Novel inexpensive twodimensional magnetooptical trap 2 DMOT for
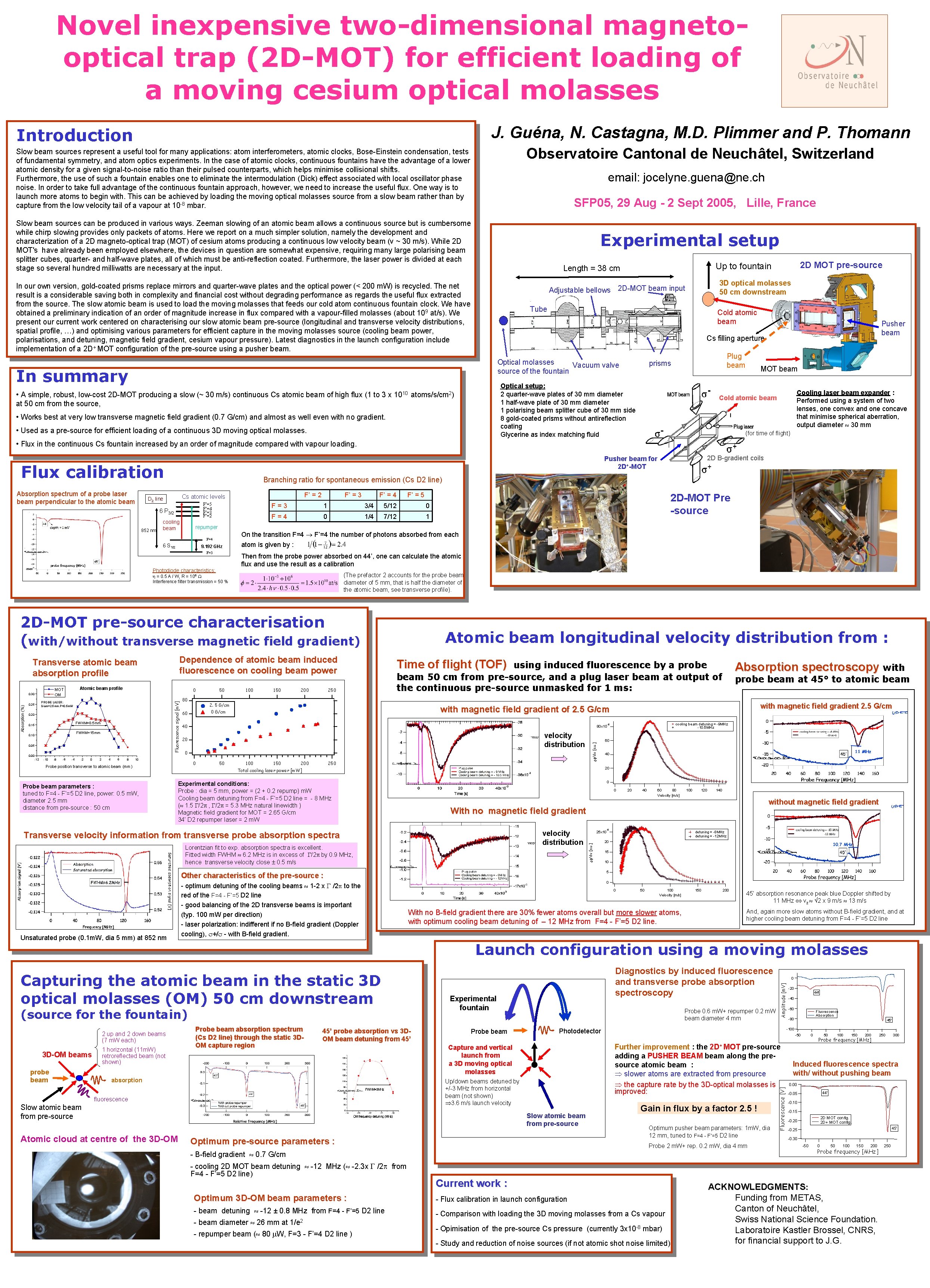
- Slides: 1
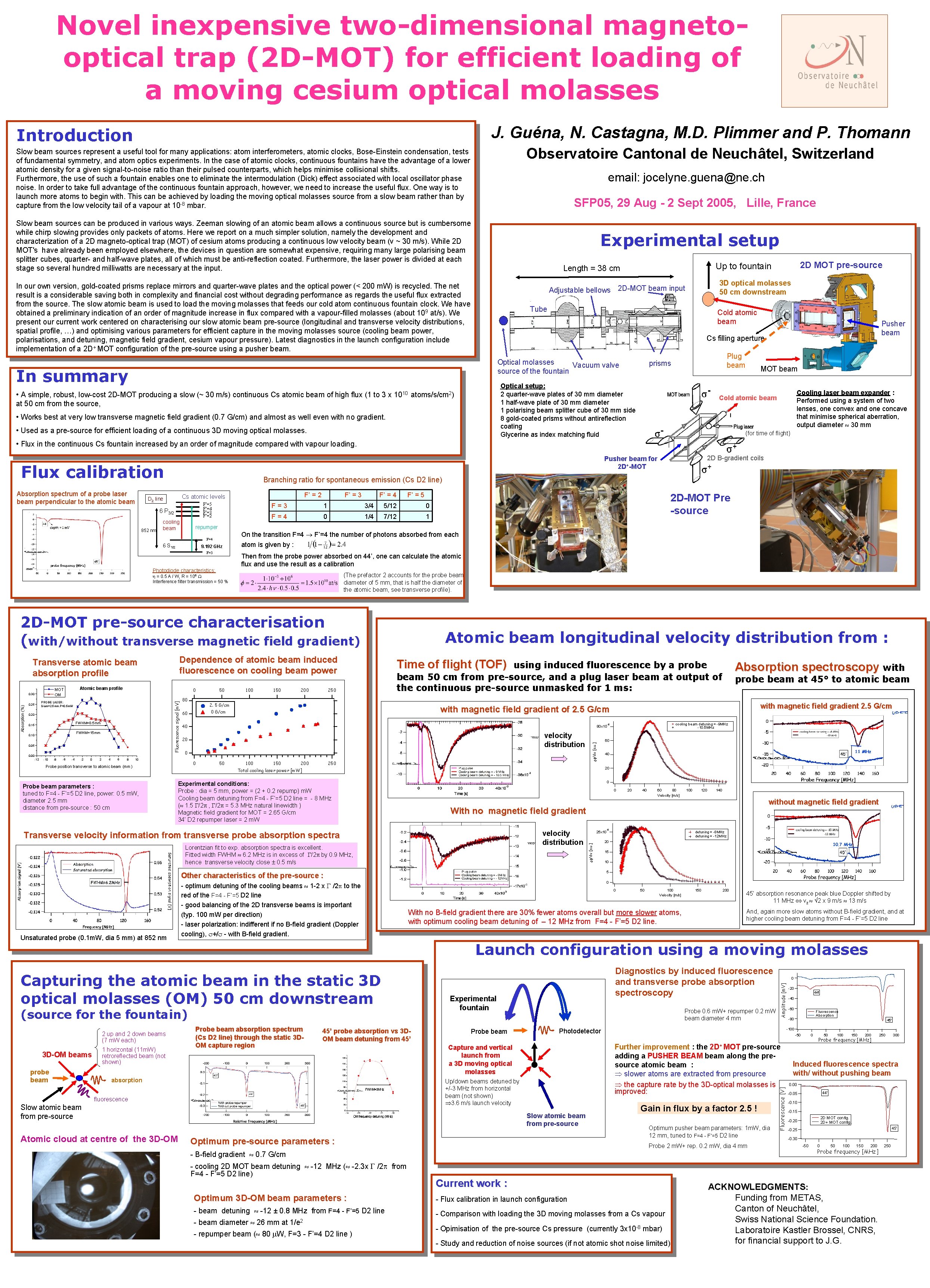
Novel inexpensive two-dimensional magnetooptical trap (2 D-MOT) for efficient loading of a moving cesium optical molasses J. Guéna, N. Castagna, M. D. Plimmer and P. Thomann Introduction Observatoire Cantonal de Neuchâtel, Switzerland Slow beam sources represent a useful tool for many applications: atom interferometers, atomic clocks, Bose-Einstein condensation, tests of fundamental symmetry, and atom optics experiments. In the case of atomic clocks, continuous fountains have the advantage of a lower atomic density for a given signal-to-noise ratio than their pulsed counterparts, which helps minimise collisional shifts. Furthermore, the use of such a fountain enables one to eliminate the intermodulation (Dick) effect associated with local oscillator phase noise. In order to take full advantage of the continuous fountain approach, however, we need to increase the useful flux. One way is to launch more atoms to begin with. This can be achieved by loading the moving optical molasses source from a slow beam rather than by capture from the low velocity tail of a vapour at 10 -8 mbar. email: jocelyne. guena@ne. ch SFP 05, 29 Aug - 2 Sept 2005, Lille, France Slow beam sources can be produced in various ways. Zeeman slowing of an atomic beam allows a continuous source but is cumbersome while chirp slowing provides only packets of atoms. Here we report on a much simpler solution, namely the development and characterization of a 2 D magneto-optical trap (MOT) of cesium atoms producing a continuous low velocity beam (v ~ 30 m/s). While 2 D MOT's have already been employed elsewhere, the devices in question are somewhat expensive, requiring many large polarising beam splitter cubes, quarter- and half-wave plates, all of which must be anti-reflection coated. Furthermore, the laser power is divided at each stage so several hundred milliwatts are necessary at the input. Experimental setup In our own version, gold-coated prisms replace mirrors and quarter-wave plates and the optical power (< 200 m. W) is recycled. The net result is a considerable saving both in complexity and financial cost without degrading performance as regards the useful flux extracted from the source. The slow atomic beam is used to load the moving molasses that feeds our cold atom continuous fountain clock. We have obtained a preliminary indication of an order of magnitude increase in flux compared with a vapour-filled molasses (about 10 9 at/s). We present our current work centered on characterising our slow atomic beam pre-source (longitudinal and transverse velocity distributions, spatial profile, …) and optimising various parameters for efficient capture in the moving molasses source (cooling beam power, polarisations, and detuning, magnetic field gradient, cesium vapour pressure). Latest diagnostics in the launch configuration include implementation of a 2 D+ MOT configuration of the pre-source using a pusher beam. 2 D MOT pre-source Up to fountain Length = 38 cm 3 D optical molasses 50 cm downstream Adjustable bellows 2 D-MOT beam input Tube Cold atomic beam Pusher beam Cs filling aperture Optical molasses Vacuum valve source of the fountain In summary Plug beam prisms Optical setup: 2 quarter-wave plates of 30 mm diameter 1 half-wave plate of 30 mm diameter 1 polarising beam splitter cube of 30 mm side 8 gold-coated prisms without antireflection coating Glycerine as index matching fluid • A simple, robust, low-cost 2 D-MOT producing a slow (~ 30 m/s) continuous Cs atomic beam of high flux (1 to 3 x 10 10 atoms/s/cm 2) at 50 cm from the source, • Works best at very low transverse magnetic field gradient (0. 7 G/cm) and almost as well even with no gradient. • Used as a pre-source for efficient loading of a continuous 3 D moving optical molasses. MOT beam Cooling laser beam expander : Performed using a system of two lenses, one convex and one concave that minimise spherical aberration, output diameter 30 mm Cold atomic beam (for time of flight) • Flux in the continuous Cs fountain increased by an order of magnitude compared with vapour loading. Flux calibration Branching ratio for spontaneous emission (Cs D 2 line) F’=5 F’=4 F’=3 F’=2 6 P 3/2 repumper F’ = 5 F=3 1 3/4 5/12 0 F=4 0 1/4 7/12 1 9. 192 GHz F=3 atom is given by : Then from the probe power absorbed on 44’, one can calculate the atomic flux and use the result as a calibration Photodiode characteristics: (The prefactor 2 accounts for the probe beam diameter of 5 mm, that is half the diameter of the atomic beam, see transverse profile). = 0. 5 A / W, R = 106 Interference filter transmission = 50 % 2 D-MOT pre-source characterisation (with/without transverse magnetic field gradient) Dependence of atomic beam induced fluorescence on cooling beam power 0. 30 0 PROBE LASER: Diam=2. 5 mm, P=0. 5 m. W 0. 25 Fluorescence signal [m. V] Absorption (%) Atomic beam profile MOT OM 0. 20 FWHM=8. 5 mm 0. 15 FWHM=15 mm 0. 10 0. 05 0. 00 -12 -10 -8 -6 -4 -2 0 2 4 6 8 80 100 150 200 250 Time of flight (TOF) using induced fluorescence by a probe beam 50 cm from pre-source, and a plug laser beam at output of the continuous pre-source unmasked for 1 ms: 2. 5 G/cm 0 G/cm 60 80 x 10 velocity distribution 20 0 0 Probe position transverse to atomic beam (mm) 50 100 150 200 250 probe beam 40 20 40 60 80 Velocity [m/s] 100 120 140 without magnetic field gradient velocity distribution 25 x 10 -6 detuning = -8 MHz detuning = -12 MHz 20 15 10 Other characteristics of the pre-source : 5 - optimum detuning of the cooling beams 1 -2 x /2 to the red of the F=4 - F’=5 D 2 line - good balancing of the 2 D transverse beams is important (typ. 100 m. W per direction) - laser polarization: indifferent if no B-field gradient (Doppler cooling), +/ - with B-field gradient. 0 0 50 100 Velocity [m/s] With no B-field gradient there are 30% fewer atoms overall but more slower atoms, with optimum cooling beam detuning of – 12 MHz from F=4 - F’=5 D 2 line. 150 200 45’ absorption resonance peak blue Doppler shifted by 11 MHz vz 2 x 9 m/s 13 m/s And, again more slow atoms without B-field gradient, and at higher cooling beam detuning from F=4 - F’=5 D 2 line Launch configuration using a moving molasses Probe beam absorption spectrum (Cs D 2 line) through the static 3 DOM capture region 45’ probe absorption vs 3 DOM beam detuning from 45’ absorption Diagnostics by induced fluorescence and transverse probe absorption spectroscopy Experimental fountain Probe beam Probe 0. 6 m. W+ repumper 0. 2 m. W beam diameter 4 mm Slow atomic beam from pre-source Optimum pre-source parameters : Gain in flux by a factor 2. 5 ! Optimum pusher beam parameters: 1 m. W, dia 12 mm, tuned to F=4 - F’=5 D 2 line Current work : Optimum 3 D-OM beam parameters : - Flux calibration in launch configuration - beam detuning -12 ± 0. 8 MHz from F=4 - F’=5 D 2 line - Comparison with loading the 3 D moving molasses from a Cs vapour - beam diameter 26 mm at 1/e 2 - repumper beam ( 80 W, F=3 - F’=4 D 2 line ) 44' -40 -60 Fluorescence Absorption -80 45' -50 Probe 2 m. W+ rep. 0. 2 m. W, dia 4 mm - B-field gradient 0. 7 G/cm -20 -100 Further improvement : the 2 D+ MOT pre-source adding a PUSHER BEAM beam along the presource atomic beam : slower atoms are extracted from presource the capture rate by the 3 D-optical molasses is improved: Capture and vertical launch from a 3 D moving optical molasses Slow atomic beam from pre-source - cooling 2 D MOT beam detuning -12 MHz ( -2. 3 x /2 from F=4 - F’=5 D 2 line) 0 Photodetector Up/down beams detuned by +/-3 MHz from horizontal beam (not shown) 3. 6 m/s launch velocity fluorescence Atomic cloud at centre of the 3 D-OM 60 With no magnetic field gradient (source for the fountain) 3 D-OM beams + cooling beam detuning = -9 MHz + -10. 5 MHz -6 0 Capturing the atomic beam in the static 3 D optical molasses (OM) 50 cm downstream 1 horizontal (11 m. W) retroreflected beam (not shown) with magnetic field gradient 2. 5 G/cm 0 Experimental conditions: Probe : dia = 5 mm, power = (2 + 0. 2 repump) m. W Cooling beam detuning from F=4 - F’=5 D 2 line = - 8 MHz ( 1. 5 /2 , /2 = 5. 3 MHz natural linewidth ) Magnetic field gradient for MOT = 2. 65 G/cm 34’ D 2 repumper laser = 2 m. W Lorentzian fit to exp. absorption spectra is excellent. Fitted width FWHM 6. 2 MHz is in excess of /2 by 0. 9 MHz, hence transverse velocity close 0. 5 m/s 2 up and 2 down beams (7 m. W each) probe beam at 45° to atomic beam 20 Total cooling laser power [m. W] Transverse velocity information from transverse probe absorption spectra Unsaturated probe (0. 1 m. W, dia 5 mm) at 852 nm Absorption spectroscopy with magnetic field gradient of 2. 5 G/cm 40 10 Probe beam parameters : tuned to F=4 - F’=5 D 2 line, power: 0. 5 m. W, diameter 2. 5 mm distance from pre-source : 50 cm 50 Atomic beam longitudinal velocity distribution from : Amplitude [m. V] Transverse atomic beam absorption profile 2 D-MOT Pre -source On the transition F=4 F’=4 the number of photons absorbed from each F=4 6 S 1/2 F’ = 4 - Opimisation of the pre-source Cs pressure (currently 3 x 10 -8 mbar) - Study and reduction of noise sources (if not atomic shot noise limited) Fluorescence [V] cooling beam F’ = 3 d f/dv [a. u. ] 852 nm F’ = 2 Cs atomic levels D 2 line d f/dv [a. u. ] Absorption spectrum of a probe laser beam perpendicular to the atomic beam 2 D B-gradient coils Pusher beam for 2 D+-MOT 0 50 100 150 200 Probe frequency [MHz] 250 300 Induced fluorescence spectra with/ without pushing beam 0. 00 44' -0. 05 -0. 10 -0. 15 2 D MOT config. 2 D+ MOT config. -0. 20 45' -0. 25 -0. 30 -50 0 50 100 150 200 Probe frequency [MHz] ACKNOWLEDGMENTS: Funding from METAS, Canton of Neuchâtel, Swiss National Science Foundation. Laboratoire Kastler Brossel, CNRS, for financial support to J. G. 250