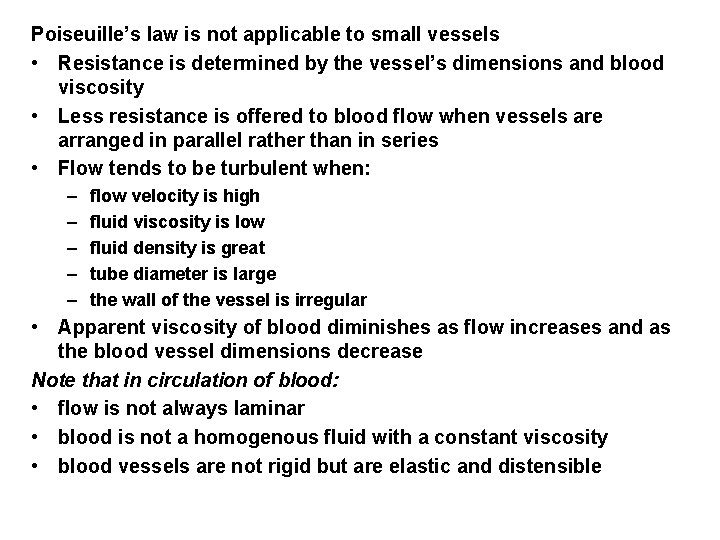
Poiseuille’s law is not applicable to small vessels • Resistance is determined by the vessel’s dimensions and blood viscosity • Less resistance is offered to blood flow when vessels are arranged in parallel rather than in series • Flow tends to be turbulent when: – – – flow velocity is high fluid viscosity is low fluid density is great tube diameter is large the wall of the vessel is irregular • Apparent viscosity of blood diminishes as flow increases and as the blood vessel dimensions decrease Note that in circulation of blood: • flow is not always laminar • blood is not a homogenous fluid with a constant viscosity • blood vessels are not rigid but are elastic and distensible
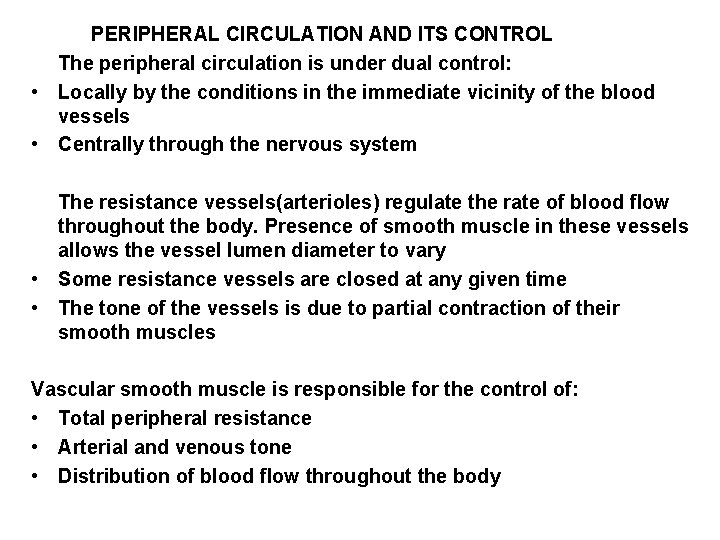
PERIPHERAL CIRCULATION AND ITS CONTROL The peripheral circulation is under dual control: • Locally by the conditions in the immediate vicinity of the blood vessels • Centrally through the nervous system The resistance vessels(arterioles) regulate the rate of blood flow throughout the body. Presence of smooth muscle in these vessels allows the vessel lumen diameter to vary • Some resistance vessels are closed at any given time • The tone of the vessels is due to partial contraction of their smooth muscles Vascular smooth muscle is responsible for the control of: • Total peripheral resistance • Arterial and venous tone • Distribution of blood flow throughout the body
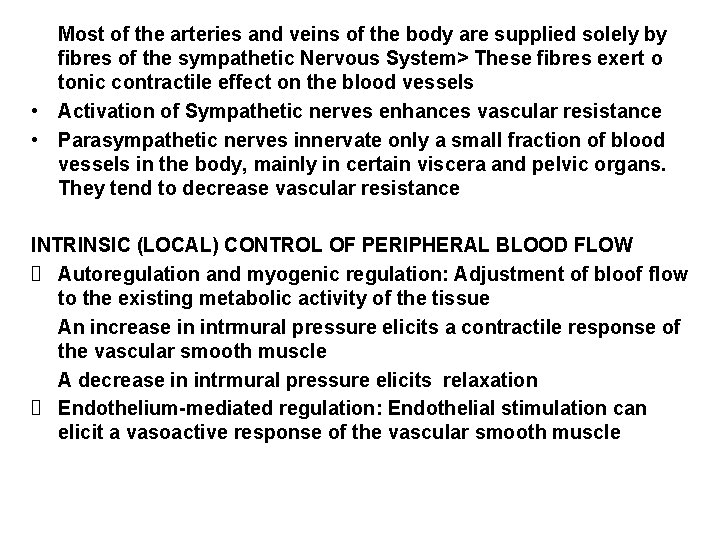
Most of the arteries and veins of the body are supplied solely by fibres of the sympathetic Nervous System> These fibres exert o tonic contractile effect on the blood vessels • Activation of Sympathetic nerves enhances vascular resistance • Parasympathetic nerves innervate only a small fraction of blood vessels in the body, mainly in certain viscera and pelvic organs. They tend to decrease vascular resistance INTRINSIC (LOCAL) CONTROL OF PERIPHERAL BLOOD FLOW Autoregulation and myogenic regulation: Adjustment of bloof flow to the existing metabolic activity of the tissue An increase in intrmural pressure elicits a contractile response of the vascular smooth muscle A decrease in intrmural pressure elicits relaxation Endothelium-mediated regulation: Endothelial stimulation can elicit a vasoactive response of the vascular smooth muscle
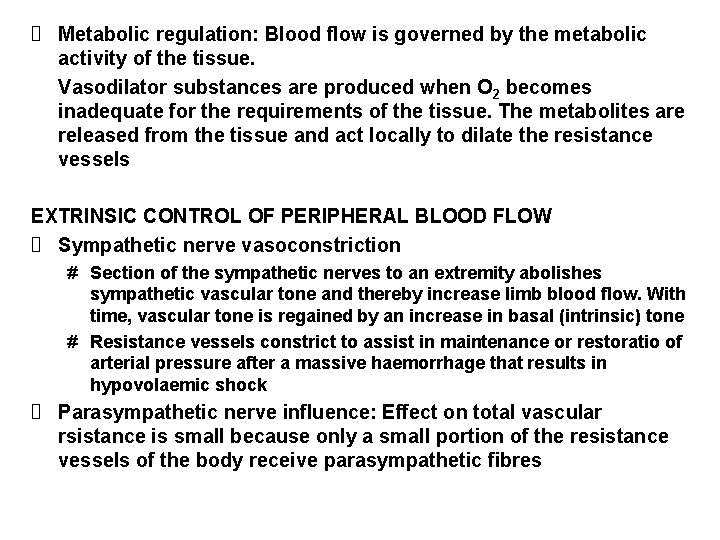
Metabolic regulation: Blood flow is governed by the metabolic activity of the tissue. Vasodilator substances are produced when O 2 becomes inadequate for the requirements of the tissue. The metabolites are released from the tissue and act locally to dilate the resistance vessels EXTRINSIC CONTROL OF PERIPHERAL BLOOD FLOW Sympathetic nerve vasoconstriction # Section of the sympathetic nerves to an extremity abolishes sympathetic vascular tone and thereby increase limb blood flow. With time, vascular tone is regained by an increase in basal (intrinsic) tone # Resistance vessels constrict to assist in maintenance or restoratio of arterial pressure after a massive haemorrhage that results in hypovolaemic shock Parasympathetic nerve influence: Effect on total vascular rsistance is small because only a small portion of the resistance vessels of the body receive parasympathetic fibres
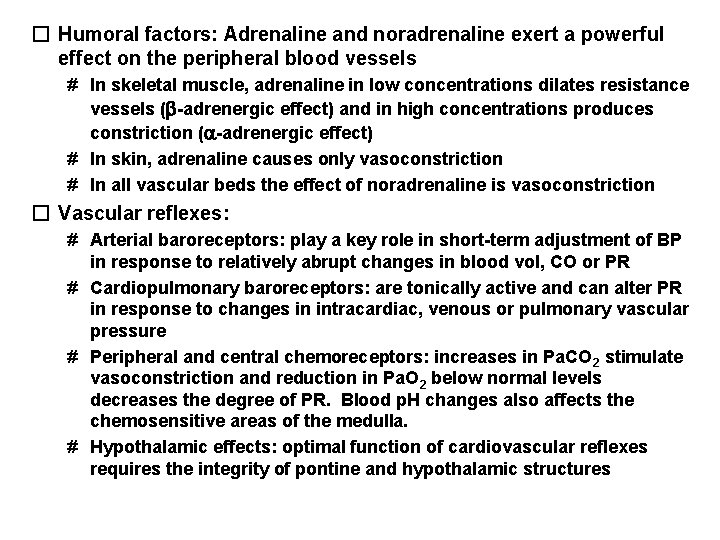
� Humoral factors: Adrenaline and noradrenaline exert a powerful effect on the peripheral blood vessels # In skeletal muscle, adrenaline in low concentrations dilates resistance vessels ( -adrenergic effect) and in high concentrations produces constriction ( -adrenergic effect) # In skin, adrenaline causes only vasoconstriction # In all vascular beds the effect of noradrenaline is vasoconstriction � Vascular reflexes: # Arterial baroreceptors: play a key role in short-term adjustment of BP in response to relatively abrupt changes in blood vol, CO or PR # Cardiopulmonary baroreceptors: are tonically active and can alter PR in response to changes in intracardiac, venous or pulmonary vascular pressure # Peripheral and central chemoreceptors: increases in Pa. CO 2 stimulate vasoconstriction and reduction in Pa. O 2 below normal levels decreases the degree of PR. Blood p. H changes also affects the chemosensitive areas of the medulla. # Hypothalamic effects: optimal function of cardiovascular reflexes requires the integrity of pontine and hypothalamic structures
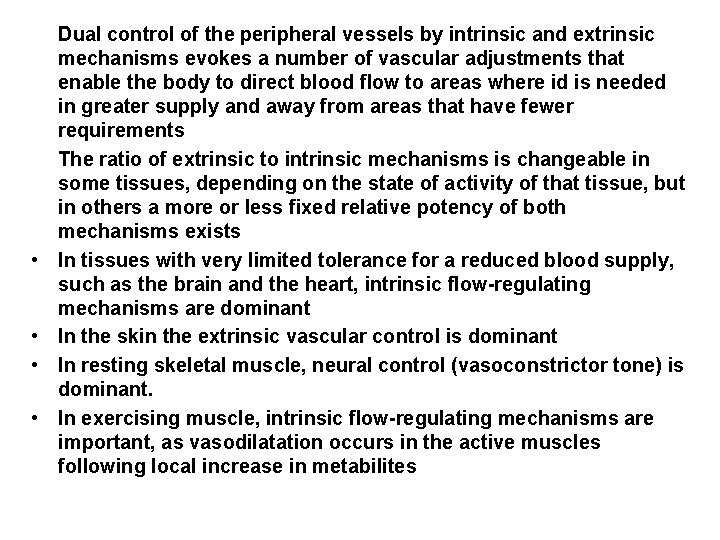
• • Dual control of the peripheral vessels by intrinsic and extrinsic mechanisms evokes a number of vascular adjustments that enable the body to direct blood flow to areas where id is needed in greater supply and away from areas that have fewer requirements The ratio of extrinsic to intrinsic mechanisms is changeable in some tissues, depending on the state of activity of that tissue, but in others a more or less fixed relative potency of both mechanisms exists In tissues with very limited tolerance for a reduced blood supply, such as the brain and the heart, intrinsic flow-regulating mechanisms are dominant In the skin the extrinsic vascular control is dominant In resting skeletal muscle, neural control (vasoconstrictor tone) is dominant. In exercising muscle, intrinsic flow-regulating mechanisms are important, as vasodilatation occurs in the active muscles following local increase in metabilites
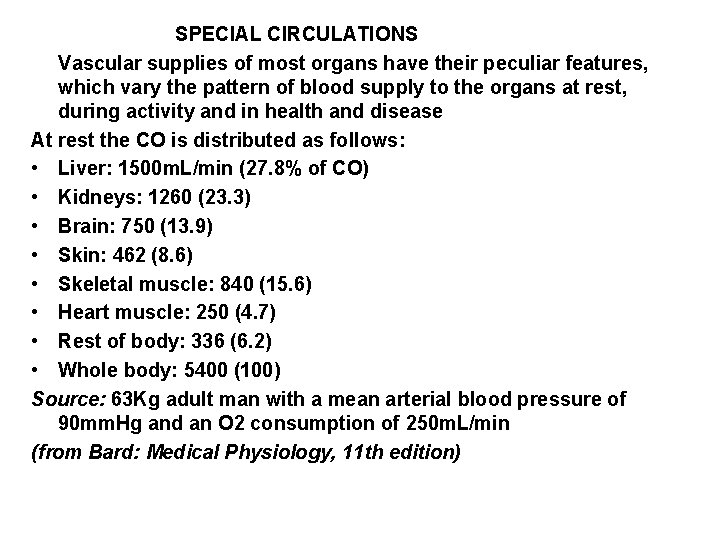
SPECIAL CIRCULATIONS Vascular supplies of most organs have their peculiar features, which vary the pattern of blood supply to the organs at rest, during activity and in health and disease At rest the CO is distributed as follows: • Liver: 1500 m. L/min (27. 8% of CO) • Kidneys: 1260 (23. 3) • Brain: 750 (13. 9) • Skin: 462 (8. 6) • Skeletal muscle: 840 (15. 6) • Heart muscle: 250 (4. 7) • Rest of body: 336 (6. 2) • Whole body: 5400 (100) Source: 63 Kg adult man with a mean arterial blood pressure of 90 mm. Hg and an O 2 consumption of 250 m. L/min (from Bard: Medical Physiology, 11 th edition)
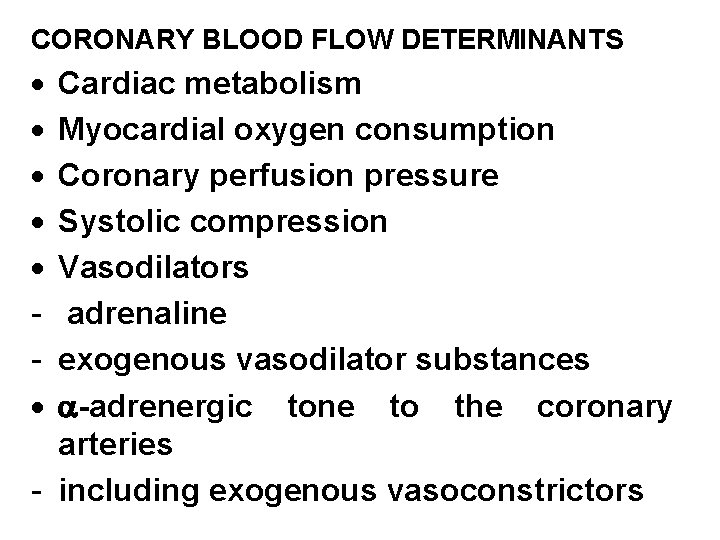
CORONARY BLOOD FLOW DETERMINANTS · · · Cardiac metabolism Myocardial oxygen consumption Coronary perfusion pressure Systolic compression Vasodilators adrenaline exogenous vasodilator substances -adrenergic tone to the coronary arteries - including exogenous vasoconstrictors
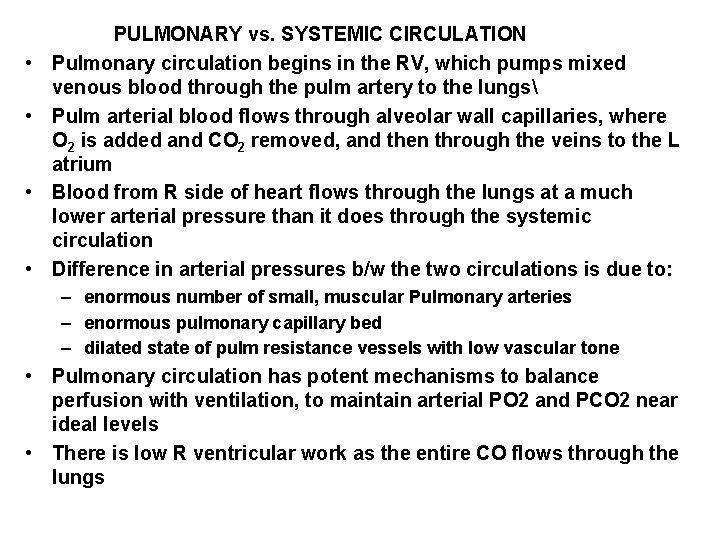
• • PULMONARY vs. SYSTEMIC CIRCULATION Pulmonary circulation begins in the RV, which pumps mixed venous blood through the pulm artery to the lungs Pulm arterial blood flows through alveolar wall capillaries, where O 2 is added and CO 2 removed, and then through the veins to the L atrium Blood from R side of heart flows through the lungs at a much lower arterial pressure than it does through the systemic circulation Difference in arterial pressures b/w the two circulations is due to: – enormous number of small, muscular Pulmonary arteries – enormous pulmonary capillary bed – dilated state of pulm resistance vessels with low vascular tone • Pulmonary circulation has potent mechanisms to balance perfusion with ventilation, to maintain arterial PO 2 and PCO 2 near ideal levels • There is low R ventricular work as the entire CO flows through the lungs
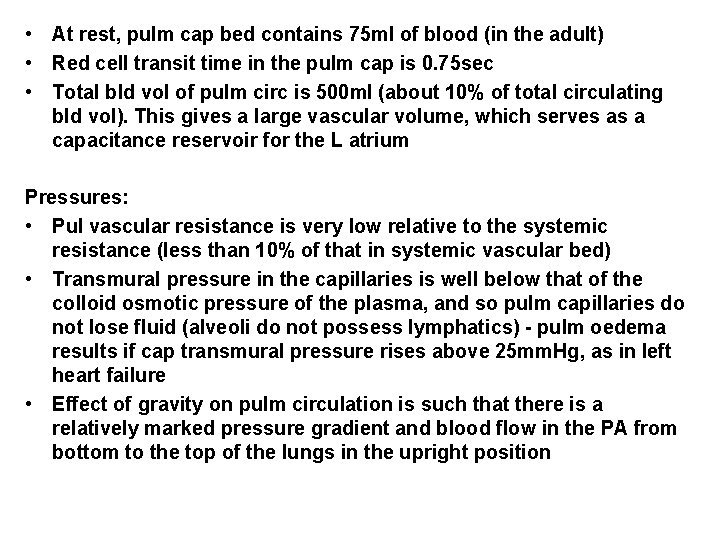
• At rest, pulm cap bed contains 75 ml of blood (in the adult) • Red cell transit time in the pulm cap is 0. 75 sec • Total bld vol of pulm circ is 500 ml (about 10% of total circulating bld vol). This gives a large vascular volume, which serves as a capacitance reservoir for the L atrium Pressures: • Pul vascular resistance is very low relative to the systemic resistance (less than 10% of that in systemic vascular bed) • Transmural pressure in the capillaries is well below that of the colloid osmotic pressure of the plasma, and so pulm capillaries do not lose fluid (alveoli do not possess lymphatics) - pulm oedema results if cap transmural pressure rises above 25 mm. Hg, as in left heart failure • Effect of gravity on pulm circulation is such that there is a relatively marked pressure gradient and blood flow in the PA from bottom to the top of the lungs in the upright position
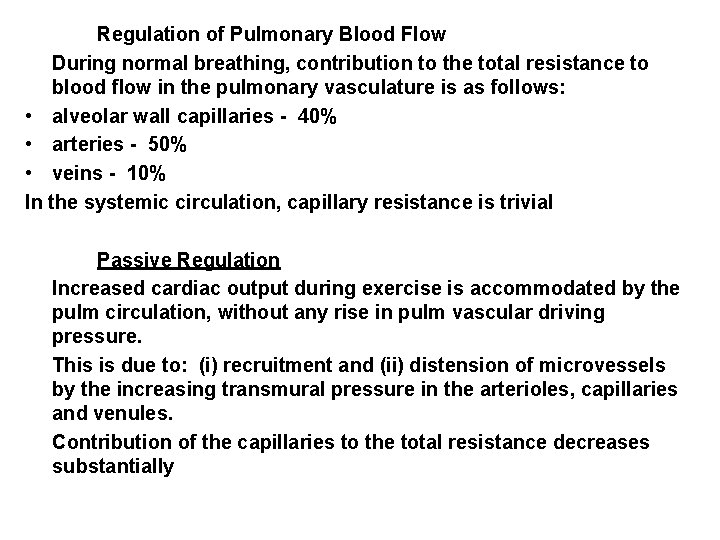
Regulation of Pulmonary Blood Flow During normal breathing, contribution to the total resistance to blood flow in the pulmonary vasculature is as follows: • alveolar wall capillaries - 40% • arteries - 50% • veins - 10% In the systemic circulation, capillary resistance is trivial Passive Regulation Increased cardiac output during exercise is accommodated by the pulm circulation, without any rise in pulm vascular driving pressure. This is due to: (i) recruitment and (ii) distension of microvessels by the increasing transmural pressure in the arterioles, capillaries and venules. Contribution of the capillaries to the total resistance decreases substantially
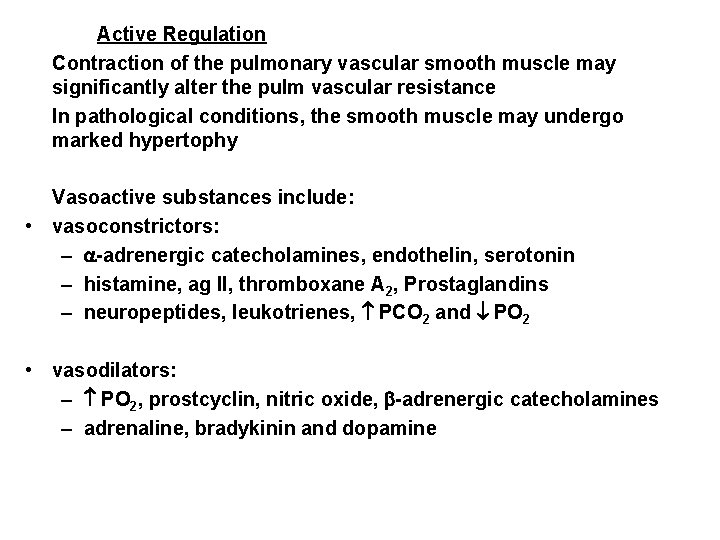
Active Regulation Contraction of the pulmonary vascular smooth muscle may significantly alter the pulm vascular resistance In pathological conditions, the smooth muscle may undergo marked hypertophy Vasoactive substances include: • vasoconstrictors: – -adrenergic catecholamines, endothelin, serotonin – histamine, ag II, thromboxane A 2, Prostaglandins – neuropeptides, leukotrienes, PCO 2 and PO 2 • vasodilators: – PO 2, prostcyclin, nitric oxide, -adrenergic catecholamines – adrenaline, bradykinin and dopamine
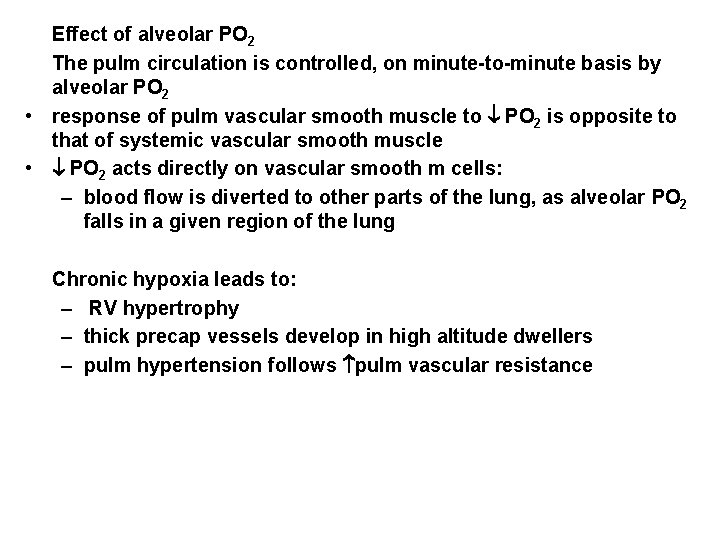
Effect of alveolar PO 2 The pulm circulation is controlled, on minute-to-minute basis by alveolar PO 2 • response of pulm vascular smooth muscle to PO 2 is opposite to that of systemic vascular smooth muscle • PO 2 acts directly on vascular smooth m cells: – blood flow is diverted to other parts of the lung, as alveolar PO 2 falls in a given region of the lung Chronic hypoxia leads to: – RV hypertrophy – thick precap vessels develop in high altitude dwellers – pulm hypertension follows pulm vascular resistance
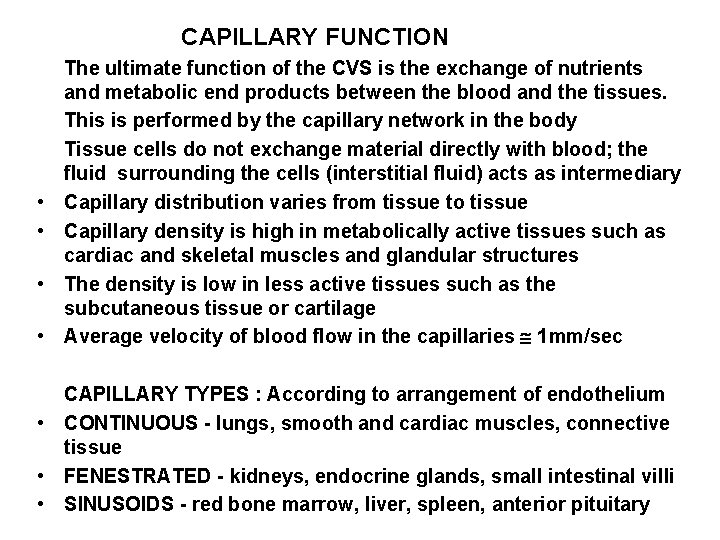
CAPILLARY FUNCTION • • The ultimate function of the CVS is the exchange of nutrients and metabolic end products between the blood and the tissues. This is performed by the capillary network in the body Tissue cells do not exchange material directly with blood; the fluid surrounding the cells (interstitial fluid) acts as intermediary Capillary distribution varies from tissue to tissue Capillary density is high in metabolically active tissues such as cardiac and skeletal muscles and glandular structures The density is low in less active tissues such as the subcutaneous tissue or cartilage Average velocity of blood flow in the capillaries 1 mm/sec CAPILLARY TYPES : According to arrangement of endothelium • CONTINUOUS - lungs, smooth and cardiac muscles, connective tissue • FENESTRATED - kidneys, endocrine glands, small intestinal villi • SINUSOIDS - red bone marrow, liver, spleen, anterior pituitary
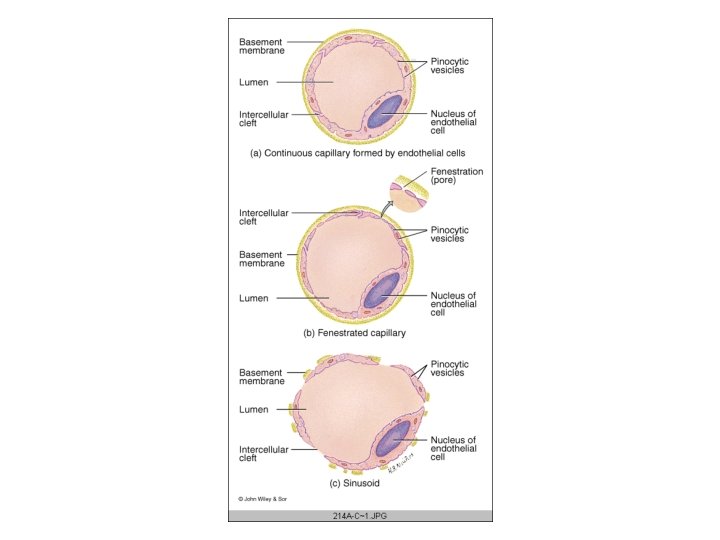
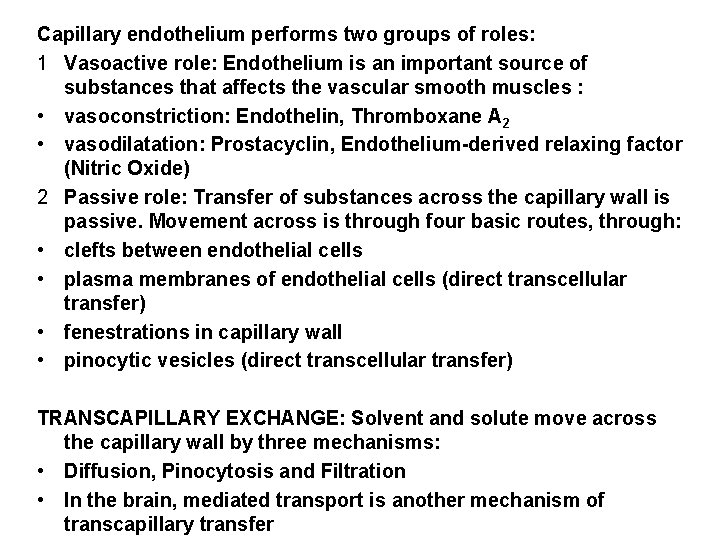
Capillary endothelium performs two groups of roles: 1 Vasoactive role: Endothelium is an important source of substances that affects the vascular smooth muscles : • vasoconstriction: Endothelin, Thromboxane A 2 • vasodilatation: Prostacyclin, Endothelium-derived relaxing factor (Nitric Oxide) 2 Passive role: Transfer of substances across the capillary wall is passive. Movement across is through four basic routes, through: • clefts between endothelial cells • plasma membranes of endothelial cells (direct transcellular transfer) • fenestrations in capillary wall • pinocytic vesicles (direct transcellular transfer) TRANSCAPILLARY EXCHANGE: Solvent and solute move across the capillary wall by three mechanisms: • Diffusion, Pinocytosis and Filtration • In the brain, mediated transport is another mechanism of transcapillary transfer
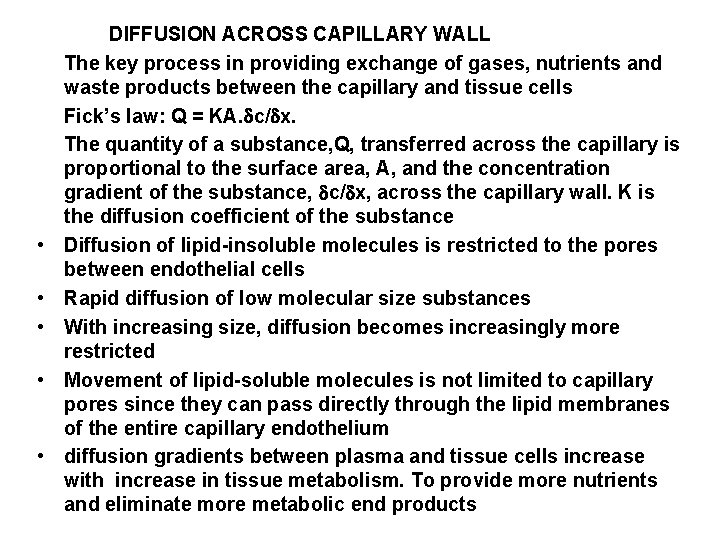
• • • DIFFUSION ACROSS CAPILLARY WALL The key process in providing exchange of gases, nutrients and waste products between the capillary and tissue cells Fick’s law: Q = KA. c/ x. The quantity of a substance, Q, transferred across the capillary is proportional to the surface area, A, and the concentration gradient of the substance, c/ x, across the capillary wall. K is the diffusion coefficient of the substance Diffusion of lipid-insoluble molecules is restricted to the pores between endothelial cells Rapid diffusion of low molecular size substances With increasing size, diffusion becomes increasingly more restricted Movement of lipid-soluble molecules is not limited to capillary pores since they can pass directly through the lipid membranes of the entire capillary endothelium diffusion gradients between plasma and tissue cells increase with increase in tissue metabolism. To provide more nutrients and eliminate more metabolic end products
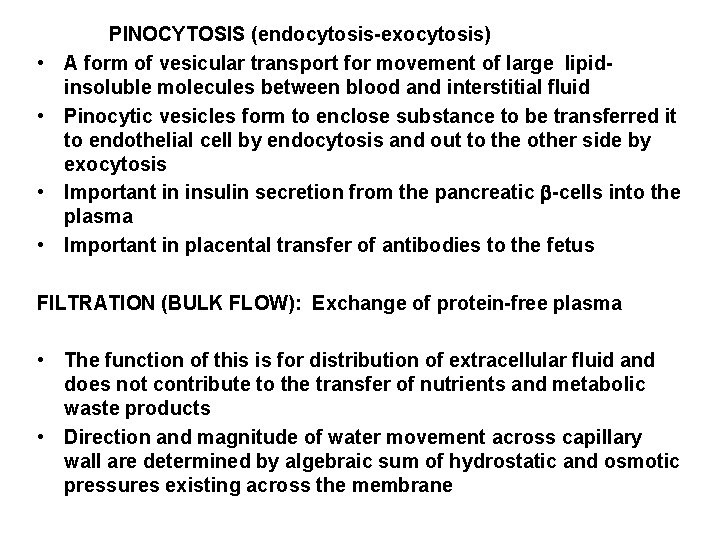
• • PINOCYTOSIS (endocytosis-exocytosis) A form of vesicular transport for movement of large lipidinsoluble molecules between blood and interstitial fluid Pinocytic vesicles form to enclose substance to be transferred it to endothelial cell by endocytosis and out to the other side by exocytosis Important in insulin secretion from the pancreatic -cells into the plasma Important in placental transfer of antibodies to the fetus FILTRATION (BULK FLOW): Exchange of protein-free plasma • The function of this is for distribution of extracellular fluid and does not contribute to the transfer of nutrients and metabolic waste products • Direction and magnitude of water movement across capillary wall are determined by algebraic sum of hydrostatic and osmotic pressures existing across the membrane
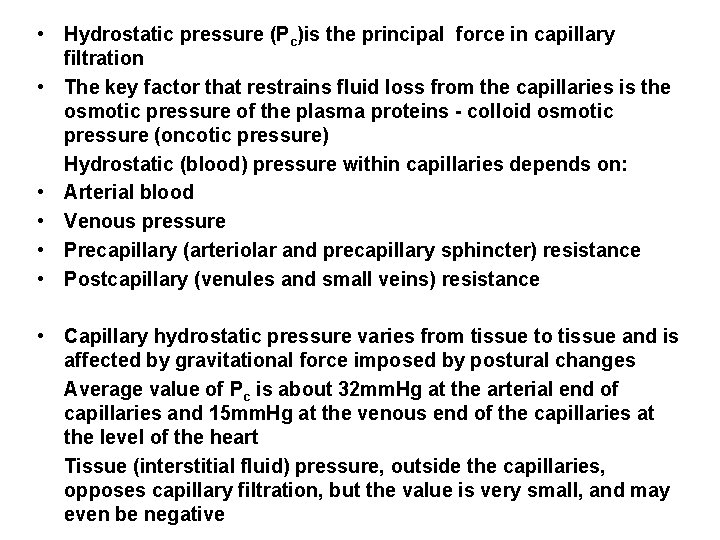
• Hydrostatic pressure (Pc)is the principal force in capillary filtration • The key factor that restrains fluid loss from the capillaries is the osmotic pressure of the plasma proteins - colloid osmotic pressure (oncotic pressure) Hydrostatic (blood) pressure within capillaries depends on: • Arterial blood • Venous pressure • Precapillary (arteriolar and precapillary sphincter) resistance • Postcapillary (venules and small veins) resistance • Capillary hydrostatic pressure varies from tissue to tissue and is affected by gravitational force imposed by postural changes Average value of Pc is about 32 mm. Hg at the arterial end of capillaries and 15 mm. Hg at the venous end of the capillaries at the level of the heart Tissue (interstitial fluid) pressure, outside the capillaries, opposes capillary filtration, but the value is very small, and may even be negative
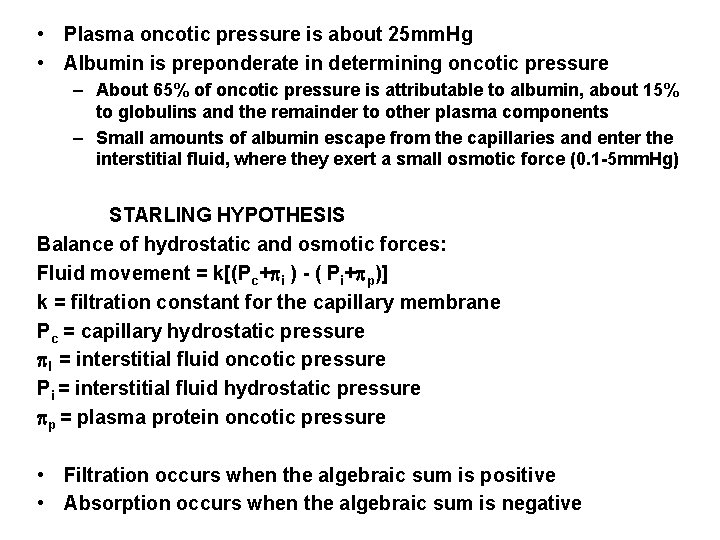
• Plasma oncotic pressure is about 25 mm. Hg • Albumin is preponderate in determining oncotic pressure – About 65% of oncotic pressure is attributable to albumin, about 15% to globulins and the remainder to other plasma components – Small amounts of albumin escape from the capillaries and enter the interstitial fluid, where they exert a small osmotic force (0. 1 -5 mm. Hg) STARLING HYPOTHESIS Balance of hydrostatic and osmotic forces: Fluid movement = k[(Pc+ i ) - ( Pi+ p)] k = filtration constant for the capillary membrane Pc = capillary hydrostatic pressure I = interstitial fluid oncotic pressure Pi = interstitial fluid hydrostatic pressure p = plasma protein oncotic pressure • Filtration occurs when the algebraic sum is positive • Absorption occurs when the algebraic sum is negative
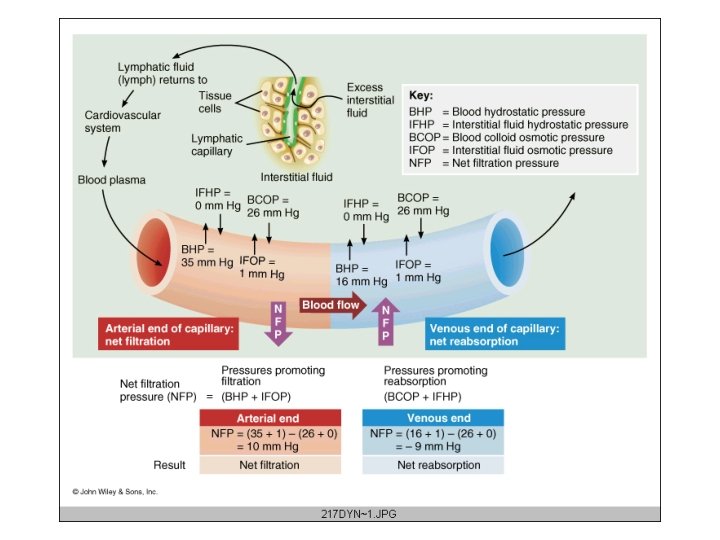
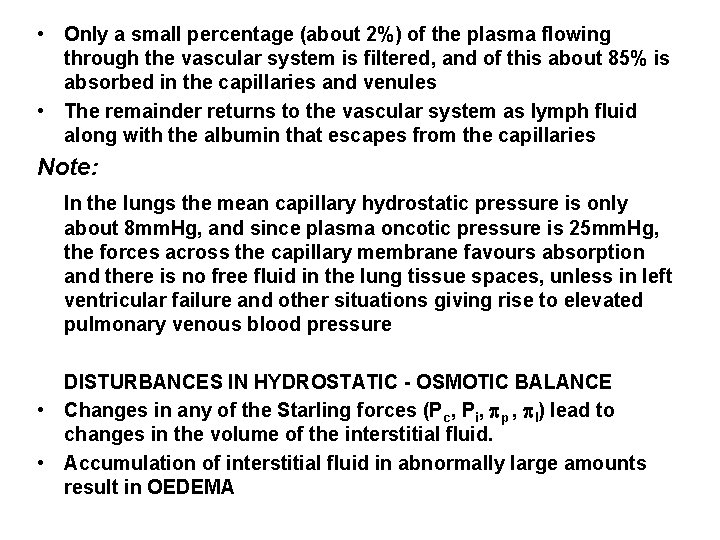
• Only a small percentage (about 2%) of the plasma flowing through the vascular system is filtered, and of this about 85% is absorbed in the capillaries and venules • The remainder returns to the vascular system as lymph fluid along with the albumin that escapes from the capillaries Note: In the lungs the mean capillary hydrostatic pressure is only about 8 mm. Hg, and since plasma oncotic pressure is 25 mm. Hg, the forces across the capillary membrane favours absorption and there is no free fluid in the lung tissue spaces, unless in left ventricular failure and other situations giving rise to elevated pulmonary venous blood pressure DISTURBANCES IN HYDROSTATIC - OSMOTIC BALANCE • Changes in any of the Starling forces (Pc, Pi, p , I) lead to changes in the volume of the interstitial fluid. • Accumulation of interstitial fluid in abnormally large amounts result in OEDEMA
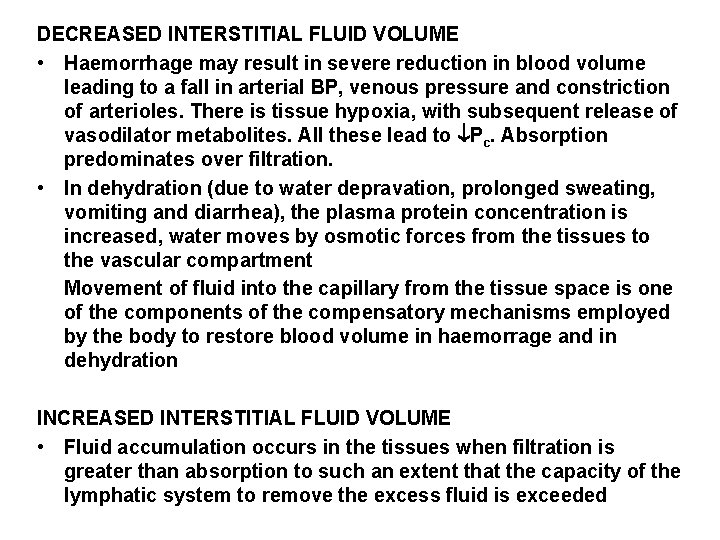
DECREASED INTERSTITIAL FLUID VOLUME • Haemorrhage may result in severe reduction in blood volume leading to a fall in arterial BP, venous pressure and constriction of arterioles. There is tissue hypoxia, with subsequent release of vasodilator metabolites. All these lead to Pc. Absorption predominates over filtration. • In dehydration (due to water depravation, prolonged sweating, vomiting and diarrhea), the plasma protein concentration is increased, water moves by osmotic forces from the tissues to the vascular compartment Movement of fluid into the capillary from the tissue space is one of the components of the compensatory mechanisms employed by the body to restore blood volume in haemorrage and in dehydration INCREASED INTERSTITIAL FLUID VOLUME • Fluid accumulation occurs in the tissues when filtration is greater than absorption to such an extent that the capacity of the lymphatic system to remove the excess fluid is exceeded
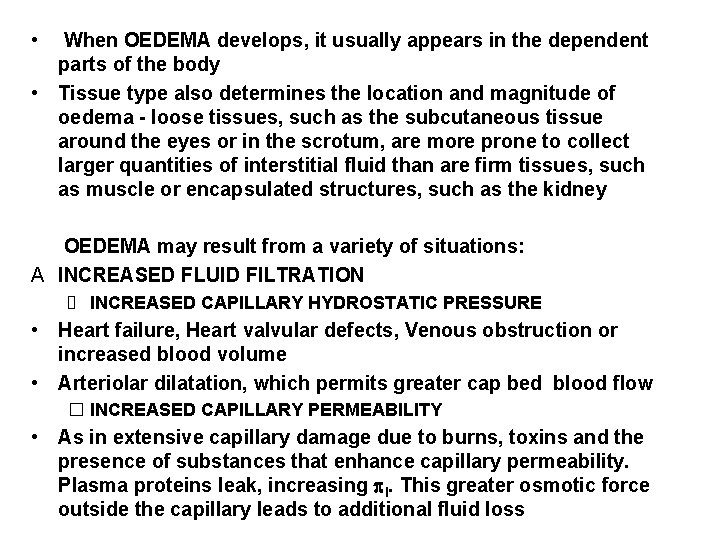
• When OEDEMA develops, it usually appears in the dependent parts of the body • Tissue type also determines the location and magnitude of oedema - loose tissues, such as the subcutaneous tissue around the eyes or in the scrotum, are more prone to collect larger quantities of interstitial fluid than are firm tissues, such as muscle or encapsulated structures, such as the kidney OEDEMA may result from a variety of situations: A INCREASED FLUID FILTRATION INCREASED CAPILLARY HYDROSTATIC PRESSURE • Heart failure, Heart valvular defects, Venous obstruction or increased blood volume • Arteriolar dilatation, which permits greater cap bed blood flow � INCREASED CAPILLARY PERMEABILITY • As in extensive capillary damage due to burns, toxins and the presence of substances that enhance capillary permeability. Plasma proteins leak, increasing I. This greater osmotic force outside the capillary leads to additional fluid loss
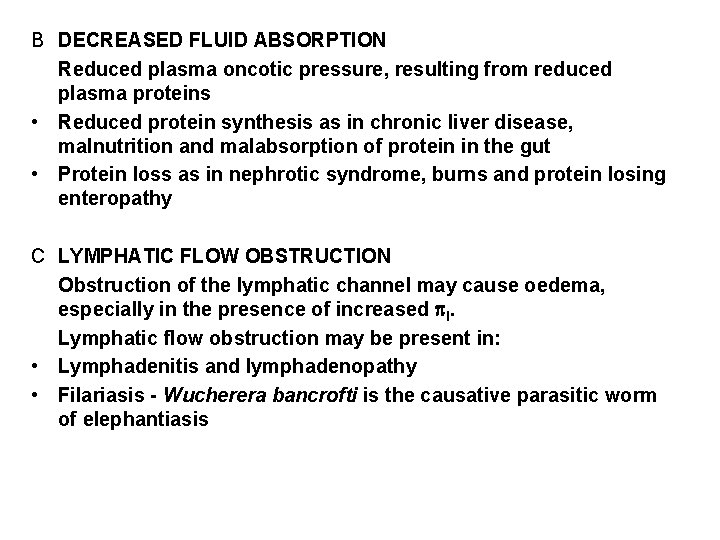
B DECREASED FLUID ABSORPTION Reduced plasma oncotic pressure, resulting from reduced plasma proteins • Reduced protein synthesis as in chronic liver disease, malnutrition and malabsorption of protein in the gut • Protein loss as in nephrotic syndrome, burns and protein losing enteropathy C LYMPHATIC FLOW OBSTRUCTION Obstruction of the lymphatic channel may cause oedema, especially in the presence of increased I. Lymphatic flow obstruction may be present in: • Lymphadenitis and lymphadenopathy • Filariasis - Wucherera bancrofti is the causative parasitic worm of elephantiasis
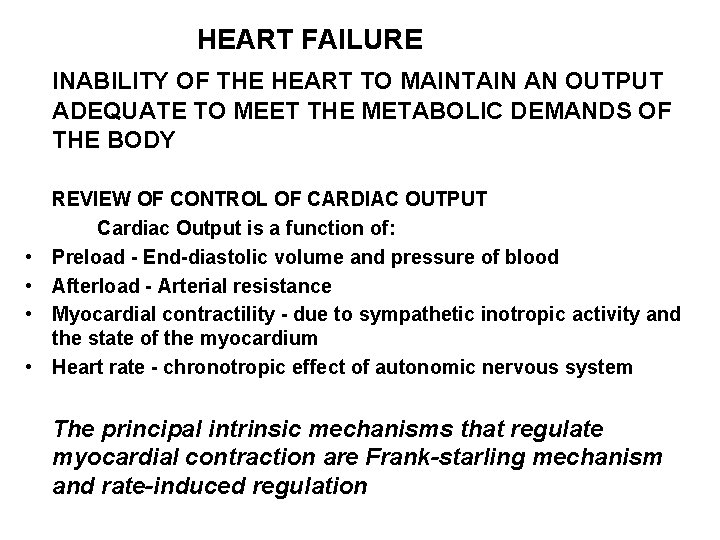
HEART FAILURE INABILITY OF THE HEART TO MAINTAIN AN OUTPUT ADEQUATE TO MEET THE METABOLIC DEMANDS OF THE BODY • • REVIEW OF CONTROL OF CARDIAC OUTPUT Cardiac Output is a function of: Preload - End-diastolic volume and pressure of blood Afterload - Arterial resistance Myocardial contractility - due to sympathetic inotropic activity and the state of the myocardium Heart rate - chronotropic effect of autonomic nervous system The principal intrinsic mechanisms that regulate myocardial contraction are Frank-starling mechanism and rate-induced regulation
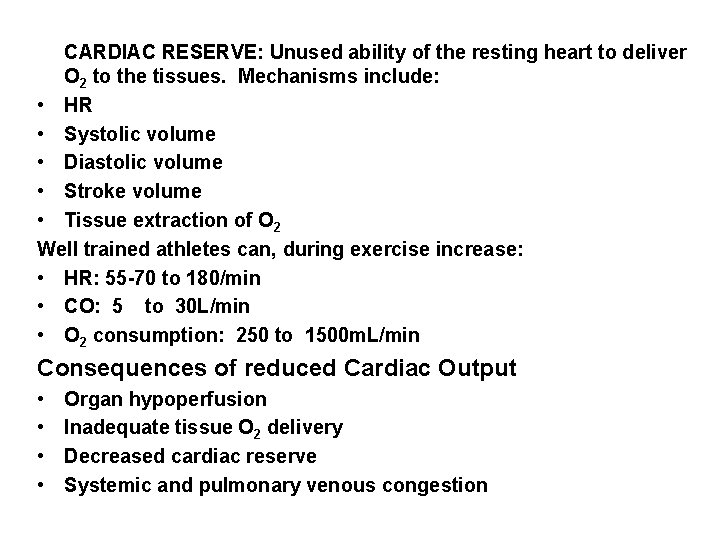
CARDIAC RESERVE: Unused ability of the resting heart to deliver O 2 to the tissues. Mechanisms include: • HR • Systolic volume • Diastolic volume • Stroke volume • Tissue extraction of O 2 Well trained athletes can, during exercise increase: • HR: 55 -70 to 180/min • CO: 5 to 30 L/min • O 2 consumption: 250 to 1500 m. L/min Consequences of reduced Cardiac Output • • Organ hypoperfusion Inadequate tissue O 2 delivery Decreased cardiac reserve Systemic and pulmonary venous congestion
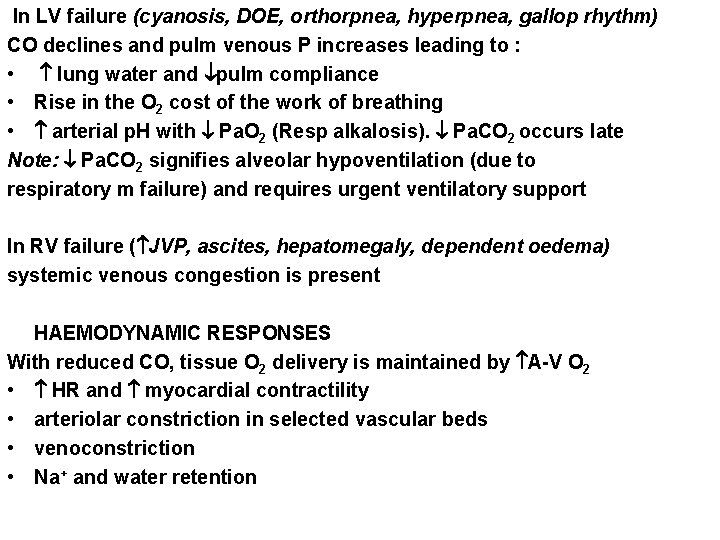
In LV failure (cyanosis, DOE, orthorpnea, hyperpnea, gallop rhythm) CO declines and pulm venous P increases leading to : • lung water and pulm compliance • Rise in the O 2 cost of the work of breathing • arterial p. H with Pa. O 2 (Resp alkalosis). Pa. CO 2 occurs late Note: Pa. CO 2 signifies alveolar hypoventilation (due to respiratory m failure) and requires urgent ventilatory support In RV failure ( JVP, ascites, hepatomegaly, dependent oedema) systemic venous congestion is present HAEMODYNAMIC RESPONSES With reduced CO, tissue O 2 delivery is maintained by A-V O 2 • HR and myocardial contractility • arteriolar constriction in selected vascular beds • venoconstriction • Na+ and water retention
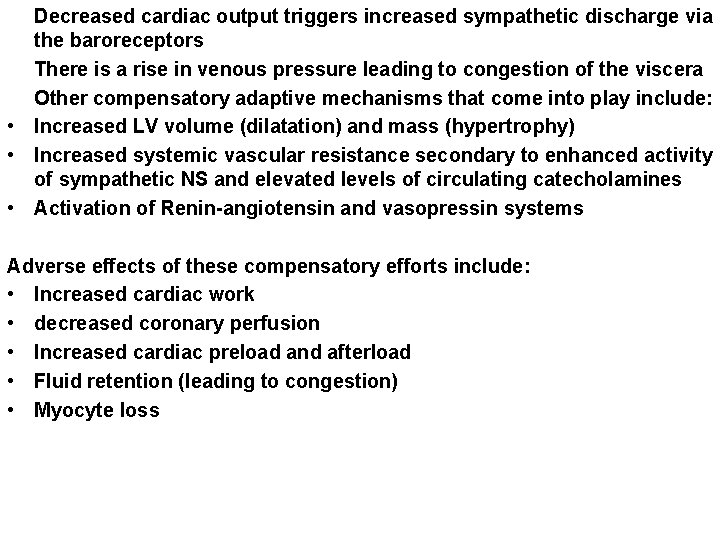
Decreased cardiac output triggers increased sympathetic discharge via the baroreceptors There is a rise in venous pressure leading to congestion of the viscera Other compensatory adaptive mechanisms that come into play include: • Increased LV volume (dilatation) and mass (hypertrophy) • Increased systemic vascular resistance secondary to enhanced activity of sympathetic NS and elevated levels of circulating catecholamines • Activation of Renin-angiotensin and vasopressin systems Adverse effects of these compensatory efforts include: • Increased cardiac work • decreased coronary perfusion • Increased cardiac preload and afterload • Fluid retention (leading to congestion) • Myocyte loss
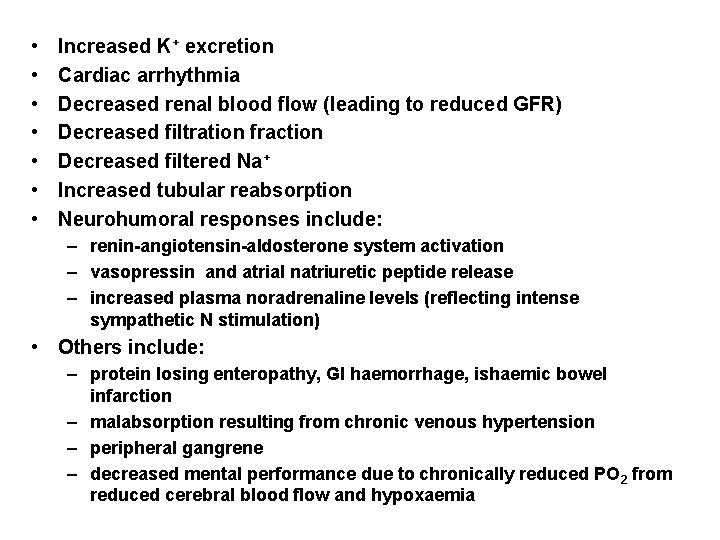
• • Increased K+ excretion Cardiac arrhythmia Decreased renal blood flow (leading to reduced GFR) Decreased filtration fraction Decreased filtered Na+ Increased tubular reabsorption Neurohumoral responses include: – renin-angiotensin-aldosterone system activation – vasopressin and atrial natriuretic peptide release – increased plasma noradrenaline levels (reflecting intense sympathetic N stimulation) • Others include: – protein losing enteropathy, GI haemorrhage, ishaemic bowel infarction – malabsorption resulting from chronic venous hypertension – peripheral gangrene – decreased mental performance due to chronically reduced PO 2 from reduced cerebral blood flow and hypoxaemia
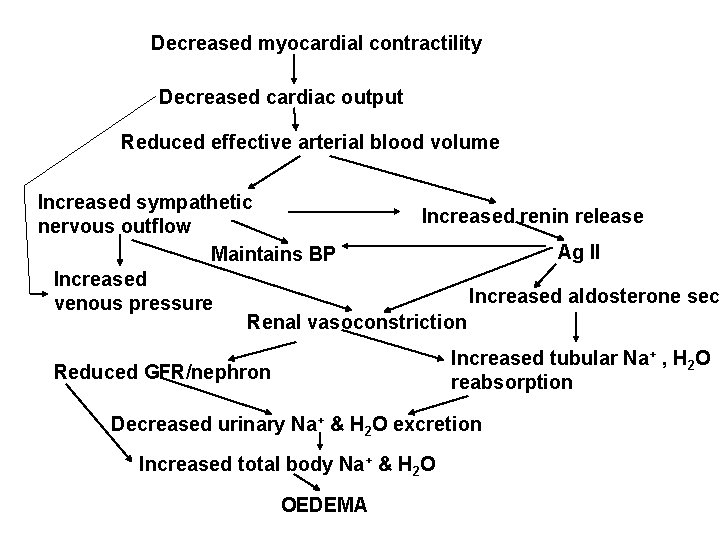
Decreased myocardial contractility Decreased cardiac output Reduced effective arterial blood volume Increased sympathetic Increased renin release nervous outflow Ag II Maintains BP Increased aldosterone sec venous pressure Renal vasoconstriction Increased tubular Na+ , H 2 O reabsorption Reduced GFR/nephron Decreased urinary Na+ & H 2 O excretion Increased total body Na+ & H 2 O OEDEMA
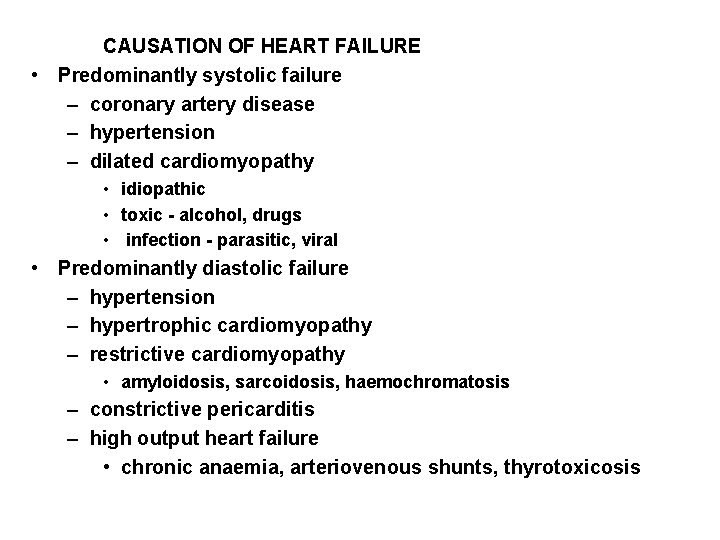
CAUSATION OF HEART FAILURE • Predominantly systolic failure – coronary artery disease – hypertension – dilated cardiomyopathy • idiopathic • toxic - alcohol, drugs • infection - parasitic, viral • Predominantly diastolic failure – hypertension – hypertrophic cardiomyopathy – restrictive cardiomyopathy • amyloidosis, sarcoidosis, haemochromatosis – constrictive pericarditis – high output heart failure • chronic anaemia, arteriovenous shunts, thyrotoxicosis
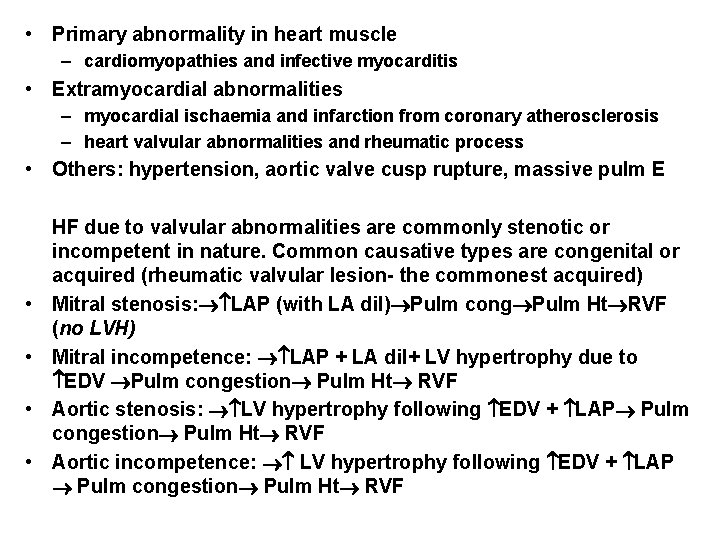
• Primary abnormality in heart muscle – cardiomyopathies and infective myocarditis • Extramyocardial abnormalities – myocardial ischaemia and infarction from coronary atherosclerosis – heart valvular abnormalities and rheumatic process • Others: hypertension, aortic valve cusp rupture, massive pulm E • • HF due to valvular abnormalities are commonly stenotic or incompetent in nature. Common causative types are congenital or acquired (rheumatic valvular lesion- the commonest acquired) Mitral stenosis: LAP (with LA dil) Pulm cong Pulm Ht RVF (no LVH) Mitral incompetence: LAP + LA dil+ LV hypertrophy due to EDV Pulm congestion Pulm Ht RVF Aortic stenosis: LV hypertrophy following EDV + LAP Pulm congestion Pulm Ht RVF Aortic incompetence: LV hypertrophy following EDV + LAP Pulm congestion Pulm Ht RVF
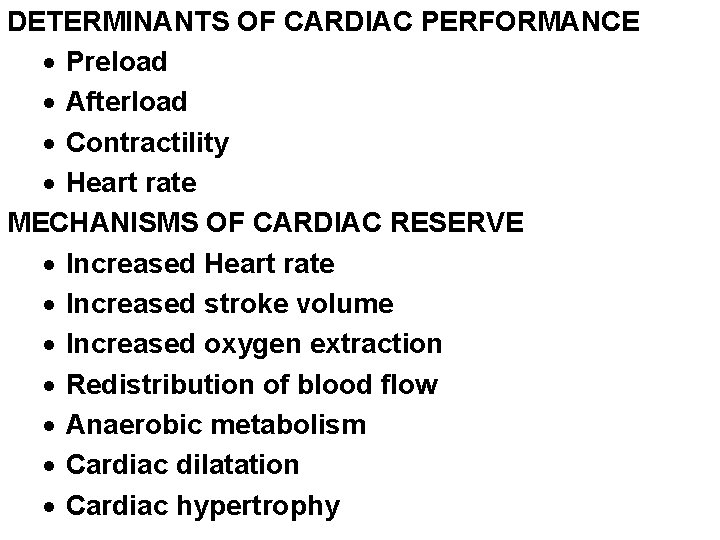
DETERMINANTS OF CARDIAC PERFORMANCE · Preload · Afterload · Contractility · Heart rate MECHANISMS OF CARDIAC RESERVE · Increased Heart rate · Increased stroke volume · Increased oxygen extraction · Redistribution of blood flow · Anaerobic metabolism · Cardiac dilatation · Cardiac hypertrophy