Ionospheric Modeling and its Challenges due to Space
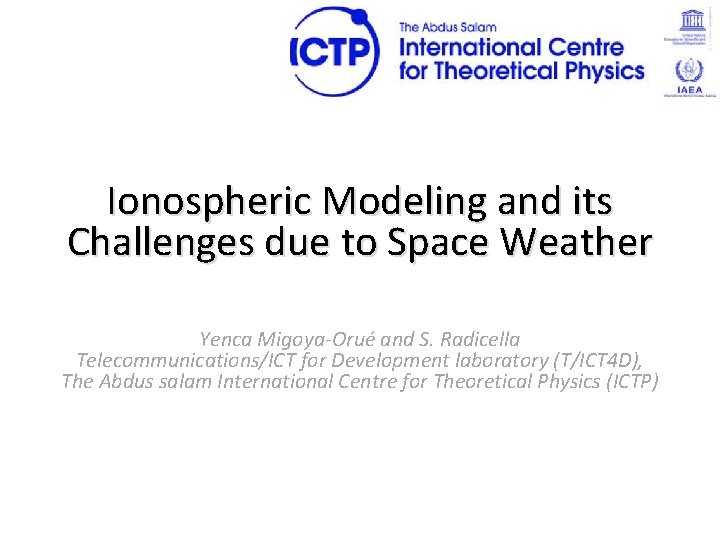
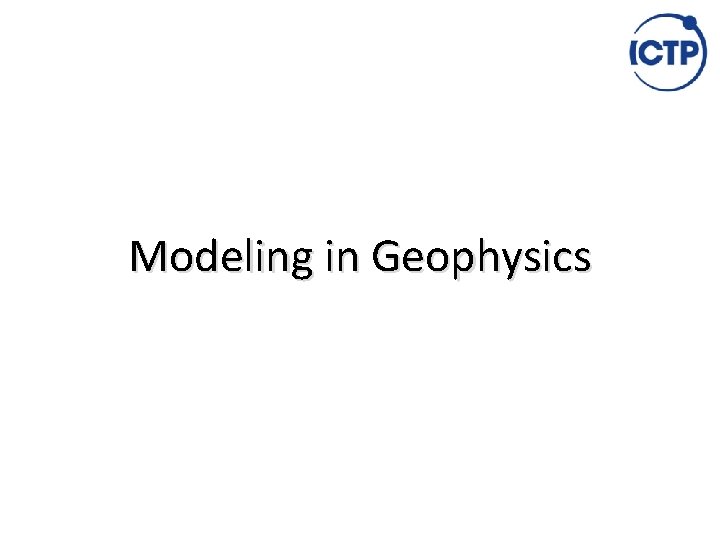
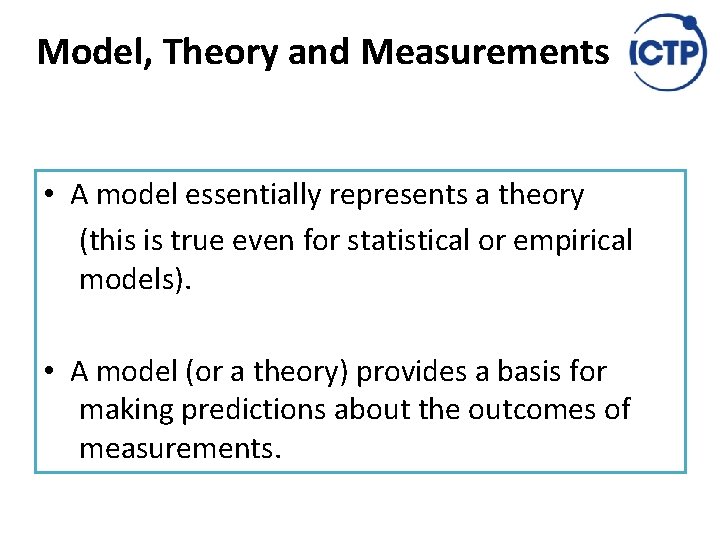
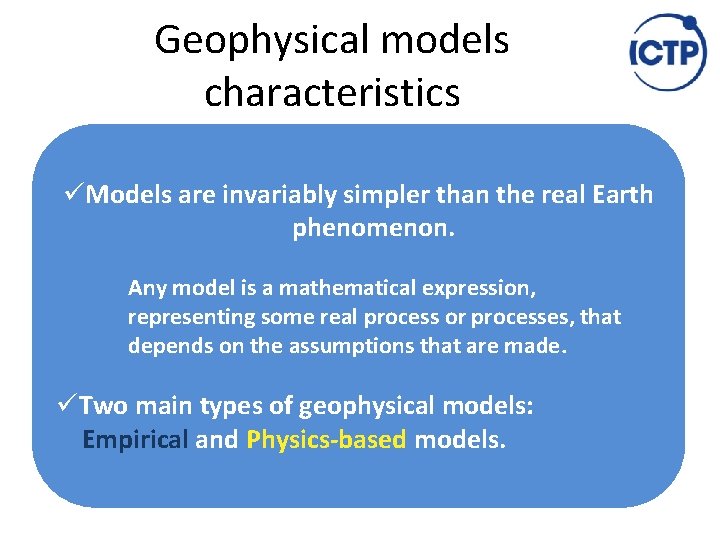
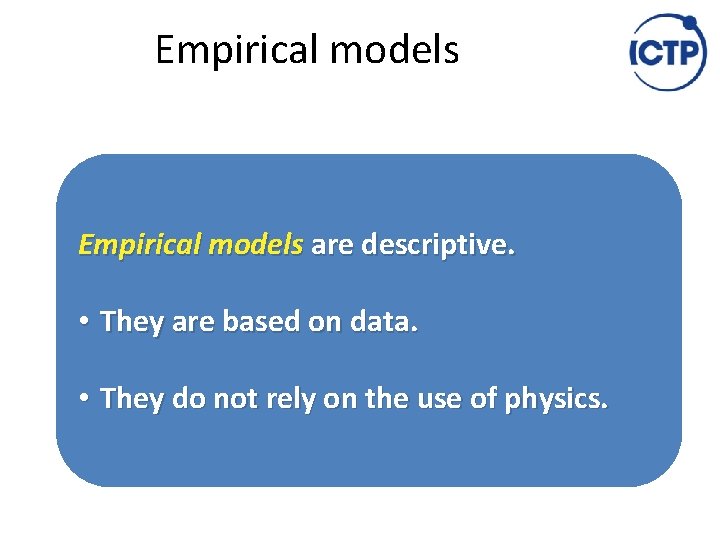
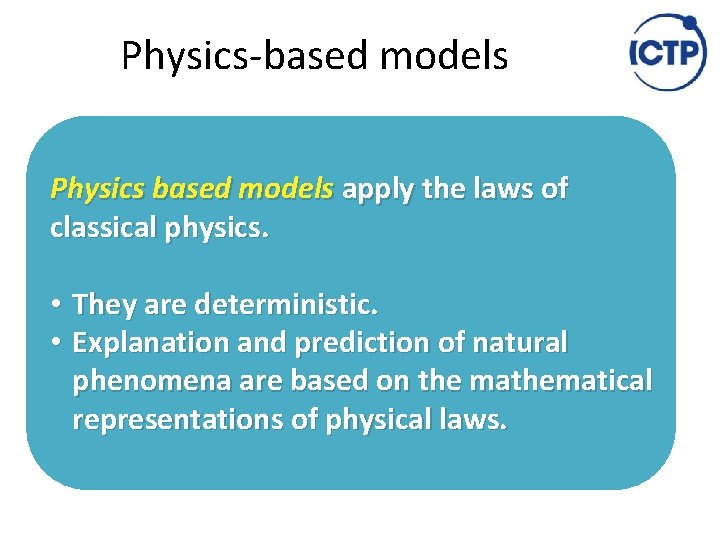
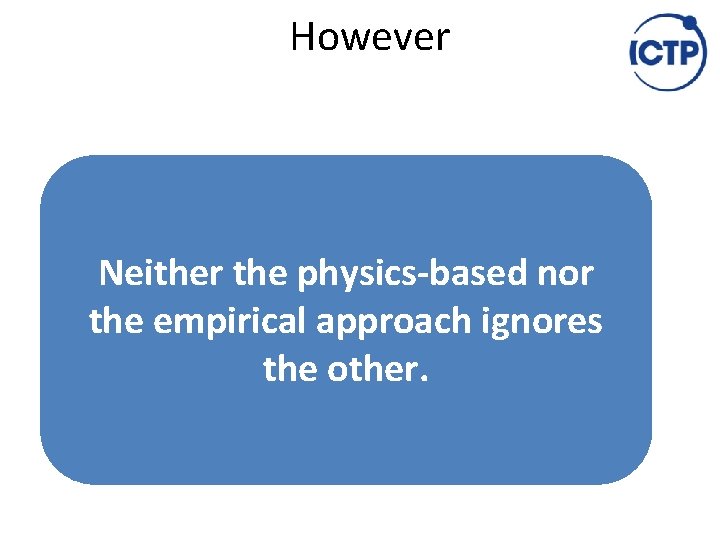
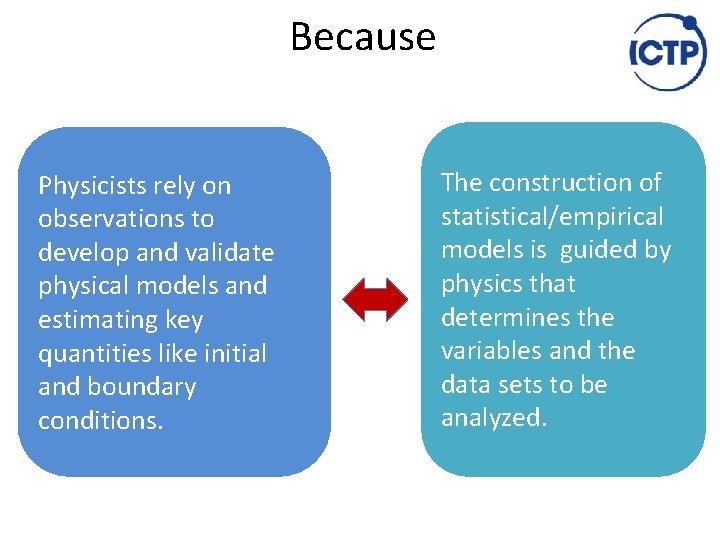
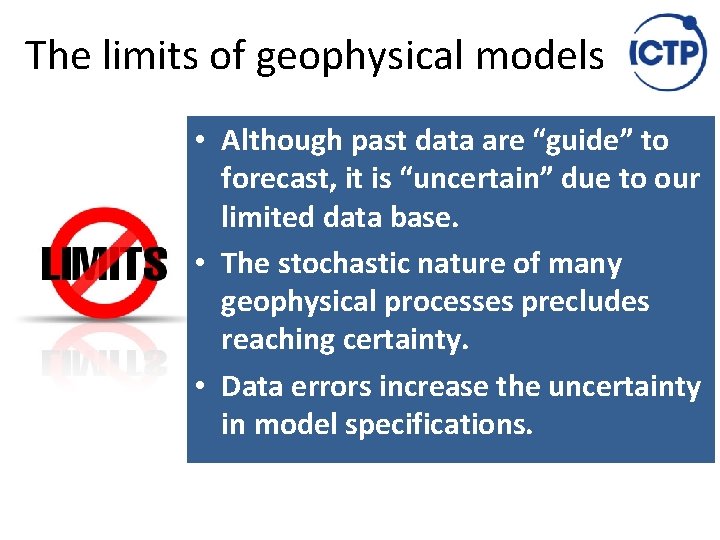
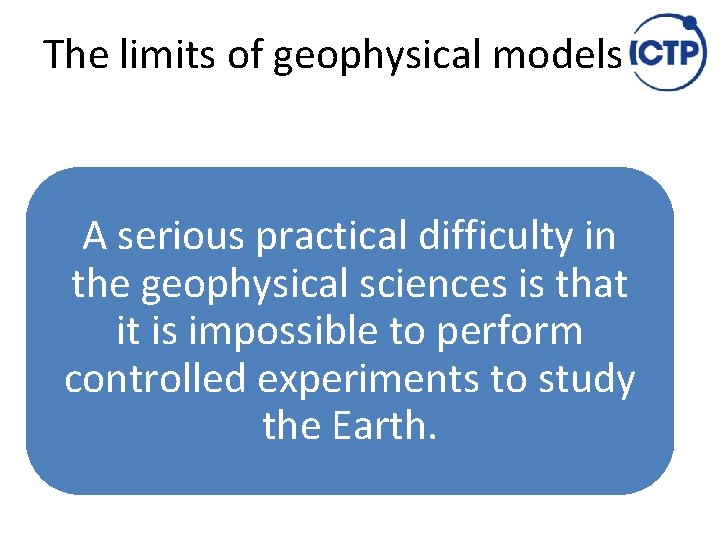
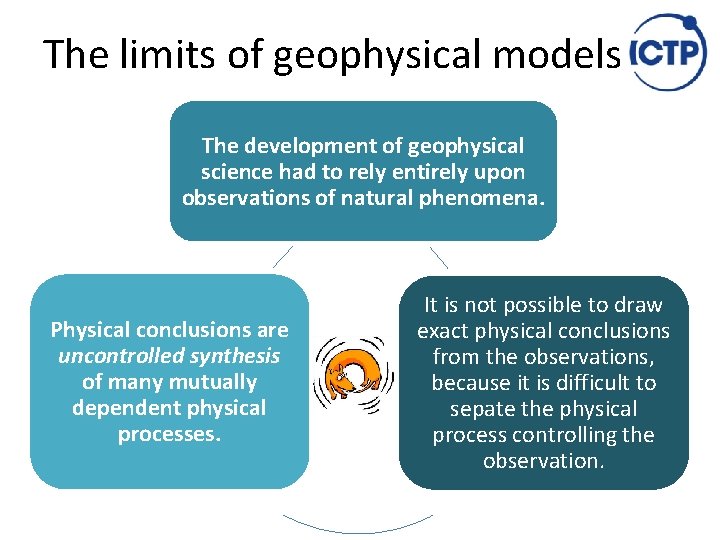
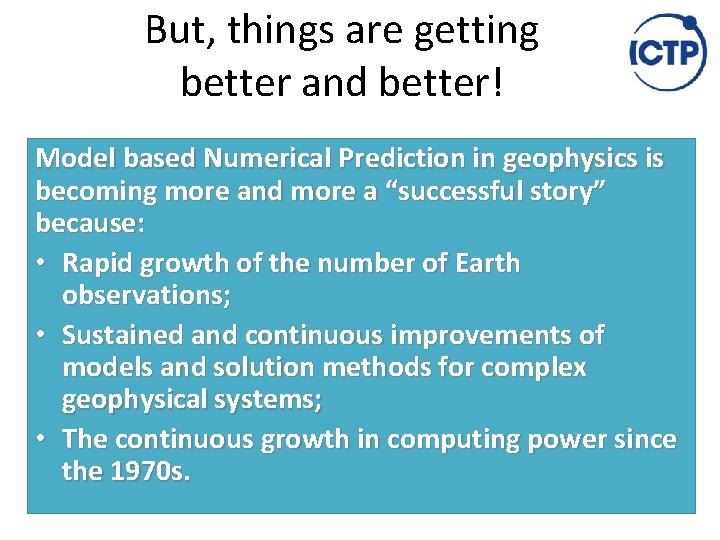
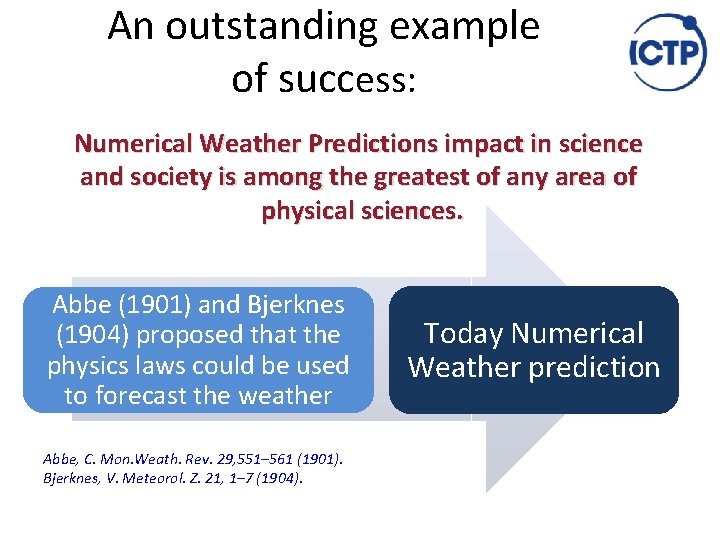
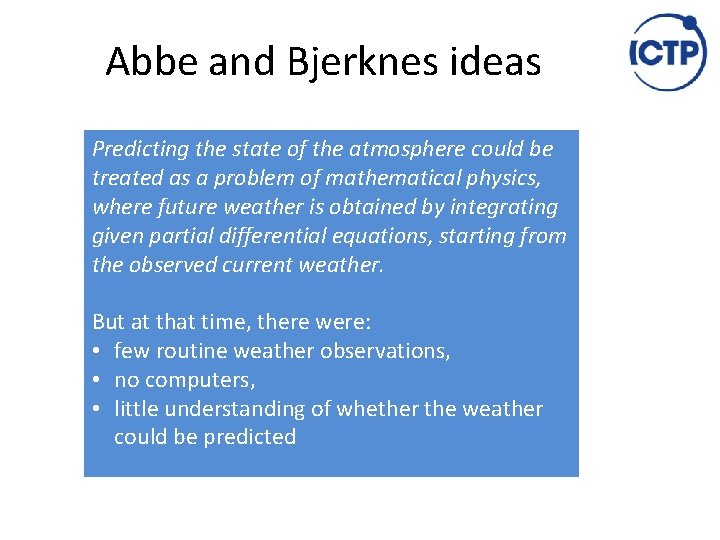
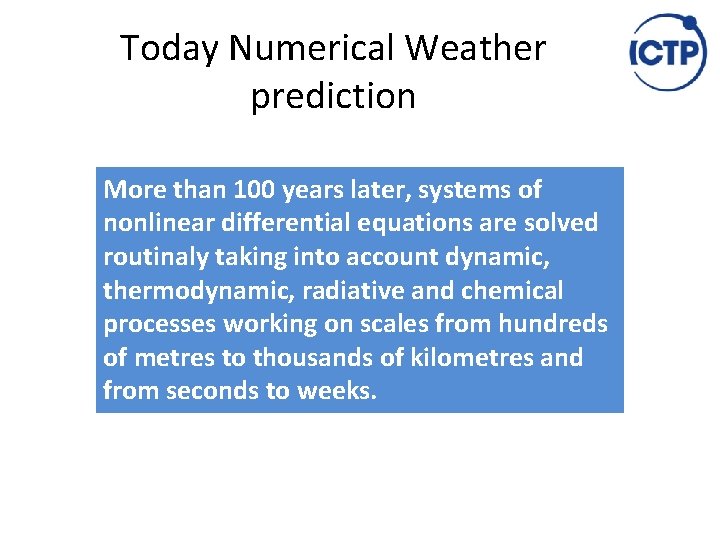
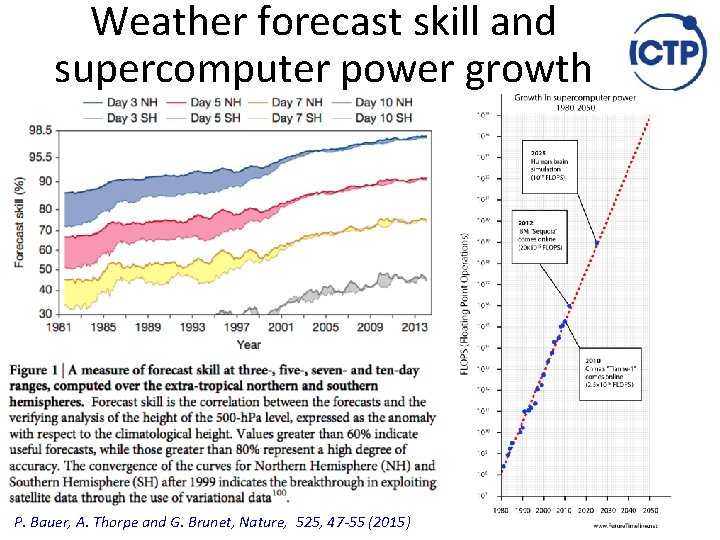
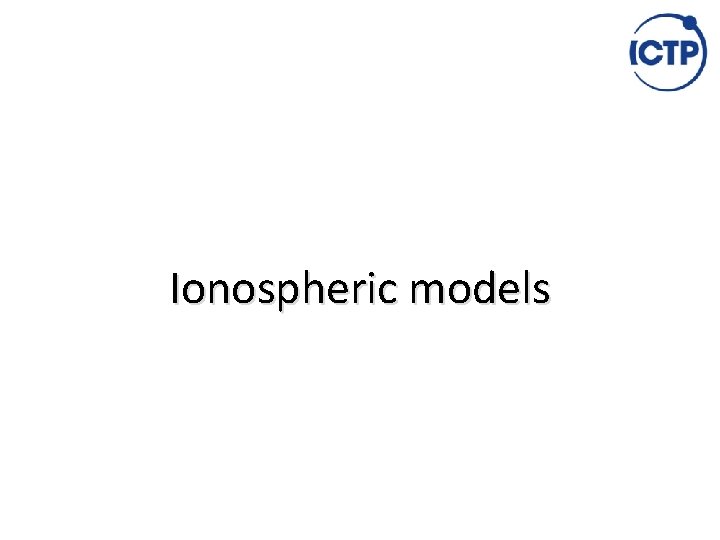
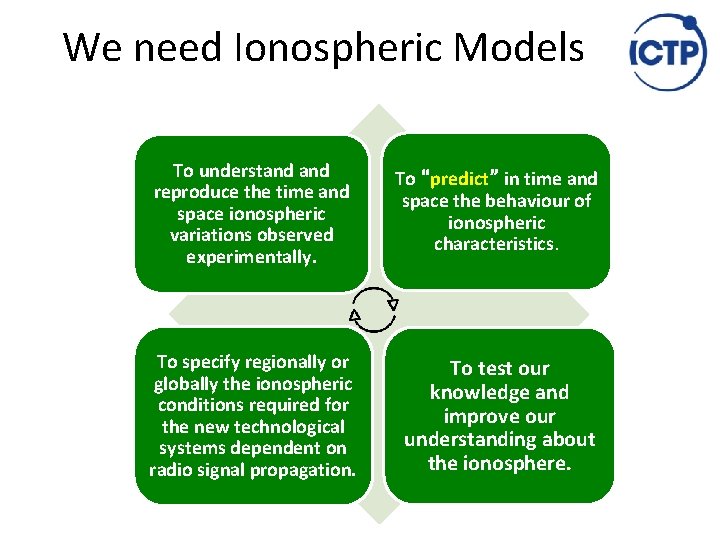
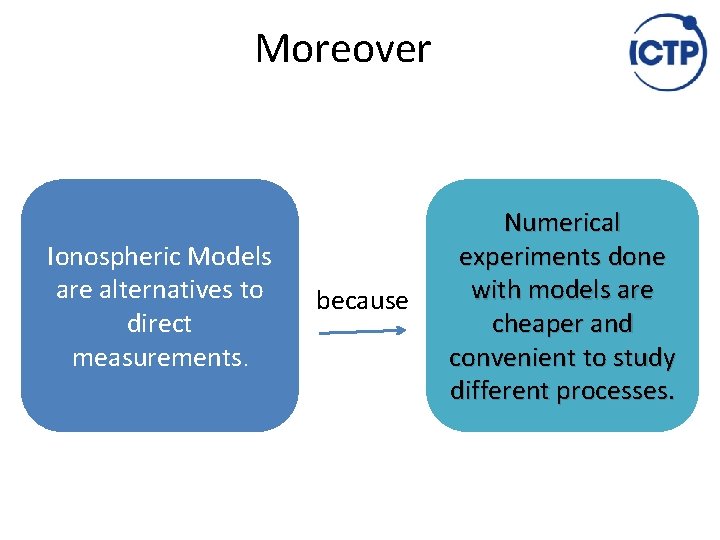
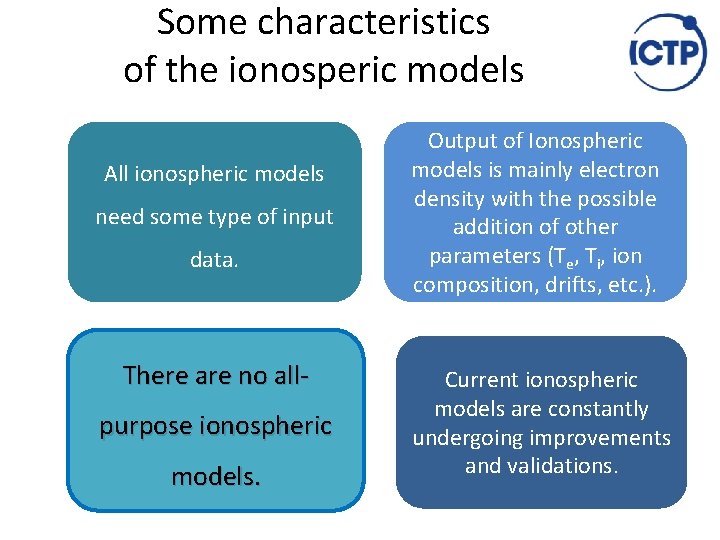
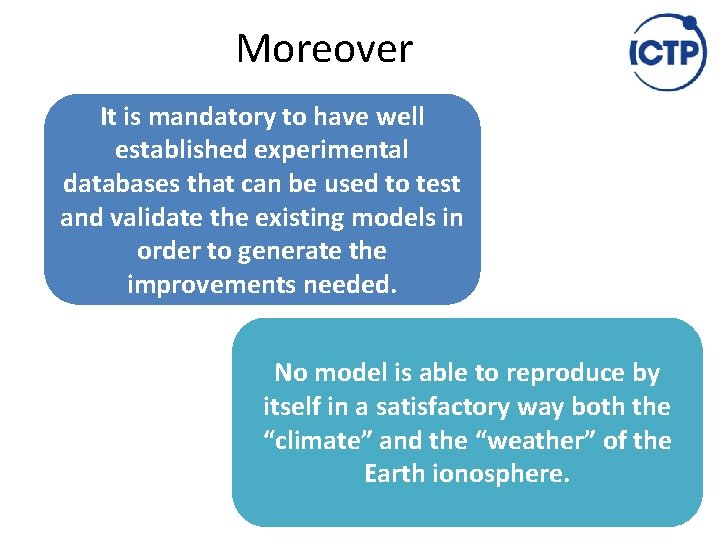
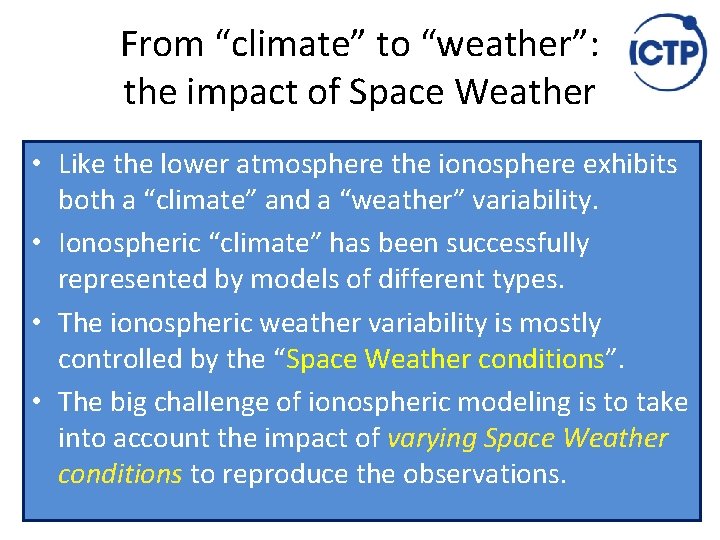
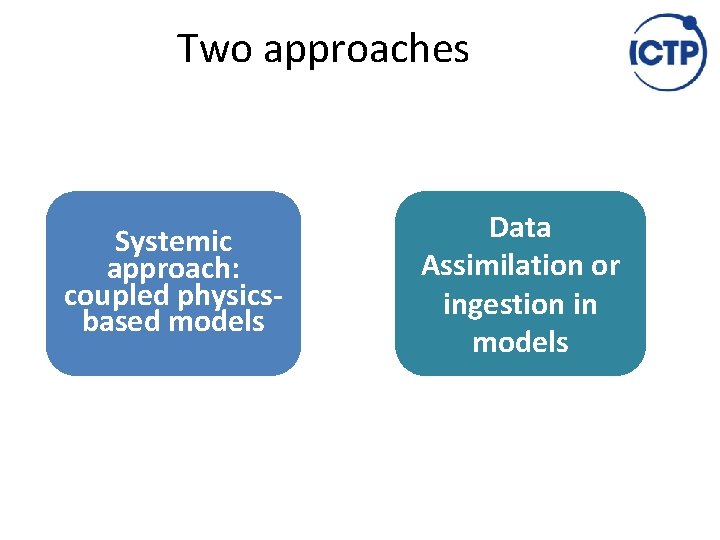
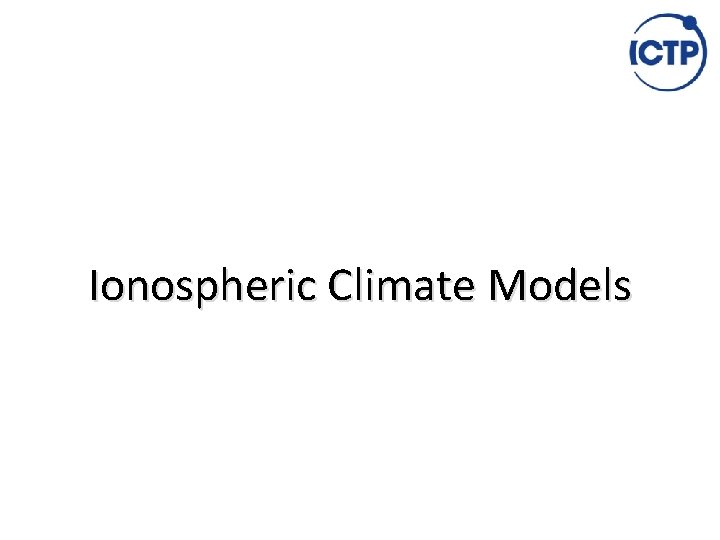
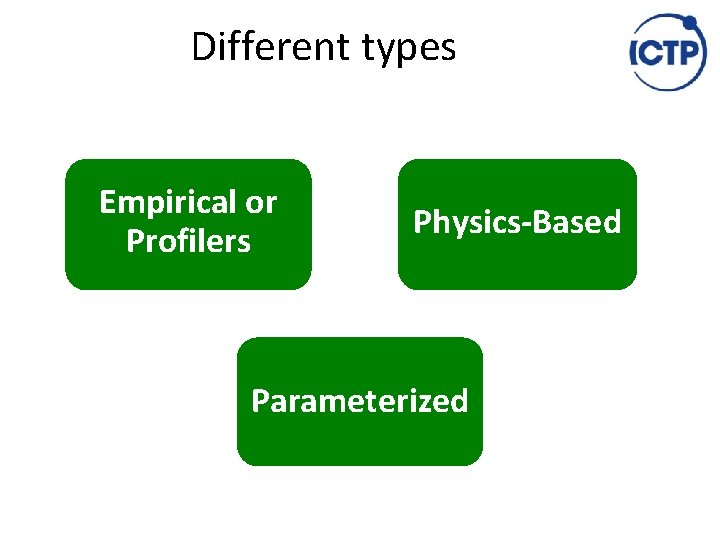
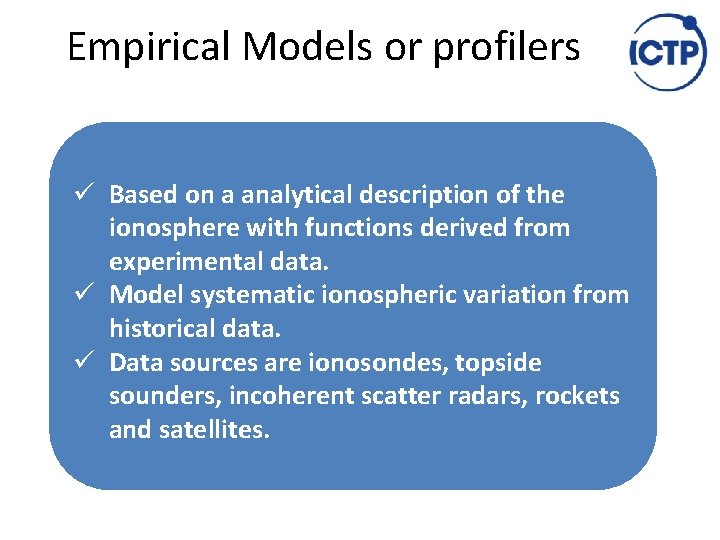
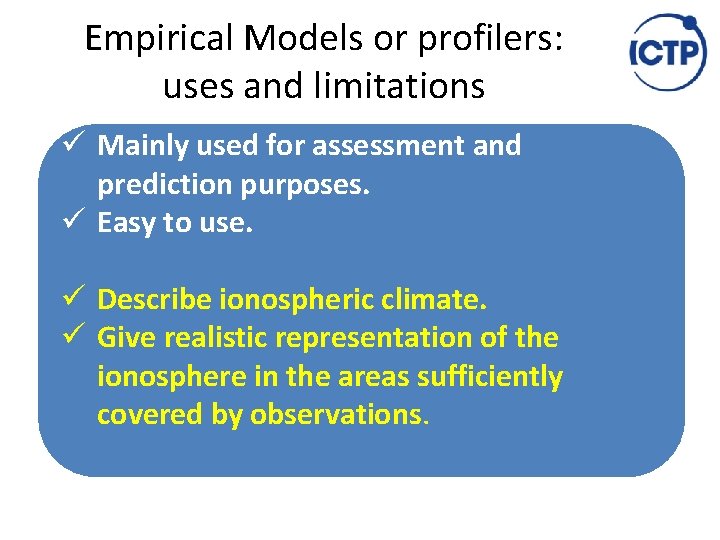
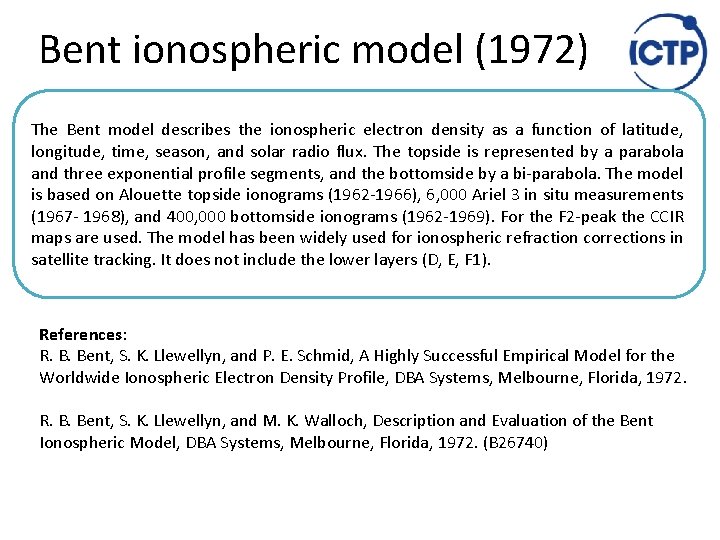
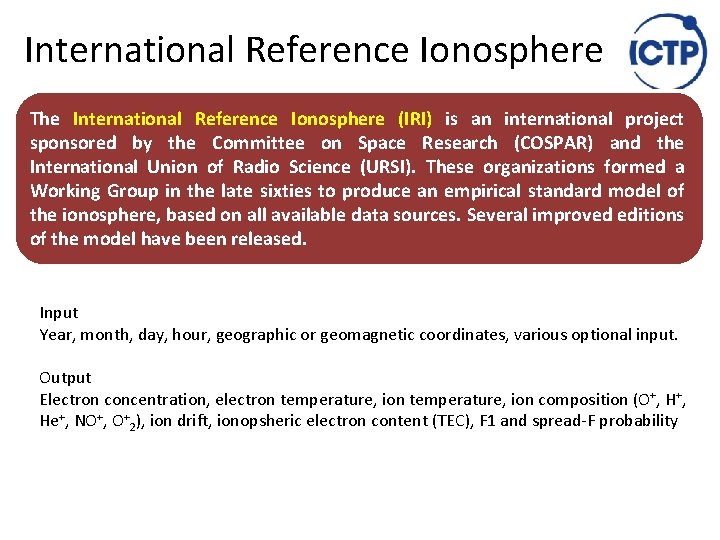
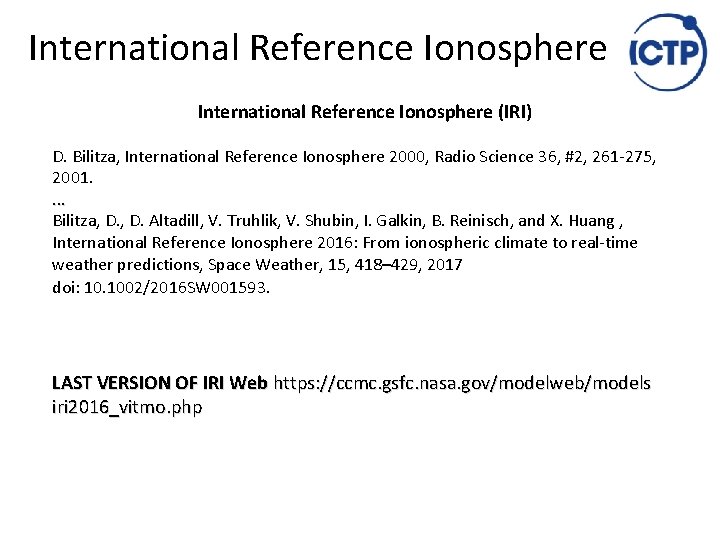
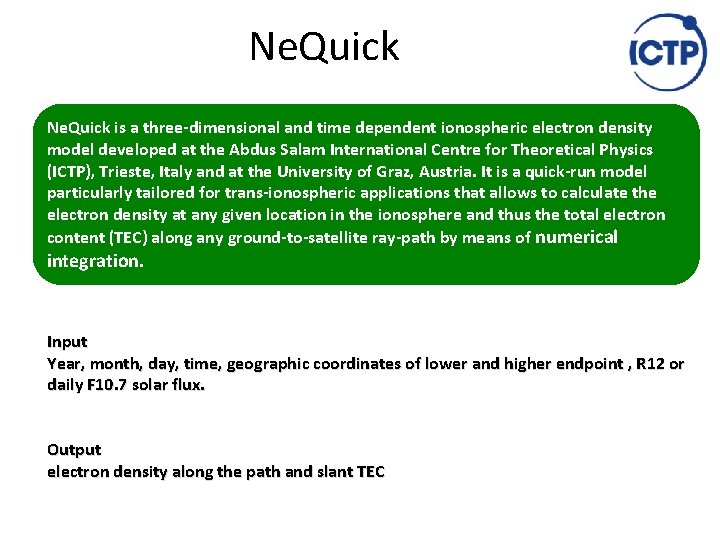
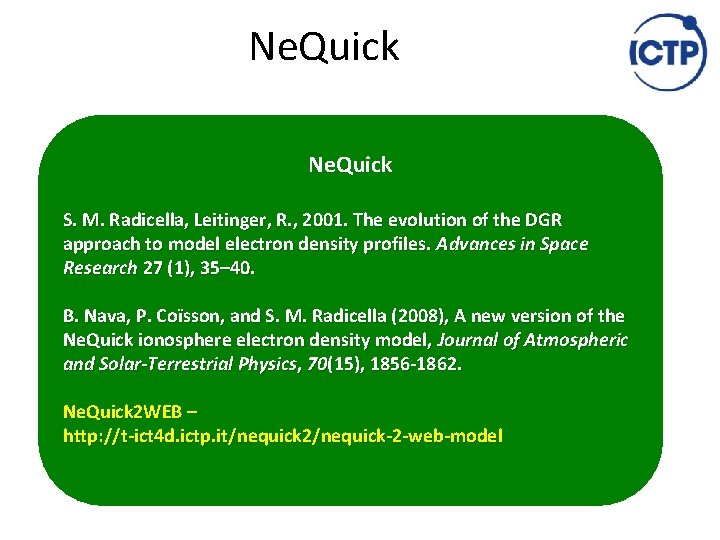
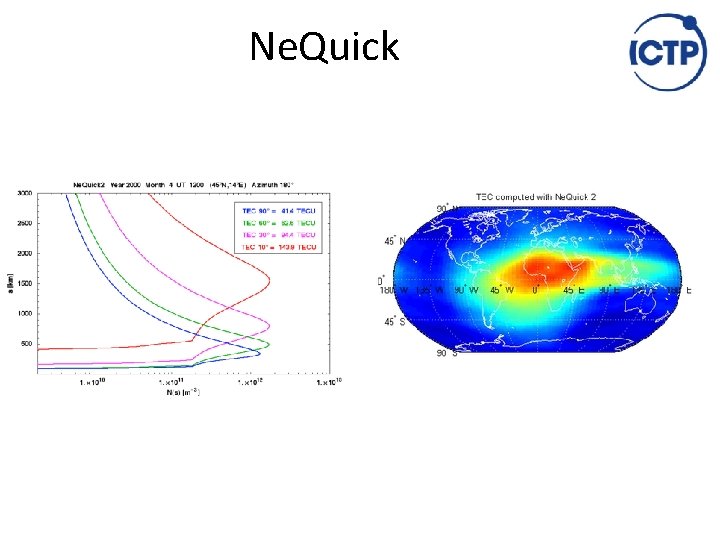
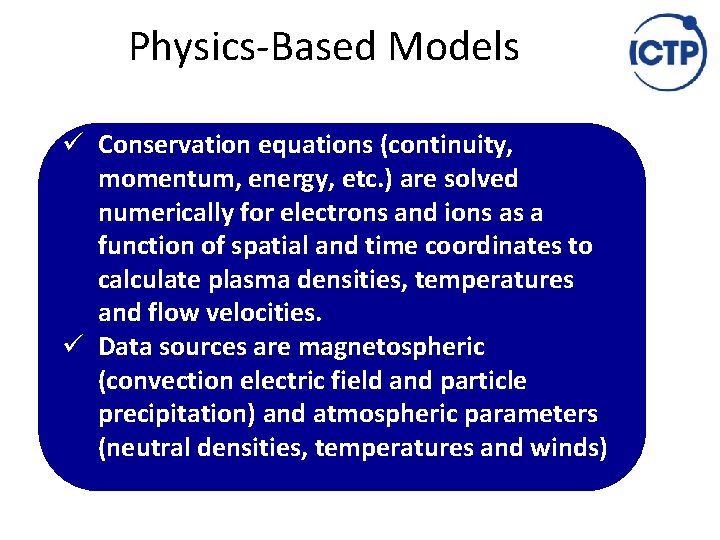
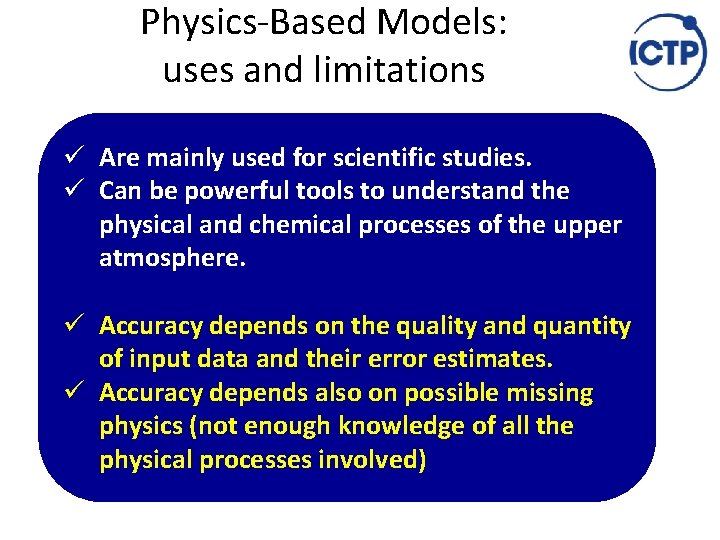
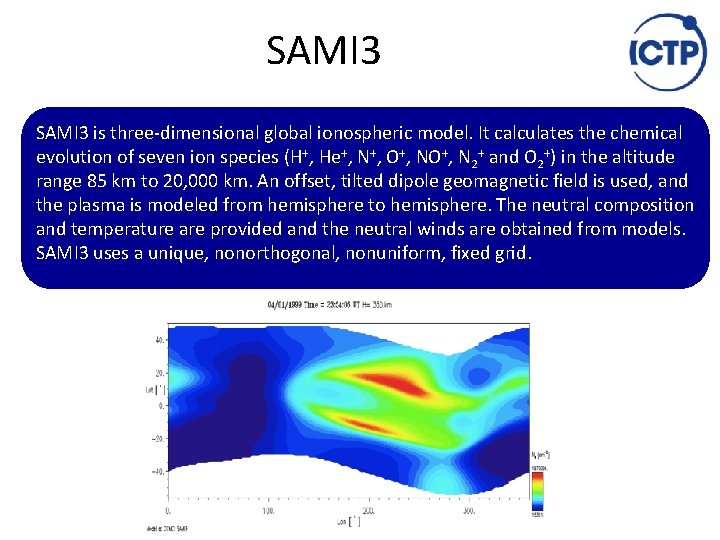
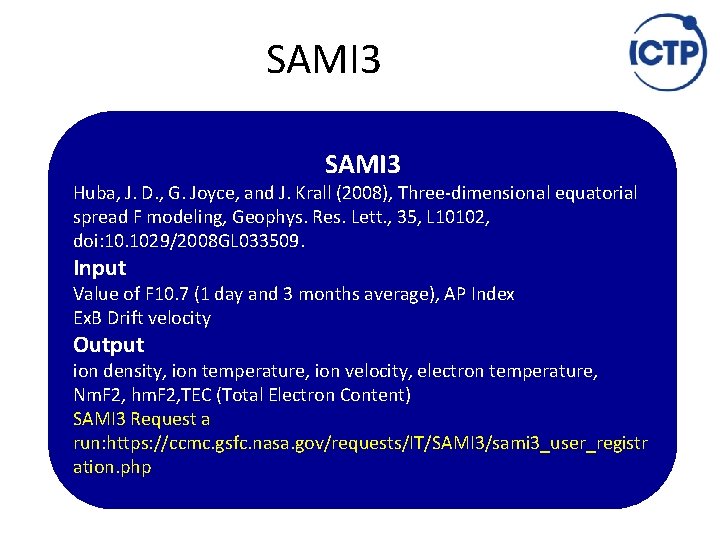
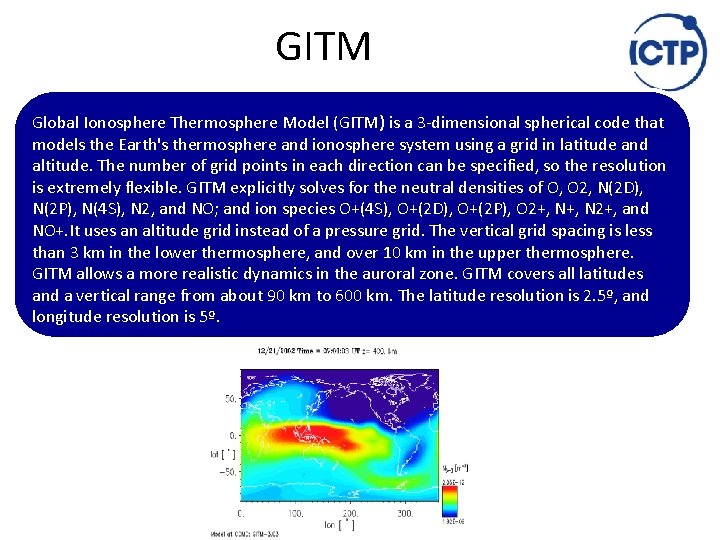
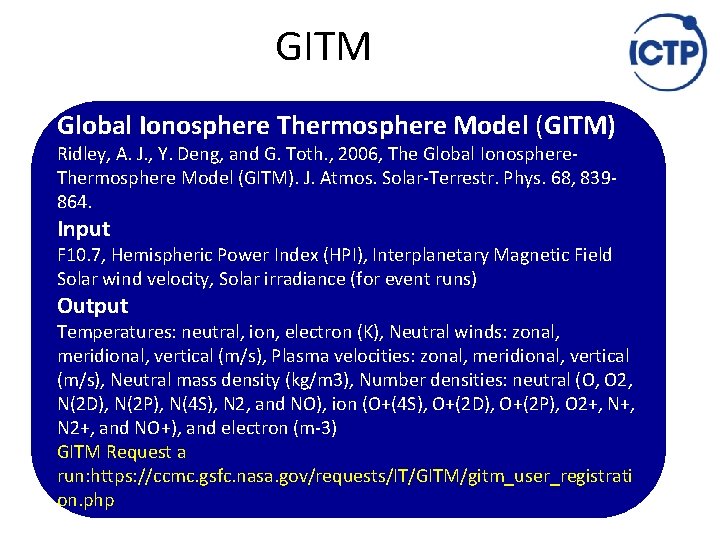
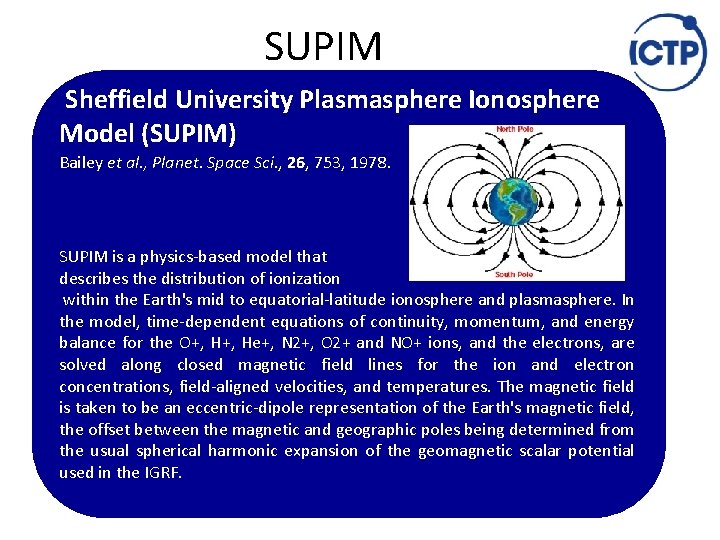
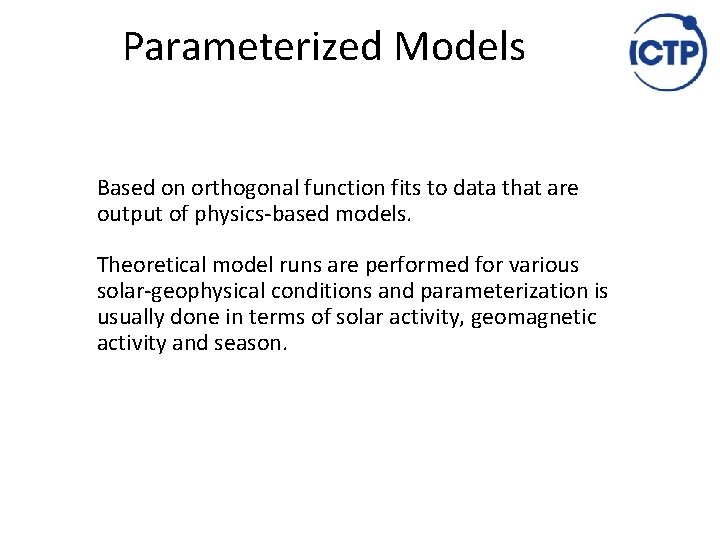
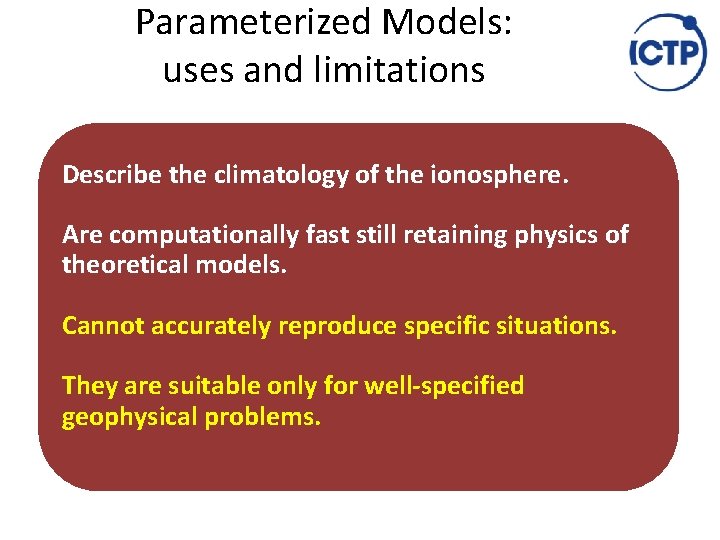
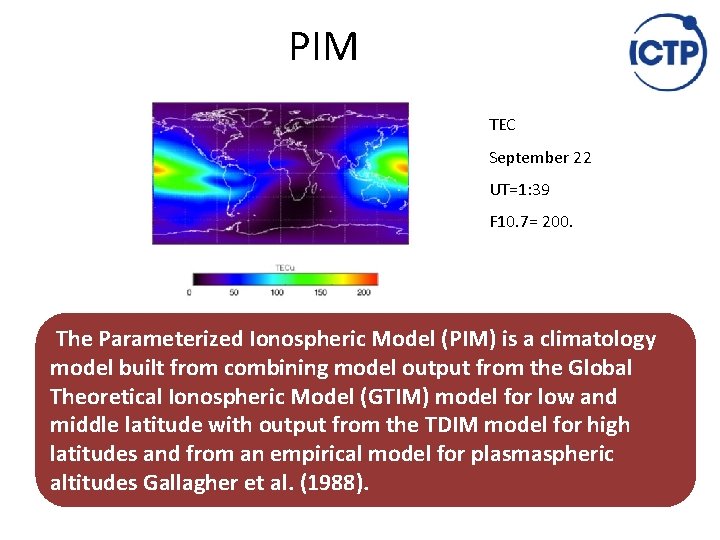
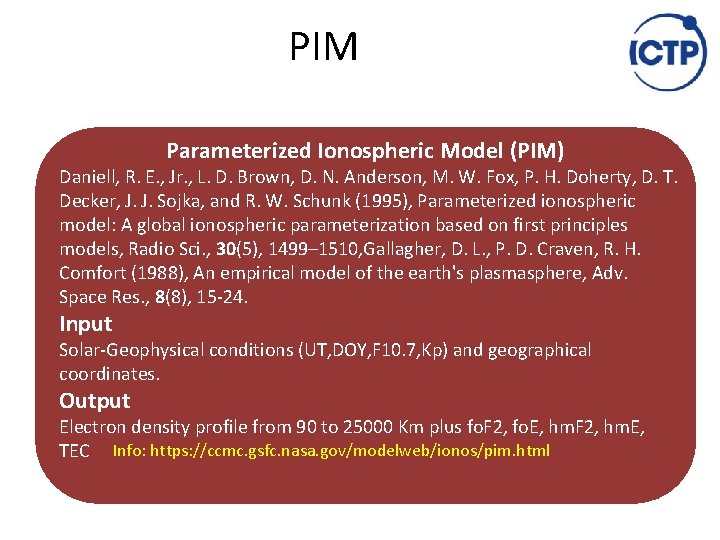
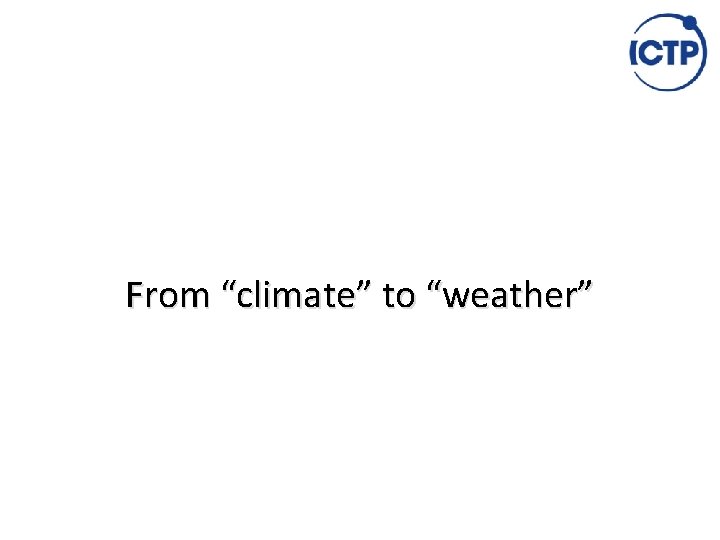
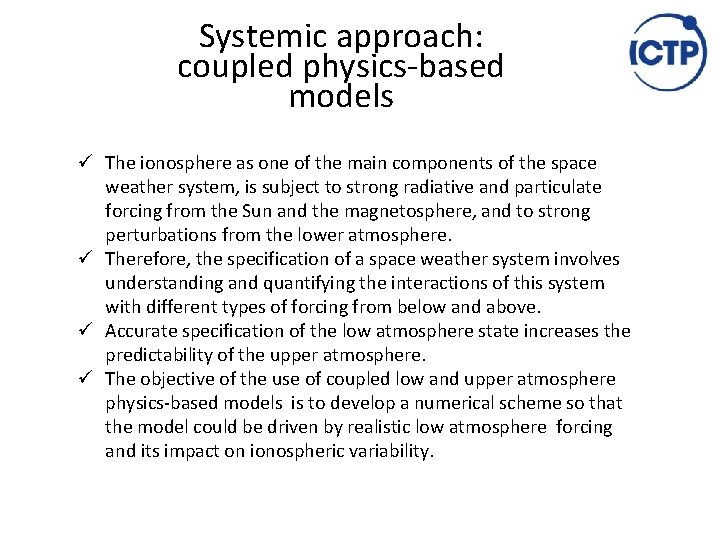
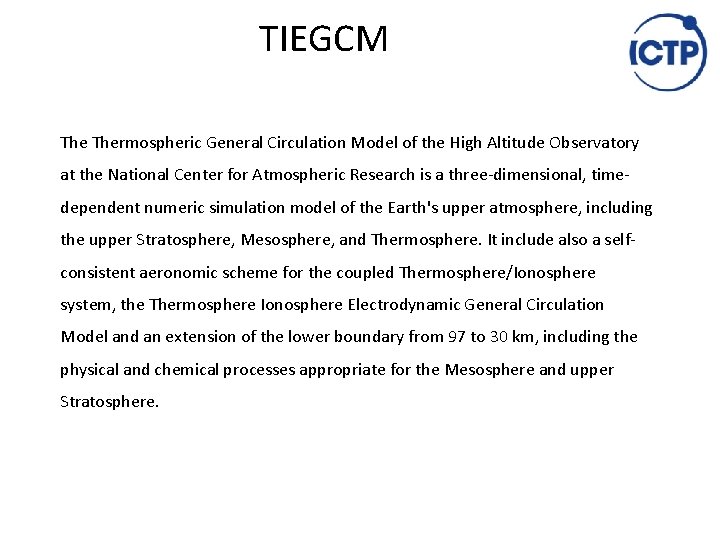
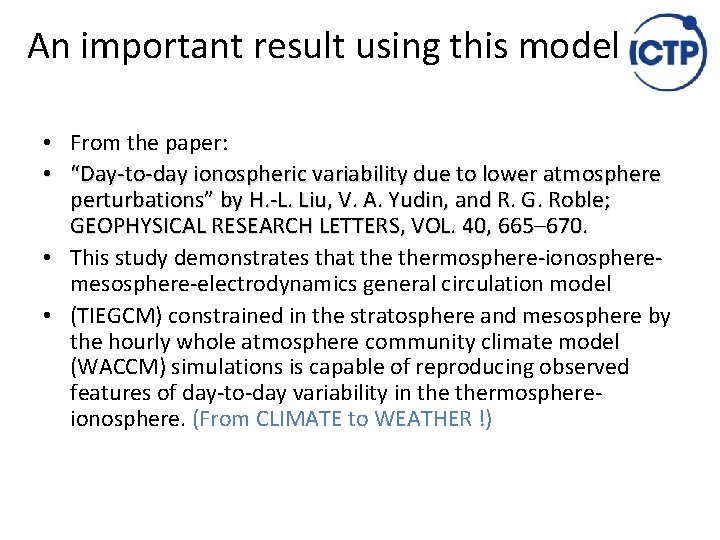
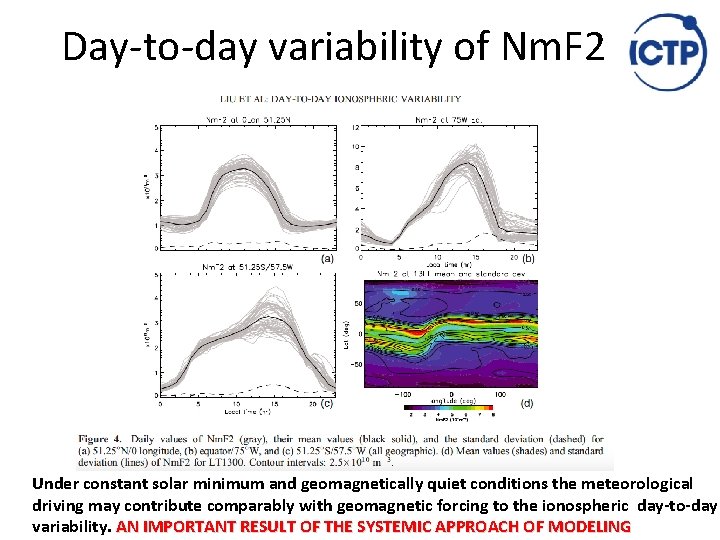
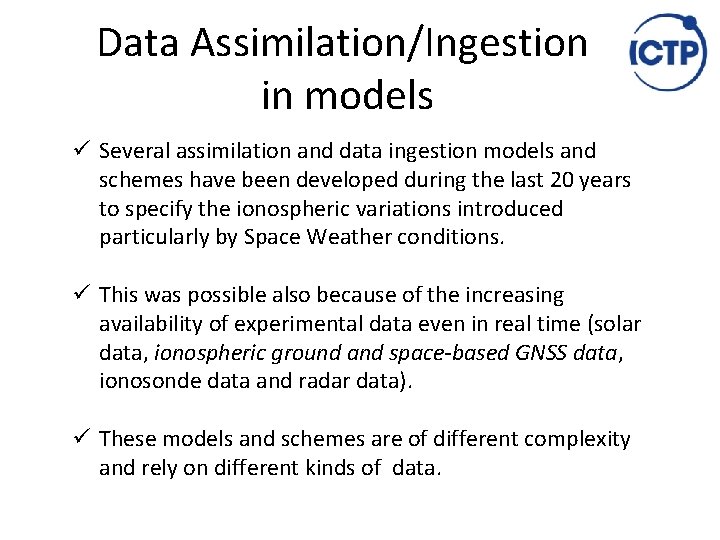
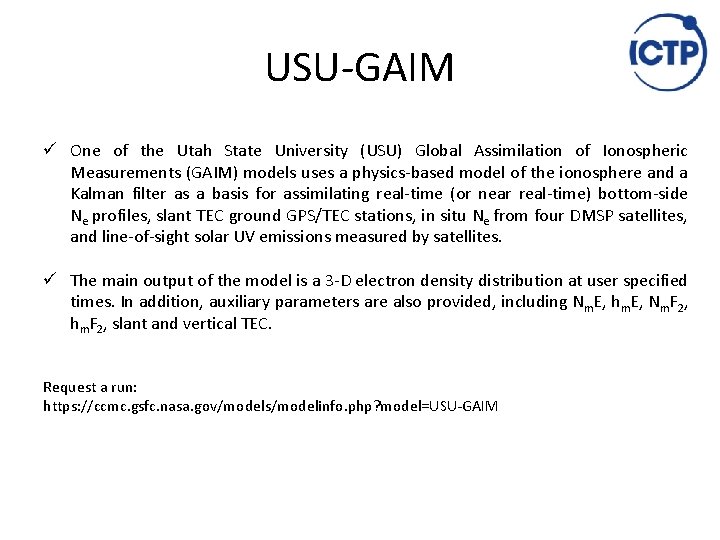
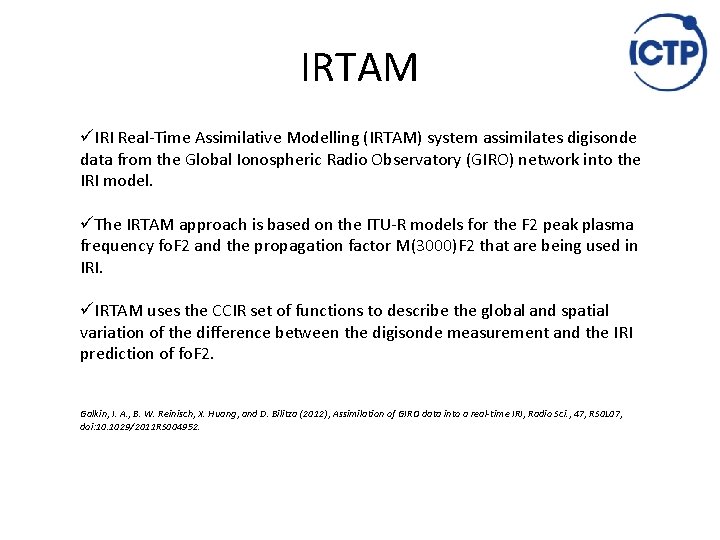
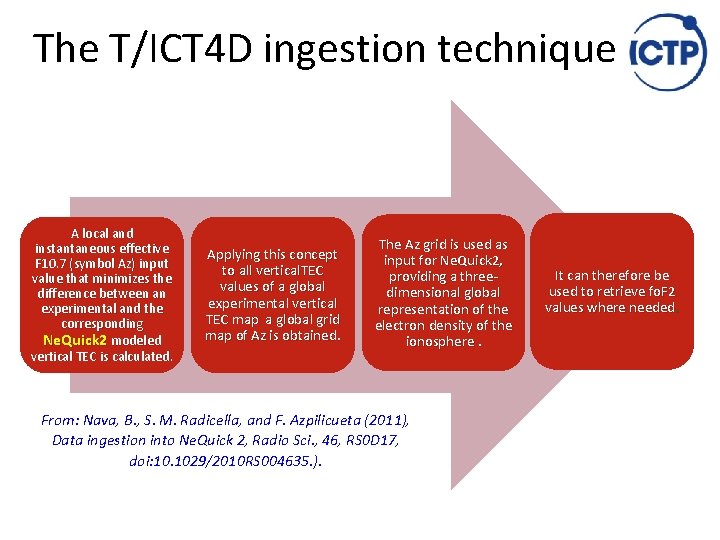
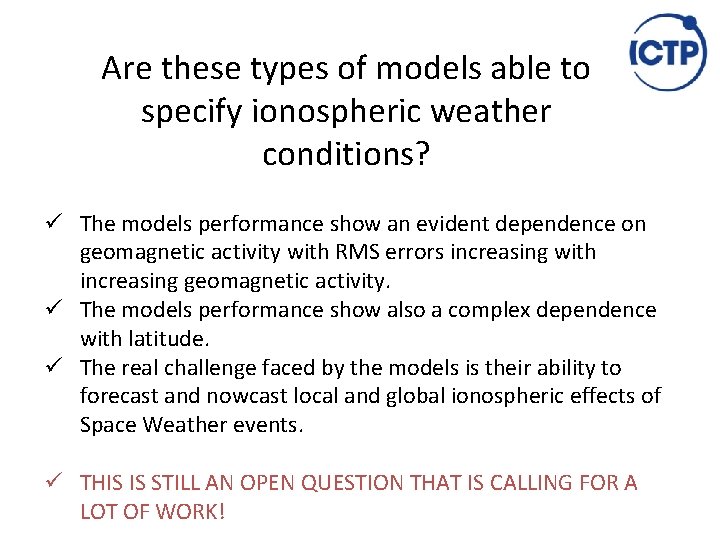
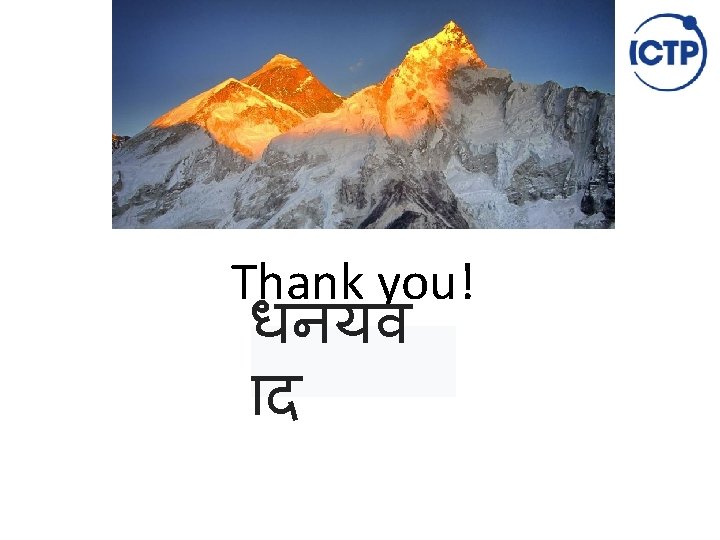
- Slides: 55
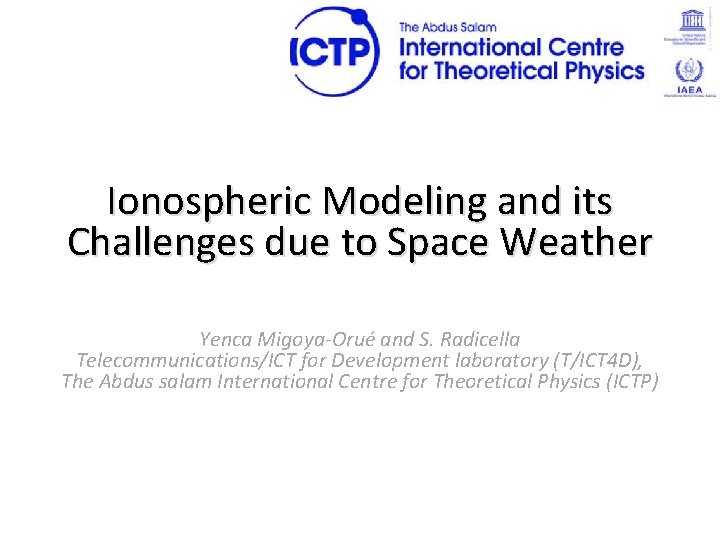
Ionospheric Modeling and its Challenges due to Space Weather Yenca Migoya-Orué and S. Radicella Telecommunications/ICT for Development laboratory (T/ICT 4 D), The Abdus salam International Centre for Theoretical Physics (ICTP)
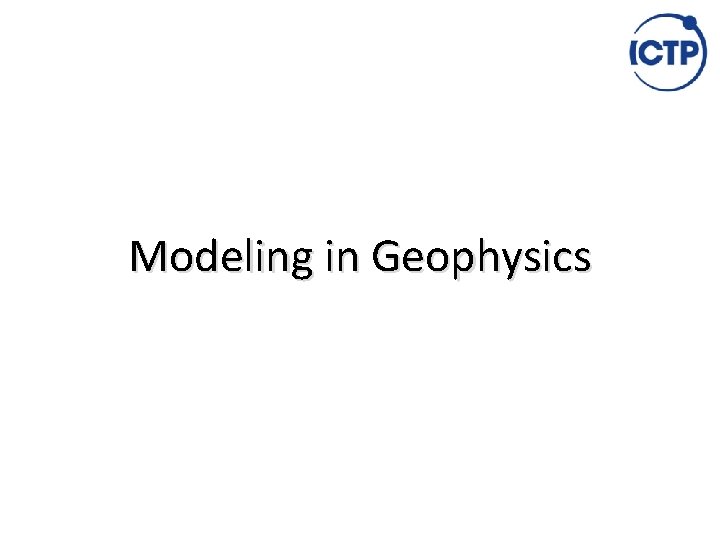
Modeling in Geophysics
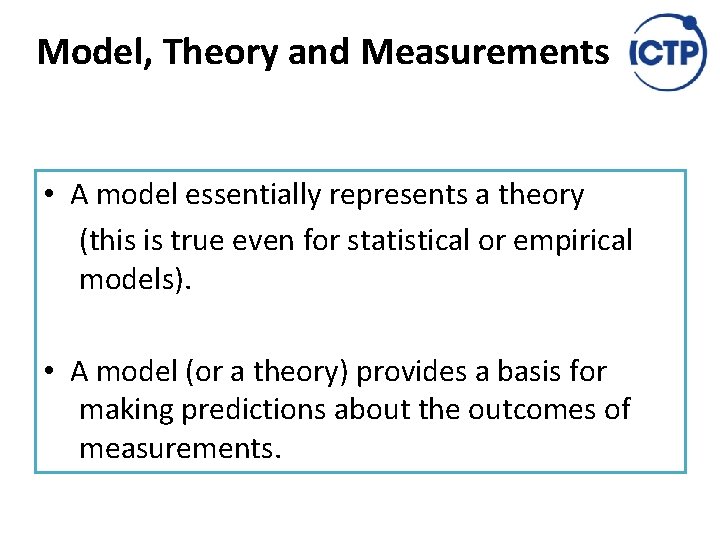
Model, Theory and Measurements • A model essentially represents a theory (this is true even for statistical or empirical models). • A model (or a theory) provides a basis for making predictions about the outcomes of measurements.
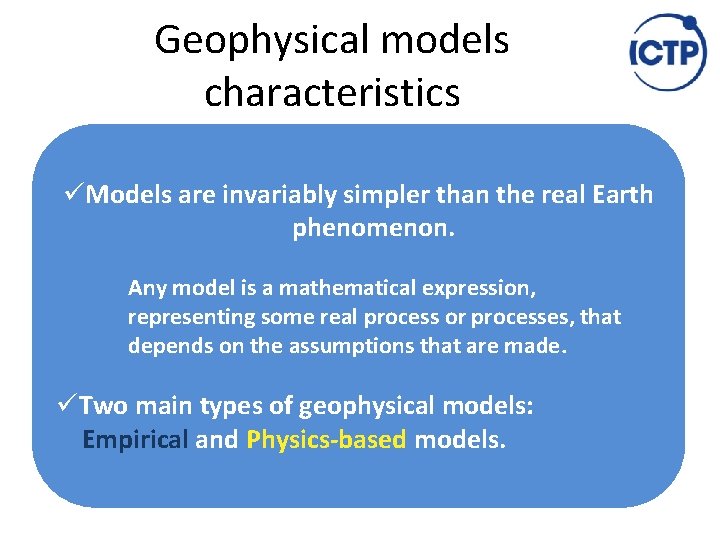
Geophysical models characteristics üModels are invariably simpler than the real Earth phenomenon. Any model is a mathematical expression, representing some real process or processes, that depends on the assumptions that are made. üTwo main types of geophysical models: Empirical and Physics-based models.
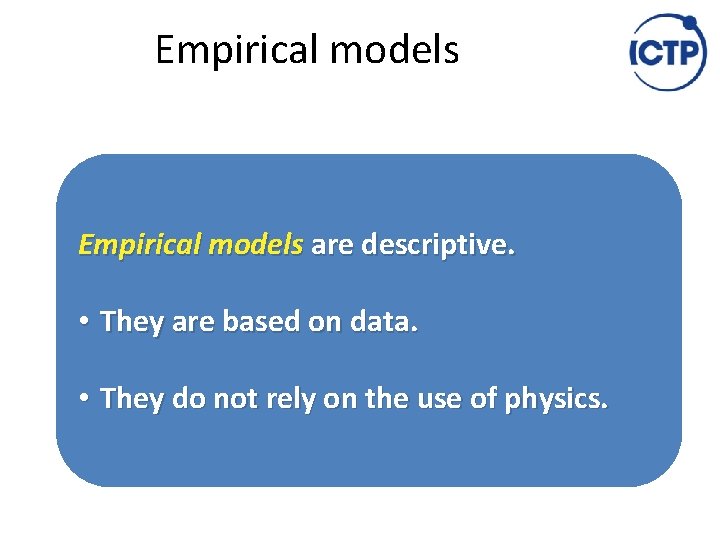
Empirical models are descriptive. • They are based on data. • They do not rely on the use of physics.
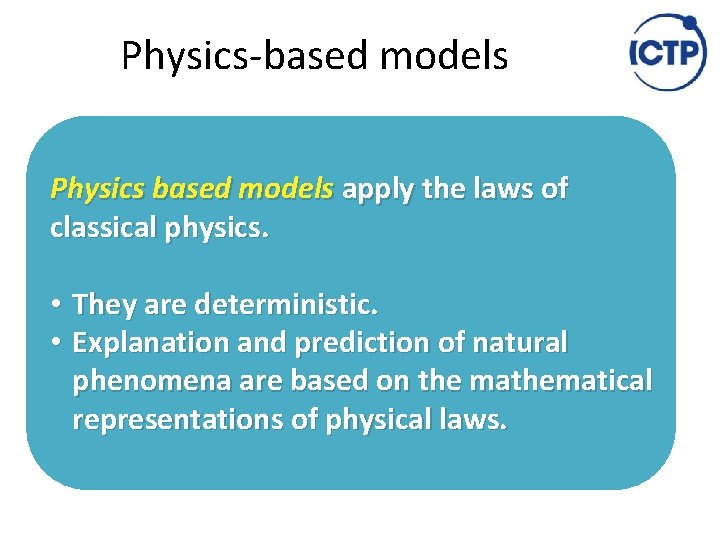
Physics‐based models Physics based models apply the laws of classical physics. • They are deterministic. • Explanation and prediction of natural phenomena are based on the mathematical representations of physical laws.
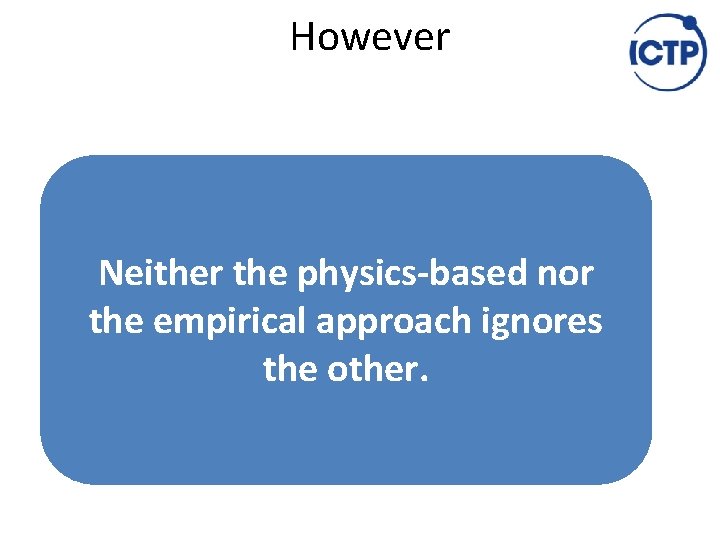
However Neither the physics-based nor the empirical approach ignores the other.
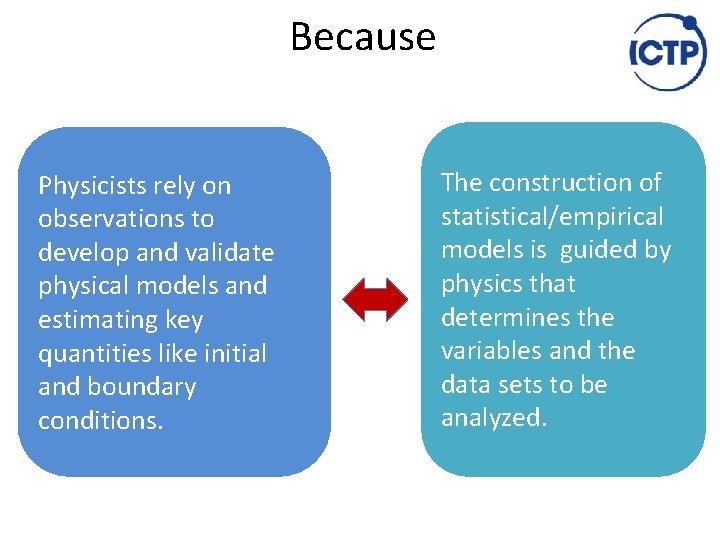
Because Physicists rely on observations to develop and validate physical models and estimating key quantities like initial and boundary conditions. The construction of statistical/empirical models is guided by physics that determines the variables and the data sets to be analyzed.
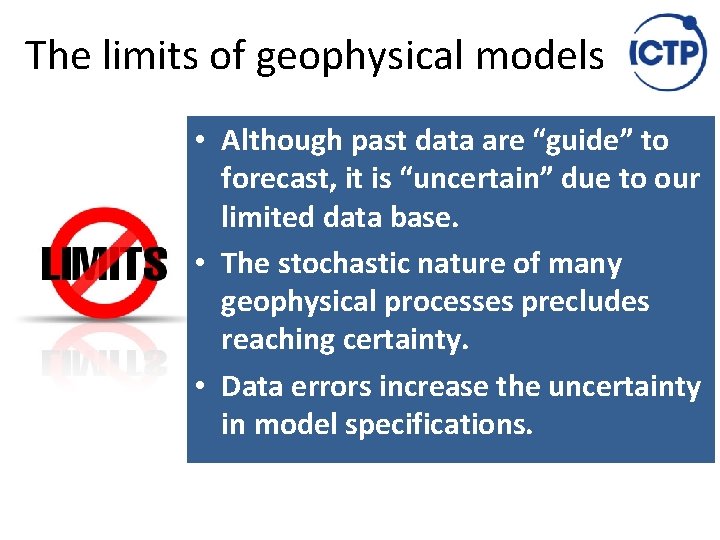
The limits of geophysical models • Although past data are “guide” to forecast, it is “uncertain” due to our limited data base. • The stochastic nature of many geophysical processes precludes reaching certainty. • Data errors increase the uncertainty in model specifications.
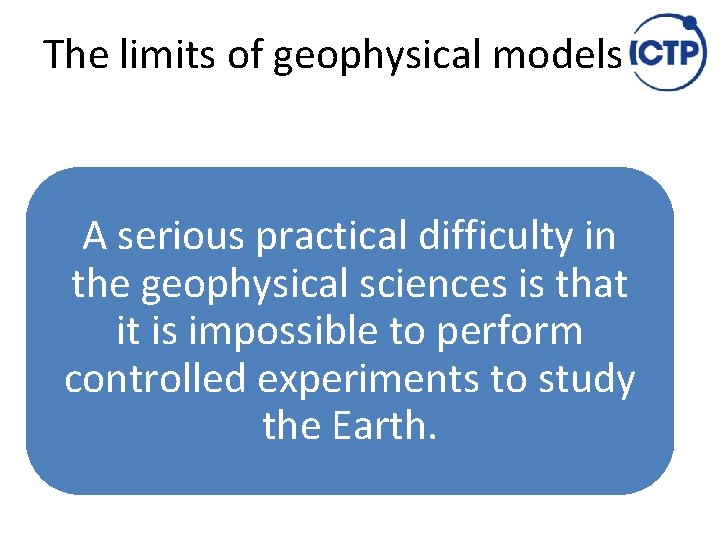
The limits of geophysical models A serious practical difficulty in the geophysical sciences is that it is impossible to perform controlled experiments to study the Earth.
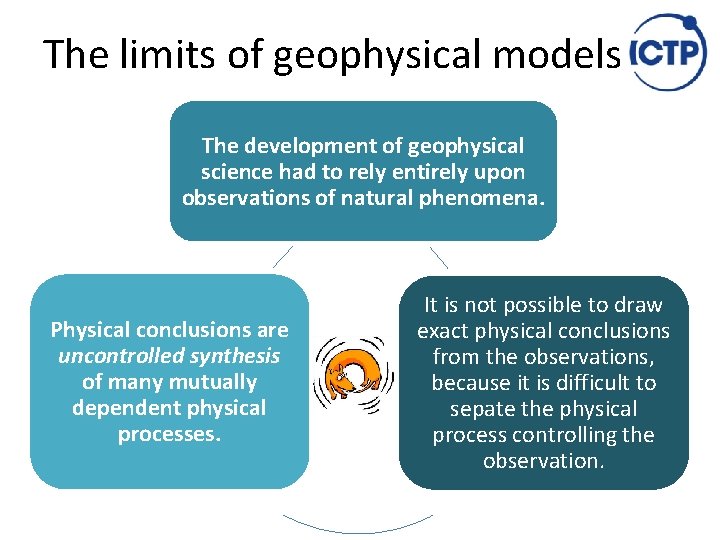
The limits of geophysical models The development of geophysical science had to rely entirely upon observations of natural phenomena. Physical conclusions are uncontrolled synthesis of many mutually dependent physical processes. It is not possible to draw exact physical conclusions from the observations, because it is difficult to sepate the physical process controlling the observation.
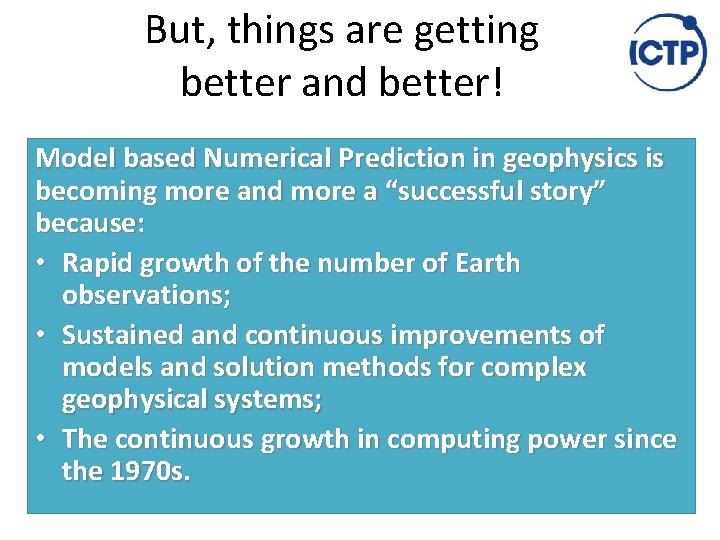
But, things are getting better and better! Model based Numerical Prediction in geophysics is becoming more and more a “successful story” because: • Rapid growth of the number of Earth observations; • Sustained and continuous improvements of models and solution methods for complex geophysical systems; • The continuous growth in computing power since the 1970 s.
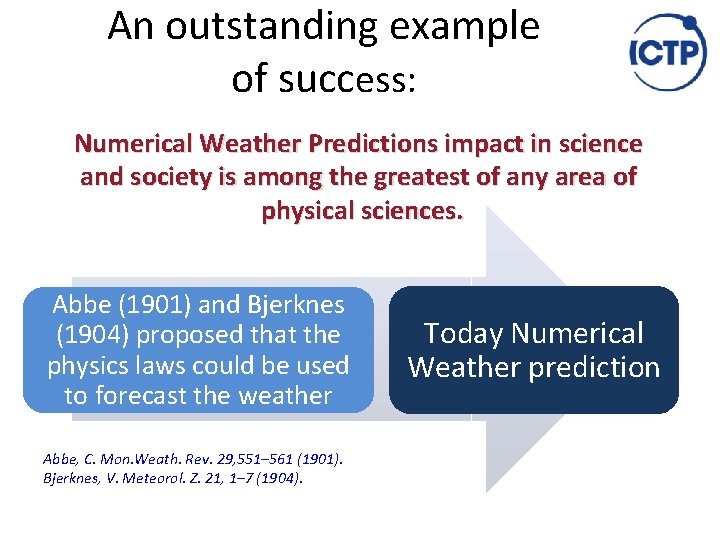
An outstanding example of success: Numerical Weather Predictions impact in science and society is among the greatest of any area of physical sciences. Abbe (1901) and Bjerknes (1904) proposed that the physics laws could be used to forecast the weather Abbe, C. Mon. Weath. Rev. 29, 551– 561 (1901). Bjerknes, V. Meteorol. Z. 21, 1– 7 (1904). Today Numerical Weather prediction
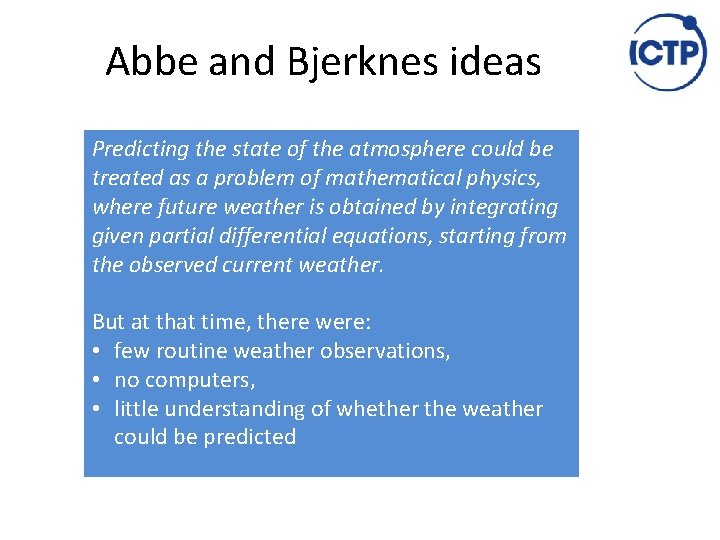
Abbe and Bjerknes ideas Predicting the state of the atmosphere could be treated as a problem of mathematical physics, where future weather is obtained by integrating given partial differential equations, starting from the observed current weather. But at that time, there were: • few routine weather observations, • no computers, • little understanding of whether the weather could be predicted
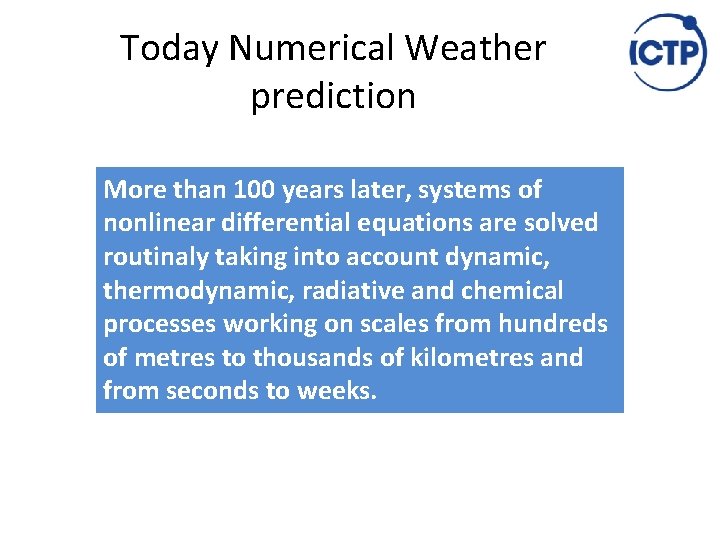
Today Numerical Weather prediction More than 100 years later, systems of nonlinear differential equations are solved routinaly taking into account dynamic, thermodynamic, radiative and chemical processes working on scales from hundreds of metres to thousands of kilometres and from seconds to weeks.
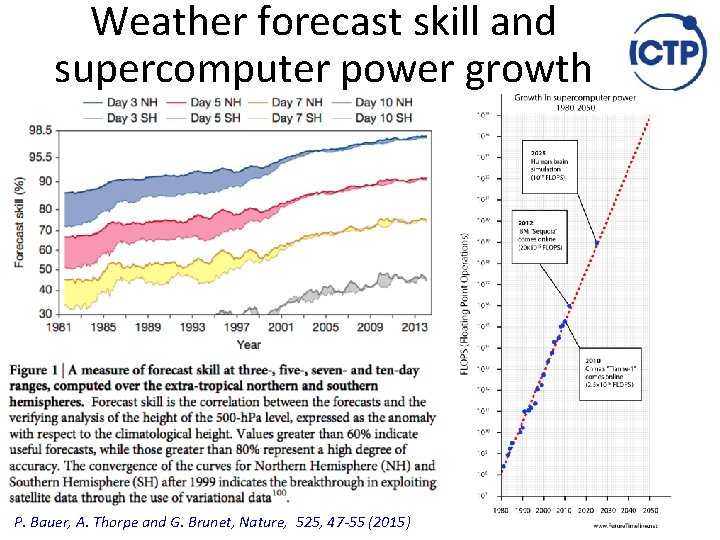
Weather forecast skill and supercomputer power growth P. Bauer, A. Thorpe and G. Brunet, Nature, 525, 47 -55 (2015)
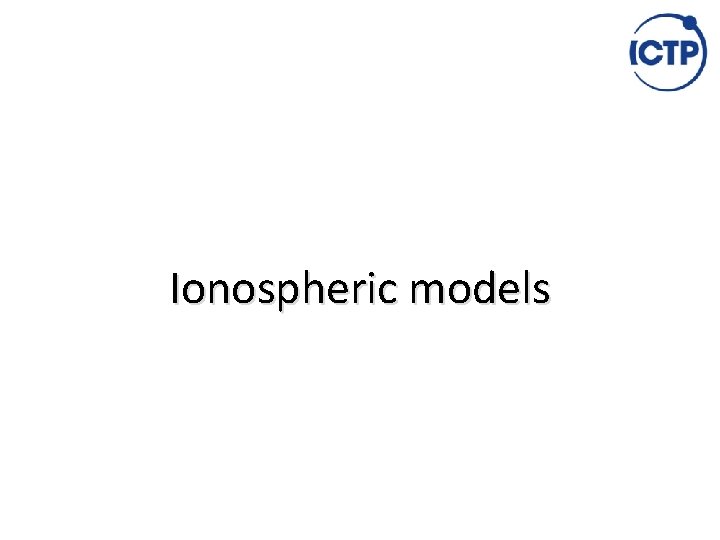
Ionospheric models
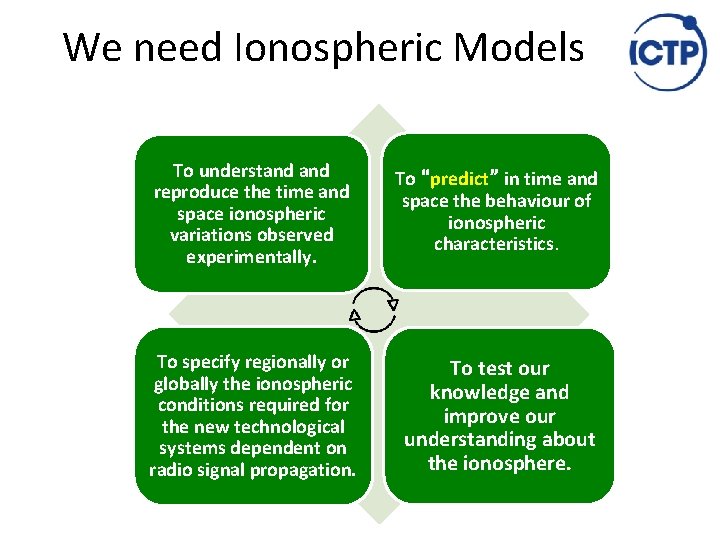
We need Ionospheric Models To understand reproduce the time and space ionospheric variations observed experimentally. To “predict” in time and space the behaviour of ionospheric characteristics. To specify regionally or globally the ionospheric conditions required for the new technological systems dependent on radio signal propagation. To test our knowledge and improve our understanding about the ionosphere.
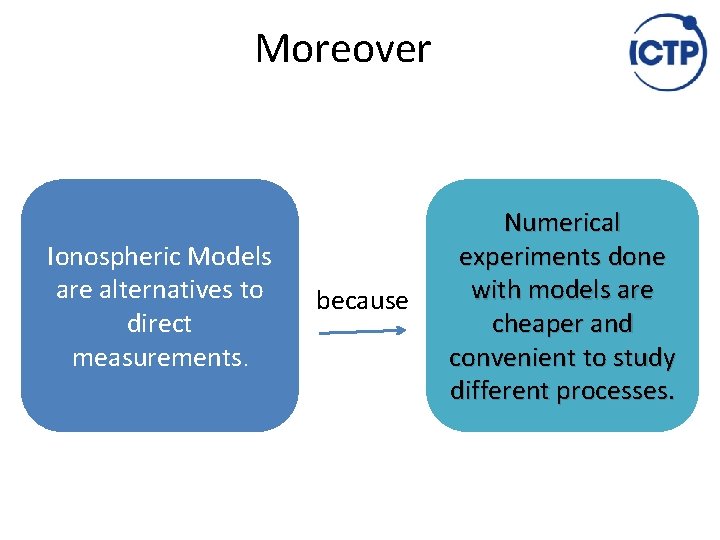
Moreover Ionospheric Models are alternatives to direct measurements. because Numerical experiments done with models are cheaper and convenient to study different processes.
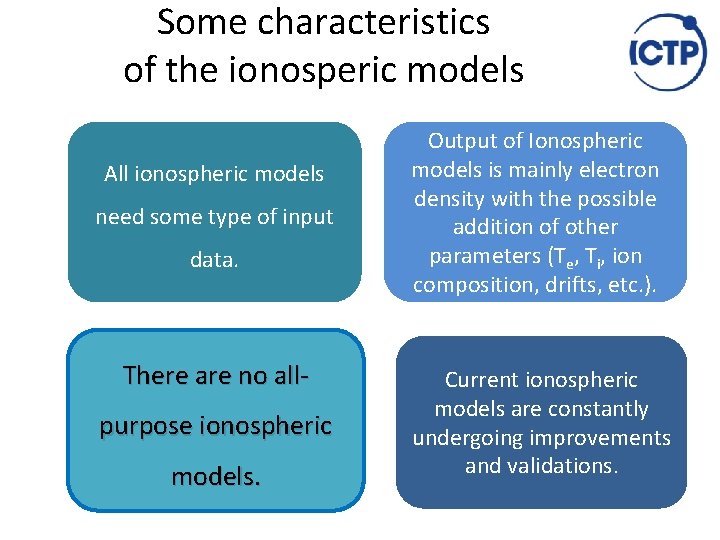
Some characteristics of the ionosperic models All ionospheric models need some type of input data. There are no all‐ purpose ionospheric models. Output of Ionospheric models is mainly electron density with the possible addition of other parameters (Te, Ti, ion composition, drifts, etc. ). Current ionospheric models are constantly undergoing improvements and validations.
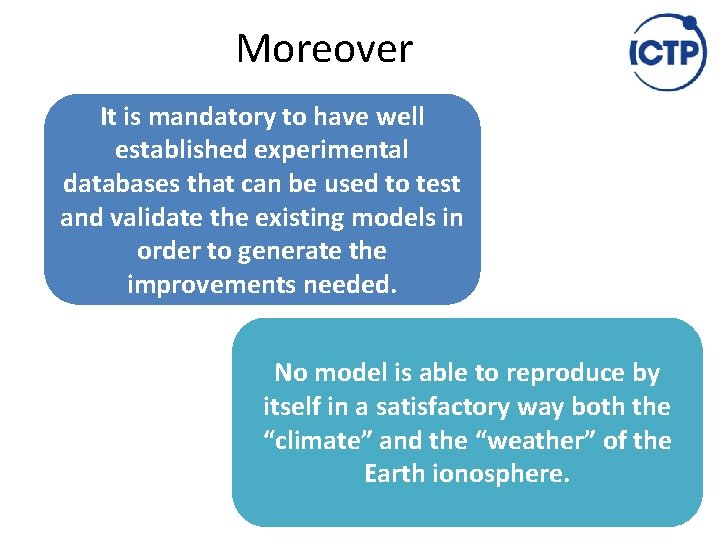
Moreover It is mandatory to have well established experimental databases that can be used to test and validate the existing models in order to generate the improvements needed. No model is able to reproduce by itself in a satisfactory way both the “climate” and the “weather” of the Earth ionosphere.
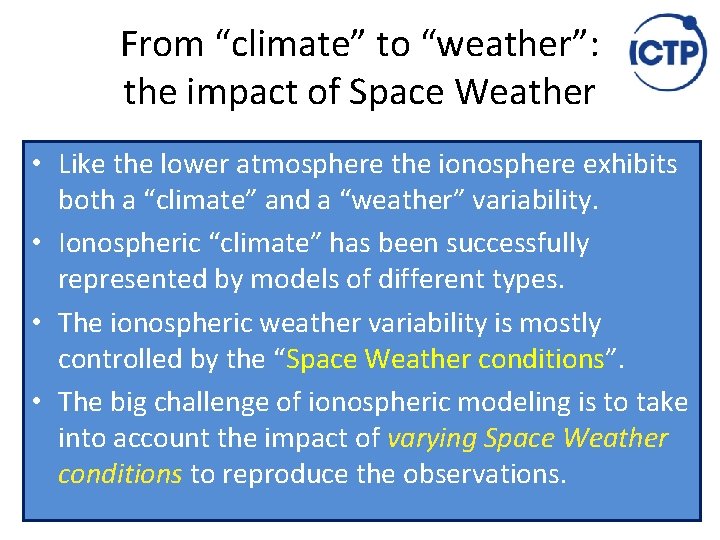
From “climate” to “weather”: the impact of Space Weather • Like the lower atmosphere the ionosphere exhibits both a “climate” and a “weather” variability. • Ionospheric “climate” has been successfully represented by models of different types. • The ionospheric weather variability is mostly controlled by the “Space Weather conditions”. • The big challenge of ionospheric modeling is to take into account the impact of varying Space Weather conditions to reproduce the observations.
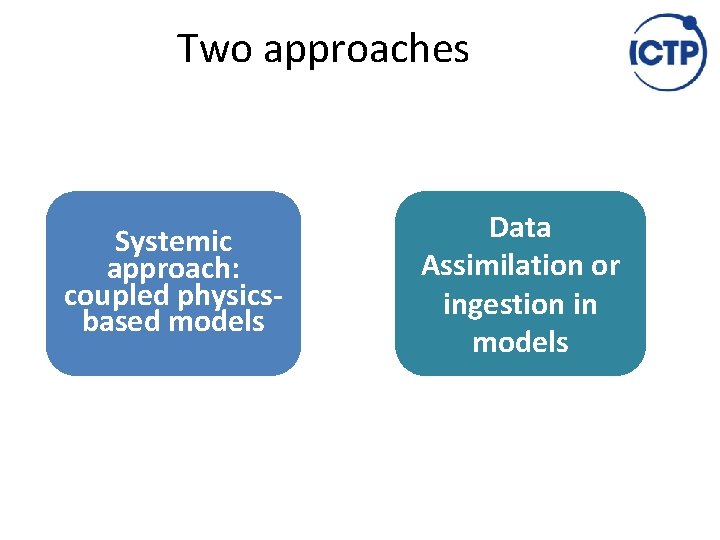
Two approaches Systemic approach: coupled physicsbased models Data Assimilation or ingestion in models
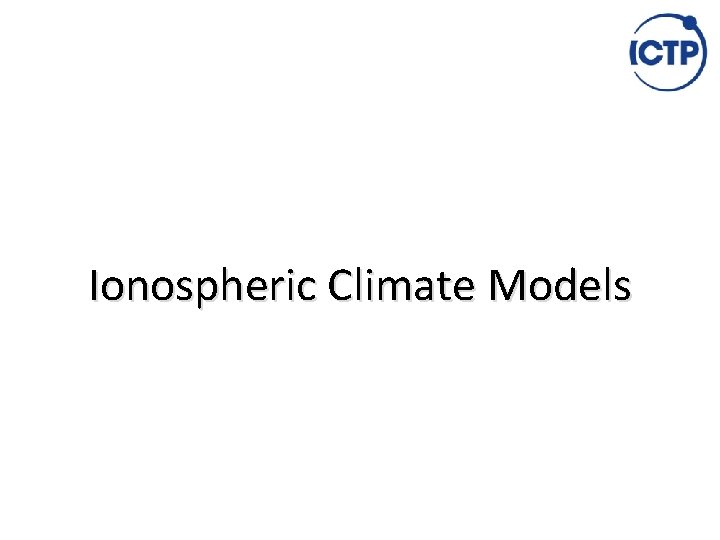
Ionospheric Climate Models
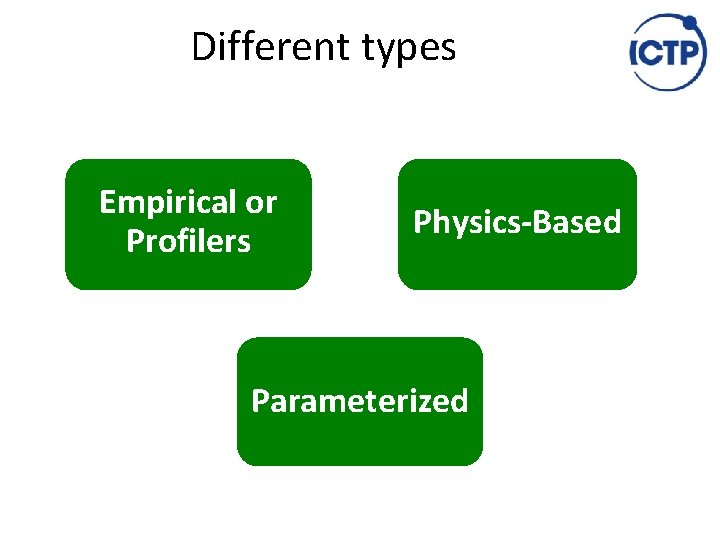
Different types Empirical or Profilers Physics-Based Parameterized
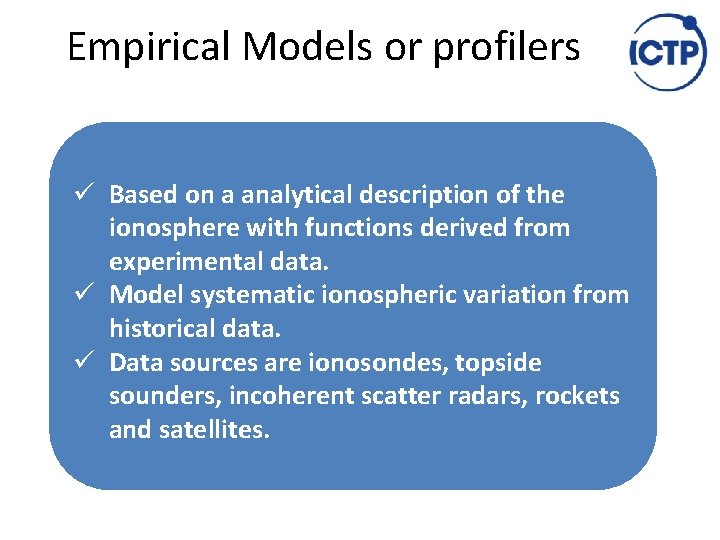
Empirical Models or profilers ü Based on a analytical description of the ionosphere with functions derived from experimental data. ü Model systematic ionospheric variation from historical data. ü Data sources are ionosondes, topside sounders, incoherent scatter radars, rockets and satellites.
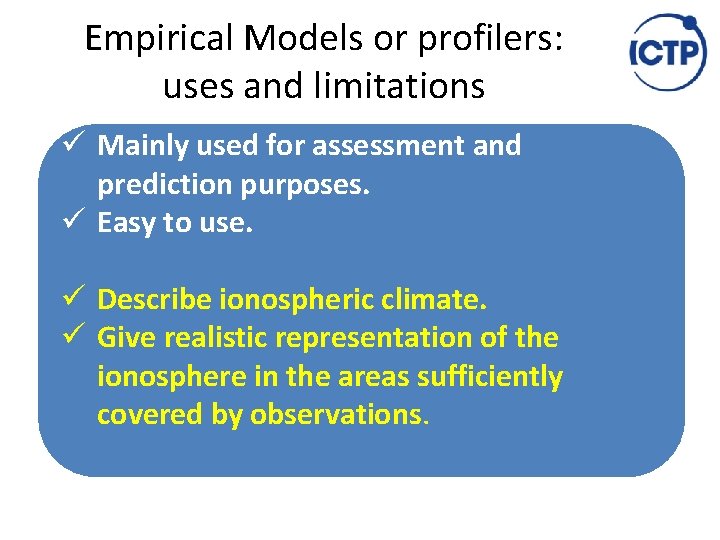
Empirical Models or profilers: uses and limitations ü Mainly used for assessment and prediction purposes. ü Easy to use. ü Describe ionospheric climate. ü Give realistic representation of the ionosphere in the areas sufficiently covered by observations.
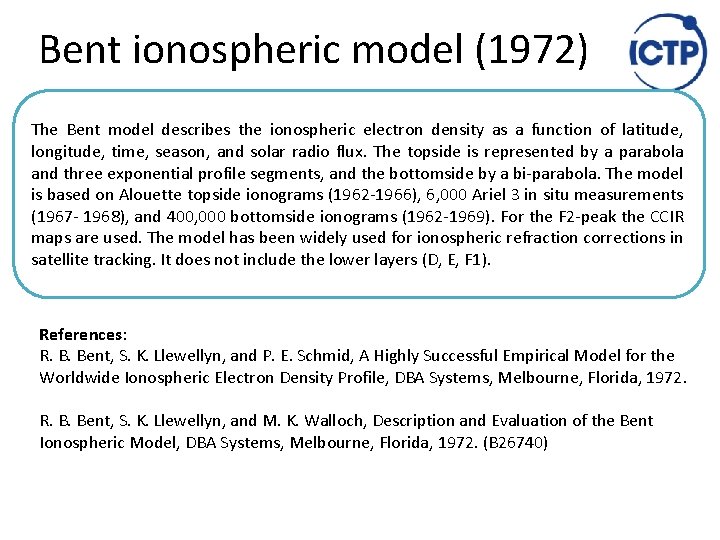
Bent ionospheric model (1972) The Bent model describes the ionospheric electron density as a function of latitude, longitude, time, season, and solar radio flux. The topside is represented by a parabola and three exponential profile segments, and the bottomside by a bi‐parabola. The model is based on Alouette topside ionograms (1962‐ 1966), 6, 000 Ariel 3 in situ measurements (1967‐ 1968), and 400, 000 bottomside ionograms (1962‐ 1969). For the F 2‐peak the CCIR maps are used. The model has been widely used for ionospheric refraction corrections in satellite tracking. It does not include the lower layers (D, E, F 1). References: R. B. Bent, S. K. Llewellyn, and P. E. Schmid, A Highly Successful Empirical Model for the Worldwide Ionospheric Electron Density Profile, DBA Systems, Melbourne, Florida, 1972. R. B. Bent, S. K. Llewellyn, and M. K. Walloch, Description and Evaluation of the Bent Ionospheric Model, DBA Systems, Melbourne, Florida, 1972. (B 26740)
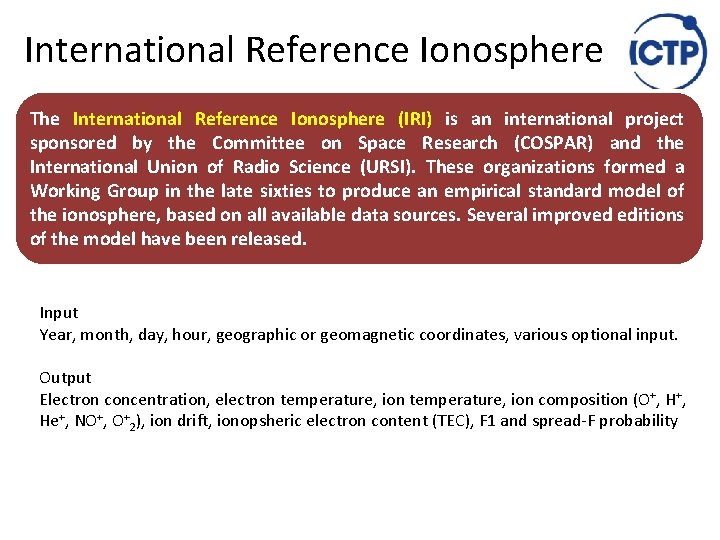
International Reference Ionosphere The International Reference Ionosphere (IRI) is an international project sponsored by the Committee on Space Research (COSPAR) and the International Union of Radio Science (URSI). These organizations formed a Working Group in the late sixties to produce an empirical standard model of the ionosphere, based on all available data sources. Several improved editions of the model have been released. Input Year, month, day, hour, geographic or geomagnetic coordinates, various optional input. Output Electron concentration, electron temperature, ion composition (O+, He+, NO+, O+2), ion drift, ionopsheric electron content (TEC), F 1 and spread‐F probability
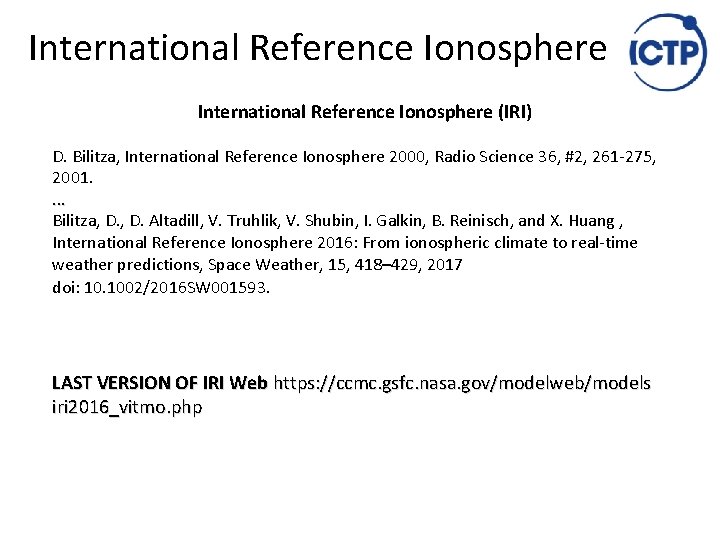
International Reference Ionosphere (IRI) D. Bilitza, International Reference Ionosphere 2000, Radio Science 36, #2, 261‐ 275, 2001. . Bilitza, D. Altadill, V. Truhlik, V. Shubin, I. Galkin, B. Reinisch, and X. Huang , International Reference Ionosphere 2016: From ionospheric climate to real‐time weather predictions, Space Weather, 15, 418– 429, 2017 doi: 10. 1002/2016 SW 001593. LAST VERSION OF IRI Web https: //ccmc. gsfc. nasa. gov/modelweb/models iri 2016_vitmo. php
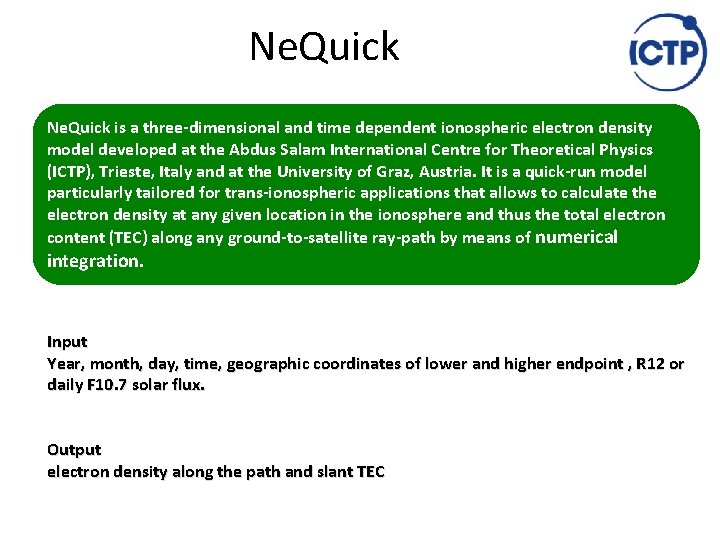
Ne. Quick is a three-dimensional and time dependent ionospheric electron density model developed at the Abdus Salam International Centre for Theoretical Physics (ICTP), Trieste, Italy and at the University of Graz, Austria. It is a quick-run model particularly tailored for trans-ionospheric applications that allows to calculate the electron density at any given location in the ionosphere and thus the total electron content (TEC) along any ground-to-satellite ray-path by means of numerical integration. Input Year, month, day, time, geographic coordinates of lower and higher endpoint , R 12 or daily F 10. 7 solar flux. Output electron density along the path and slant TEC
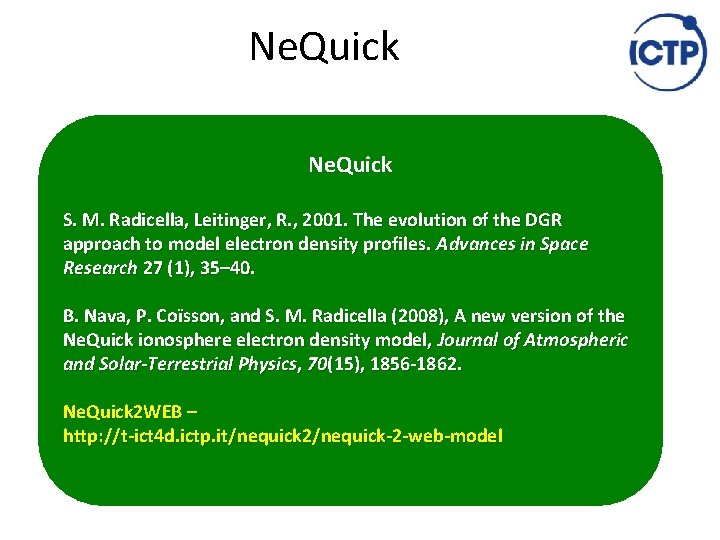
Ne. Quick S. M. Radicella, Leitinger, R. , 2001. The evolution of the DGR approach to model electron density profiles. Advances in Space Research 27 (1), 35– 40. B. Nava, P. Coïsson, and S. M. Radicella (2008), A new version of the Ne. Quick ionosphere electron density model, Journal of Atmospheric and Solar-Terrestrial Physics, 70(15), 1856 -1862. Ne. Quick 2 WEB – http: //t-ict 4 d. ictp. it/nequick 2/nequick-2 -web-model
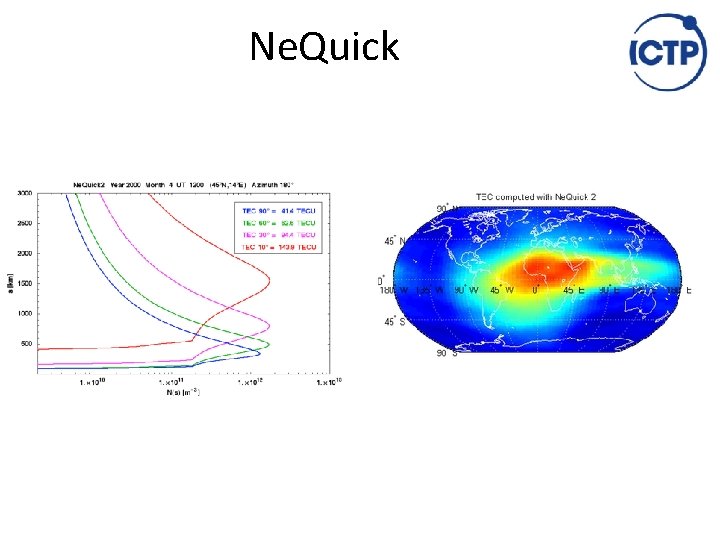
Ne. Quick
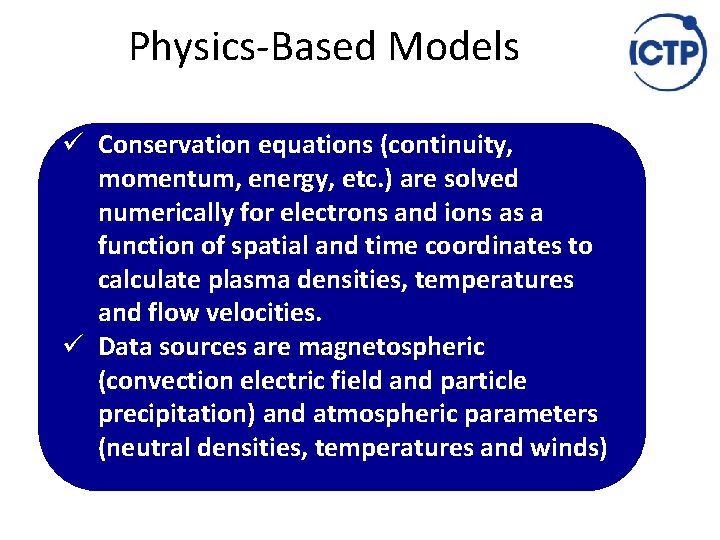
Physics‐Based Models ü Conservation equations (continuity, momentum, energy, etc. ) are solved numerically for electrons and ions as a function of spatial and time coordinates to calculate plasma densities, temperatures and flow velocities. ü Data sources are magnetospheric (convection electric field and particle precipitation) and atmospheric parameters (neutral densities, temperatures and winds)
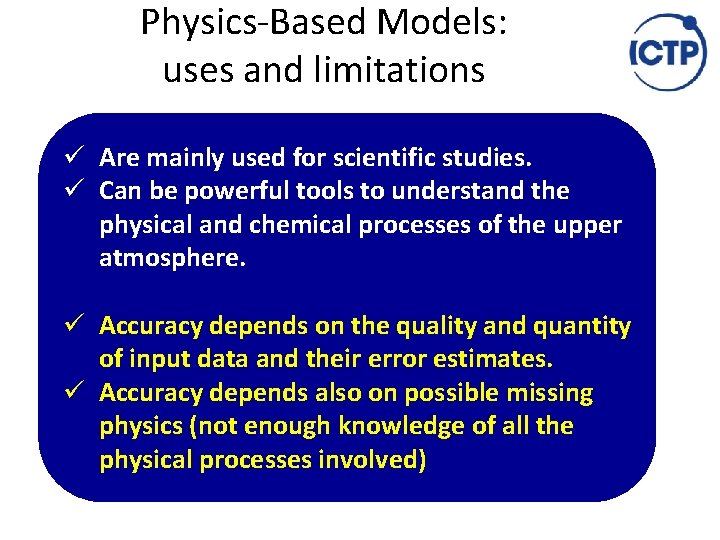
Physics‐Based Models: uses and limitations ü Are mainly used for scientific studies. ü Can be powerful tools to understand the physical and chemical processes of the upper atmosphere. ü Accuracy depends on the quality and quantity of input data and their error estimates. ü Accuracy depends also on possible missing physics (not enough knowledge of all the physical processes involved)
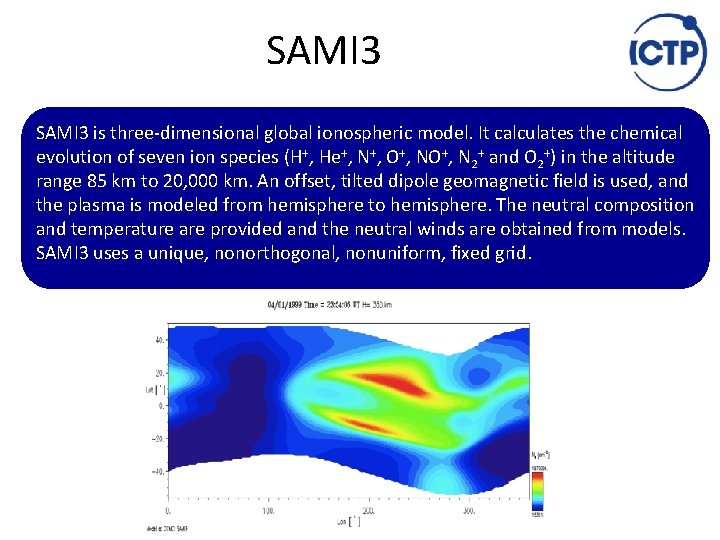
SAMI 3 is three‐dimensional global ionospheric model. It calculates the chemical evolution of seven ion species (H+, He+, N+, O+, N 2+ and O 2+) in the altitude range 85 km to 20, 000 km. An offset, tilted dipole geomagnetic field is used, and the plasma is modeled from hemisphere to hemisphere. The neutral composition and temperature are provided and the neutral winds are obtained from models. SAMI 3 uses a unique, nonorthogonal, nonuniform, fixed grid.
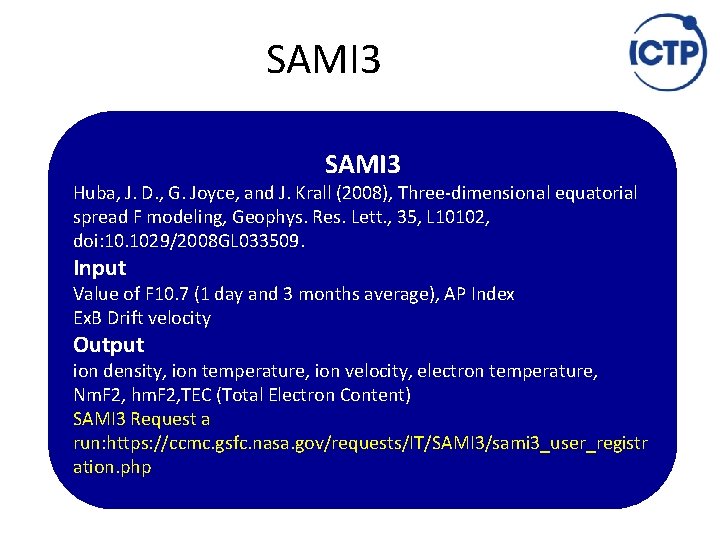
SAMI 3 Huba, J. D. , G. Joyce, and J. Krall (2008), Three‐dimensional equatorial spread F modeling, Geophys. Res. Lett. , 35, L 10102, doi: 10. 1029/2008 GL 033509. Input Value of F 10. 7 (1 day and 3 months average), AP Index Ex. B Drift velocity Output ion density, ion temperature, ion velocity, electron temperature, Nm. F 2, hm. F 2, TEC (Total Electron Content) SAMI 3 Request a run: https: //ccmc. gsfc. nasa. gov/requests/IT/SAMI 3/sami 3_user_registr ation. php
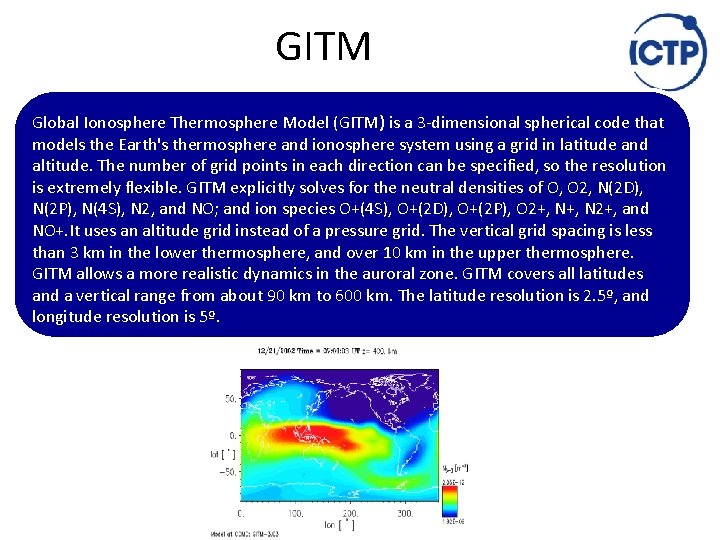
GITM Global Ionosphere Thermosphere Model (GITM) is a 3‐dimensional spherical code that models the Earth's thermosphere and ionosphere system using a grid in latitude and altitude. The number of grid points in each direction can be specified, so the resolution is extremely flexible. GITM explicitly solves for the neutral densities of O, O 2, N(2 D), N(2 P), N(4 S), N 2, and NO; and ion species O+(4 S), O+(2 D), O+(2 P), O 2+, N 2+, and NO+. It uses an altitude grid instead of a pressure grid. The vertical grid spacing is less than 3 km in the lower thermosphere, and over 10 km in the upper thermosphere. GITM allows a more realistic dynamics in the auroral zone. GITM covers all latitudes and a vertical range from about 90 km to 600 km. The latitude resolution is 2. 5º, and longitude resolution is 5º.
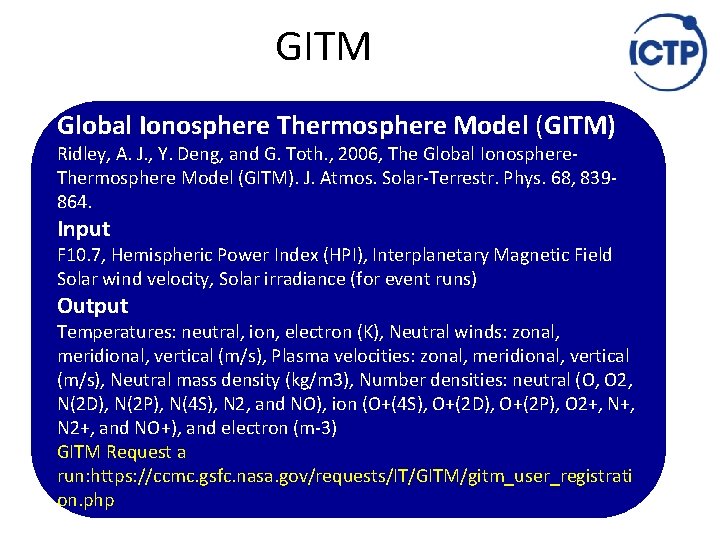
GITM Global Ionosphere Thermosphere Model (GITM) Ridley, A. J. , Y. Deng, and G. Toth. , 2006, The Global Ionosphere‐ Thermosphere Model (GITM). J. Atmos. Solar‐Terrestr. Phys. 68, 839‐ 864. Input F 10. 7, Hemispheric Power Index (HPI), Interplanetary Magnetic Field Solar wind velocity, Solar irradiance (for event runs) Output Temperatures: neutral, ion, electron (K), Neutral winds: zonal, meridional, vertical (m/s), Plasma velocities: zonal, meridional, vertical (m/s), Neutral mass density (kg/m 3), Number densities: neutral (O, O 2, N(2 D), N(2 P), N(4 S), N 2, and NO), ion (O+(4 S), O+(2 D), O+(2 P), O 2+, N 2+, and NO+), and electron (m‐ 3) GITM Request a run: https: //ccmc. gsfc. nasa. gov/requests/IT/GITM/gitm_user_registrati on. php
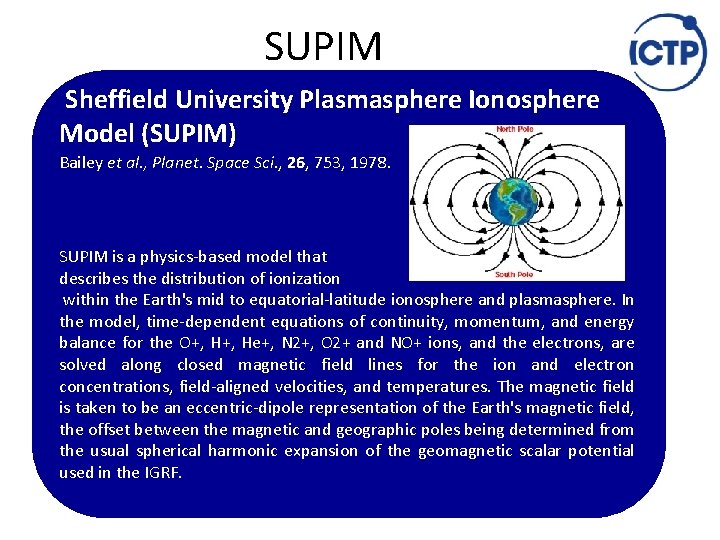
SUPIM Sheffield University Plasmasphere Ionosphere Model (SUPIM) Bailey et al. , Planet. Space Sci. , 26, 753, 1978. SUPIM is a physics‐based model that describes the distribution of ionization within the Earth's mid to equatorial‐latitude ionosphere and plasmasphere. In the model, time‐dependent equations of continuity, momentum, and energy balance for the O+, He+, N 2+, O 2+ and NO+ ions, and the electrons, are solved along closed magnetic field lines for the ion and electron concentrations, field‐aligned velocities, and temperatures. The magnetic field is taken to be an eccentric‐dipole representation of the Earth's magnetic field, the offset between the magnetic and geographic poles being determined from the usual spherical harmonic expansion of the geomagnetic scalar potential used in the IGRF.
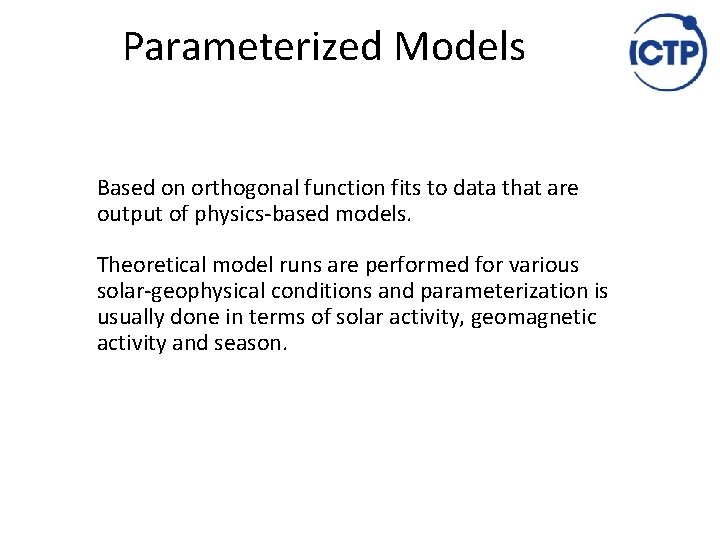
Parameterized Models Based on orthogonal function fits to data that are output of physics‐based models. Theoretical model runs are performed for various solar‐geophysical conditions and parameterization is usually done in terms of solar activity, geomagnetic activity and season.
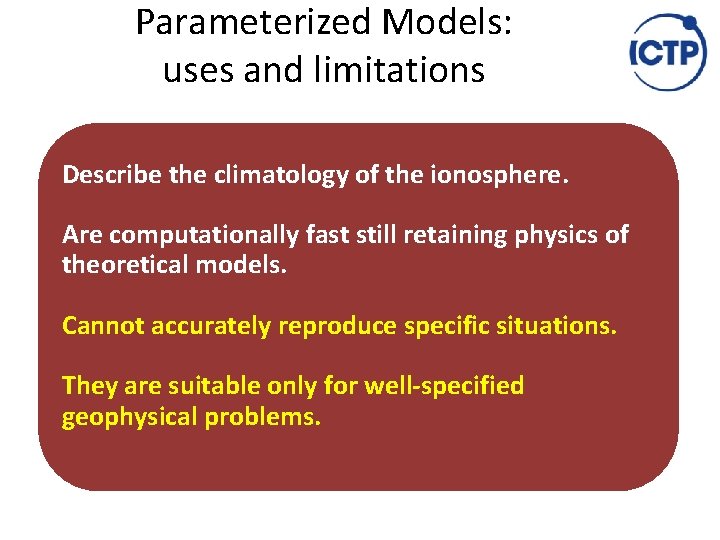
Parameterized Models: uses and limitations Describe the climatology of the ionosphere. Are computationally fast still retaining physics of theoretical models. Cannot accurately reproduce specific situations. They are suitable only for well-specified geophysical problems.
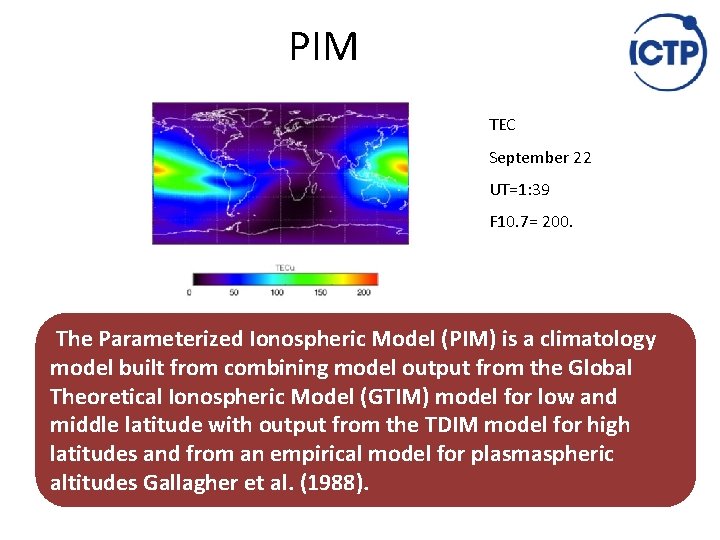
PIM TEC September 22 UT=1: 39 F 10. 7= 200. The Parameterized Ionospheric Model (PIM) is a climatology model built from combining model output from the Global Theoretical Ionospheric Model (GTIM) model for low and middle latitude with output from the TDIM model for high latitudes and from an empirical model for plasmaspheric altitudes Gallagher et al. (1988).
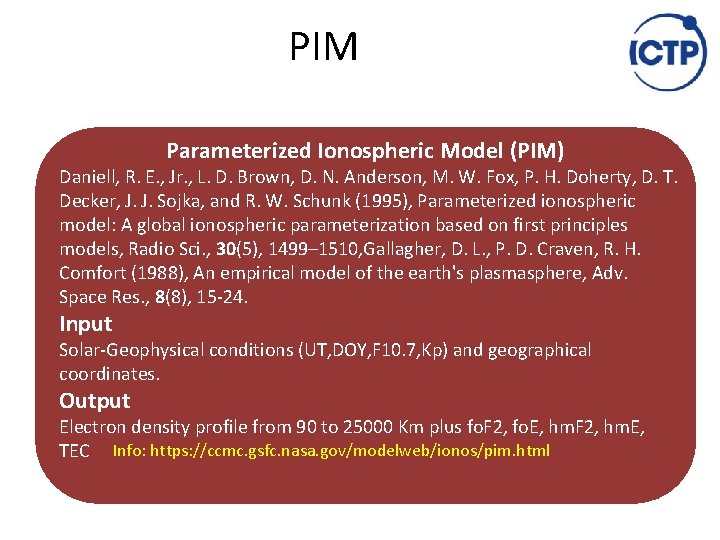
PIM Parameterized Ionospheric Model (PIM) Daniell, R. E. , Jr. , L. D. Brown, D. N. Anderson, M. W. Fox, P. H. Doherty, D. T. Decker, J. J. Sojka, and R. W. Schunk (1995), Parameterized ionospheric model: A global ionospheric parameterization based on first principles models, Radio Sci. , 30(5), 1499– 1510, Gallagher, D. L. , P. D. Craven, R. H. Comfort (1988), An empirical model of the earth's plasmasphere, Adv. Space Res. , 8(8), 15‐ 24. Input Solar‐Geophysical conditions (UT, DOY, F 10. 7, Kp) and geographical coordinates. Output Electron density profile from 90 to 25000 Km plus fo. F 2, fo. E, hm. F 2, hm. E, TEC Info: https: //ccmc. gsfc. nasa. gov/modelweb/ionos/pim. html
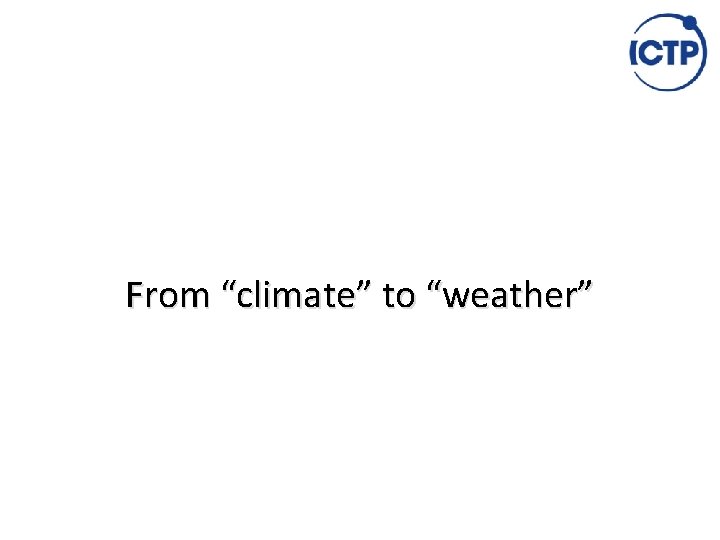
From “climate” to “weather”
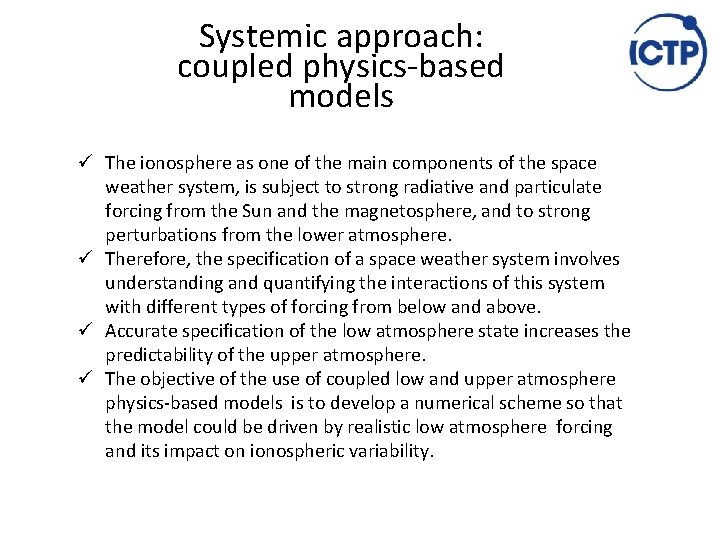
Systemic approach: coupled physics‐based models ü The ionosphere as one of the main components of the space weather system, is subject to strong radiative and particulate forcing from the Sun and the magnetosphere, and to strong perturbations from the lower atmosphere. ü Therefore, the specification of a space weather system involves understanding and quantifying the interactions of this system with different types of forcing from below and above. ü Accurate specification of the low atmosphere state increases the predictability of the upper atmosphere. ü The objective of the use of coupled low and upper atmosphere physics‐based models is to develop a numerical scheme so that the model could be driven by realistic low atmosphere forcing and its impact on ionospheric variability.
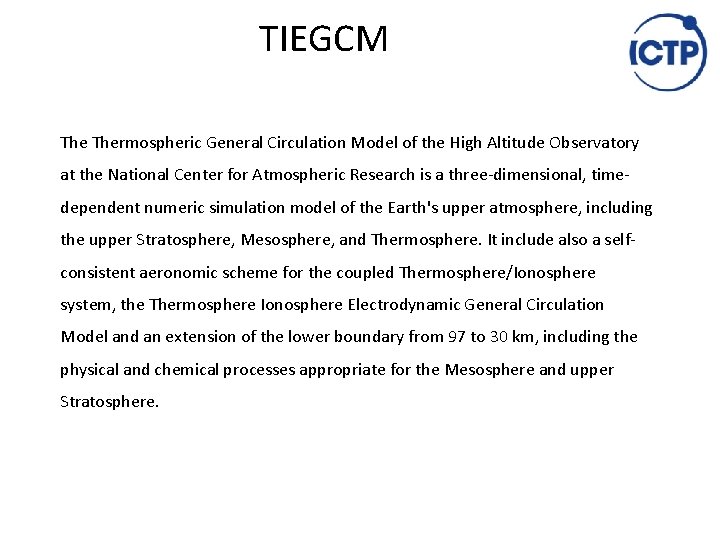
TIEGCM Thermospheric General Circulation Model of the High Altitude Observatory at the National Center for Atmospheric Research is a three‐dimensional, time‐ dependent numeric simulation model of the Earth's upper atmosphere, including the upper Stratosphere, Mesosphere, and Thermosphere. It include also a self‐ consistent aeronomic scheme for the coupled Thermosphere/Ionosphere system, the Thermosphere Ionosphere Electrodynamic General Circulation Model and an extension of the lower boundary from 97 to 30 km, including the physical and chemical processes appropriate for the Mesosphere and upper Stratosphere.
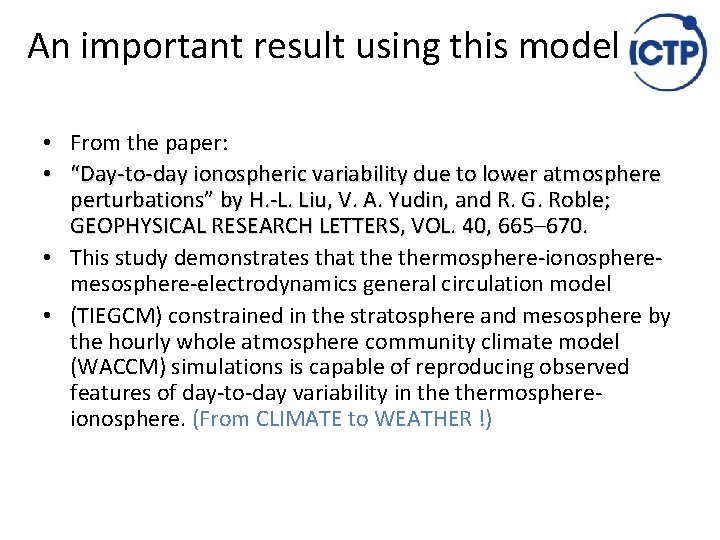
An important result using this model • From the paper: • “Day‐to‐day ionospheric variability due to lower atmosphere perturbations” by H. ‐L. Liu, V. A. Yudin, and R. G. Roble; GEOPHYSICAL RESEARCH LETTERS, VOL. 40, 665– 670. • This study demonstrates that thermosphere‐ionosphere‐ mesosphere‐electrodynamics general circulation model • (TIEGCM) constrained in the stratosphere and mesosphere by the hourly whole atmosphere community climate model (WACCM) simulations is capable of reproducing observed features of day‐to‐day variability in thermosphere‐ ionosphere. (From CLIMATE to WEATHER !)
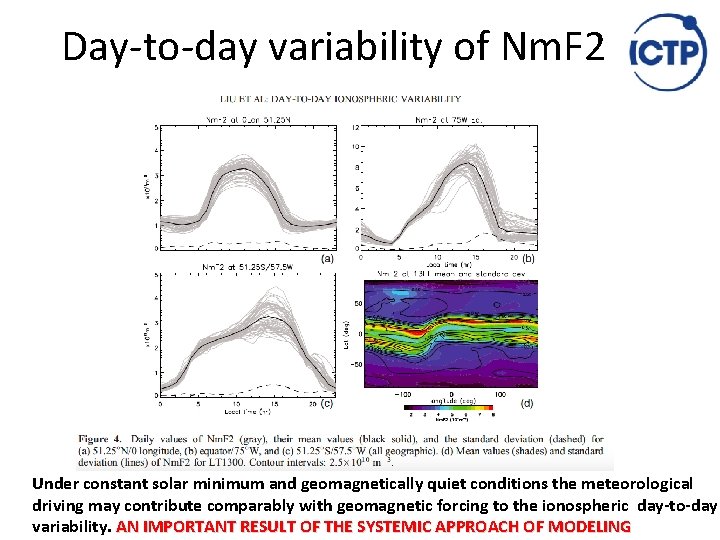
Day‐to‐day variability of Nm. F 2 Under constant solar minimum and geomagnetically quiet conditions the meteorological driving may contribute comparably with geomagnetic forcing to the ionospheric day-to-day variability. AN IMPORTANT RESULT OF THE SYSTEMIC APPROACH OF MODELING
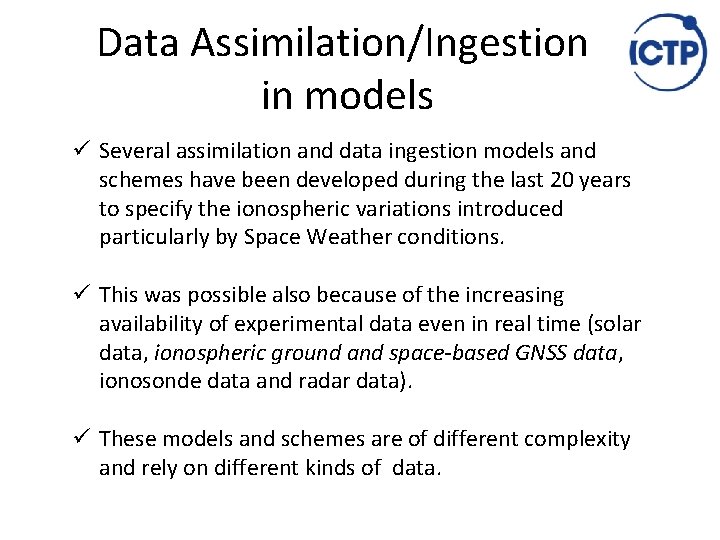
Data Assimilation/Ingestion in models ü Several assimilation and data ingestion models and schemes have been developed during the last 20 years to specify the ionospheric variations introduced particularly by Space Weather conditions. ü This was possible also because of the increasing availability of experimental data even in real time (solar data, ionospheric ground and space-based GNSS data, ionosonde data and radar data). ü These models and schemes are of different complexity and rely on different kinds of data.
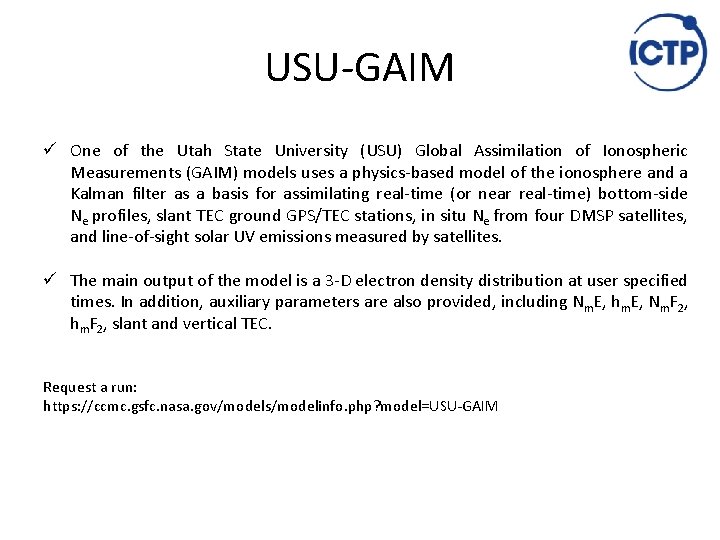
USU‐GAIM ü One of the Utah State University (USU) Global Assimilation of Ionospheric Measurements (GAIM) models uses a physics‐based model of the ionosphere and a Kalman filter as a basis for assimilating real‐time (or near real‐time) bottom‐side Ne profiles, slant TEC ground GPS/TEC stations, in situ Ne from four DMSP satellites, and line‐of‐sight solar UV emissions measured by satellites. ü The main output of the model is a 3‐D electron density distribution at user specified times. In addition, auxiliary parameters are also provided, including Nm. E, hm. E, Nm. F 2, hm. F 2, slant and vertical TEC. Request a run: https: //ccmc. gsfc. nasa. gov/models/modelinfo. php? model=USU‐GAIM
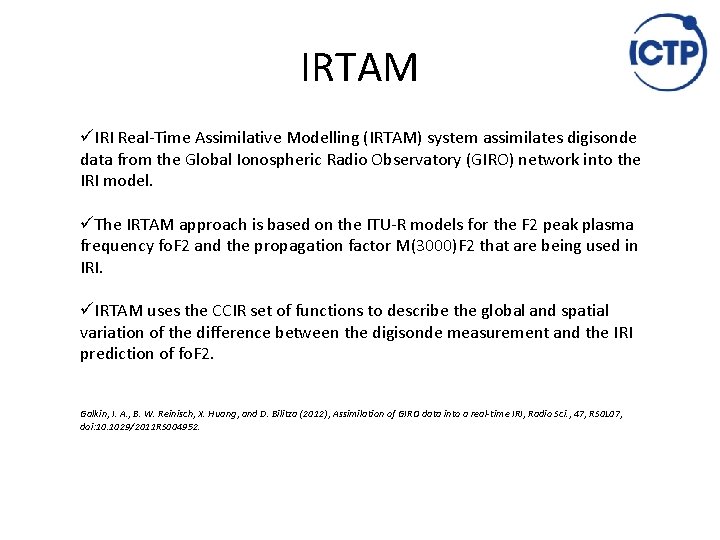
IRTAM üIRI Real‐Time Assimilative Modelling (IRTAM) system assimilates digisonde data from the Global Ionospheric Radio Observatory (GIRO) network into the IRI model. üThe IRTAM approach is based on the ITU‐R models for the F 2 peak plasma frequency fo. F 2 and the propagation factor M(3000)F 2 that are being used in IRI. üIRTAM uses the CCIR set of functions to describe the global and spatial variation of the difference between the digisonde measurement and the IRI prediction of fo. F 2. Galkin, I. A. , B. W. Reinisch, X. Huang, and D. Bilitza (2012), Assimilation of GIRO data into a real-time IRI, Radio Sci. , 47, RS 0 L 07, doi: 10. 1029/2011 RS 004952.
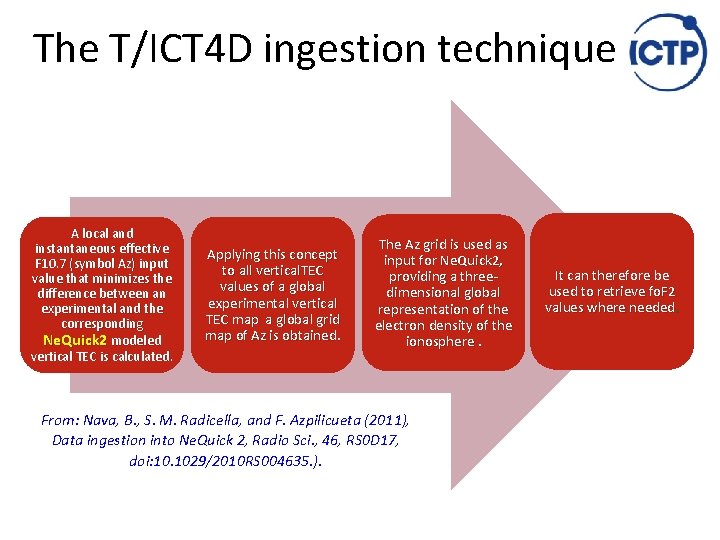
The T/ICT 4 D ingestion technique A local and instantaneous effective F 10. 7 (symbol Az) input value that minimizes the difference between an experimental and the corresponding Ne. Quick 2 modeled vertical TEC is calculated. Applying this concept to all vertical. TEC values of a global experimental vertical TEC map a global grid map of Az is obtained. The Az grid is used as input for Ne. Quick 2, providing a three‐ dimensional global representation of the electron density of the ionosphere. From: Nava, B. , S. M. Radicella, and F. Azpilicueta (2011), Data ingestion into Ne. Quick 2, Radio Sci. , 46, RS 0 D 17, doi: 10. 1029/2010 RS 004635. ). It can therefore be used to retrieve fo. F 2 values where needed.
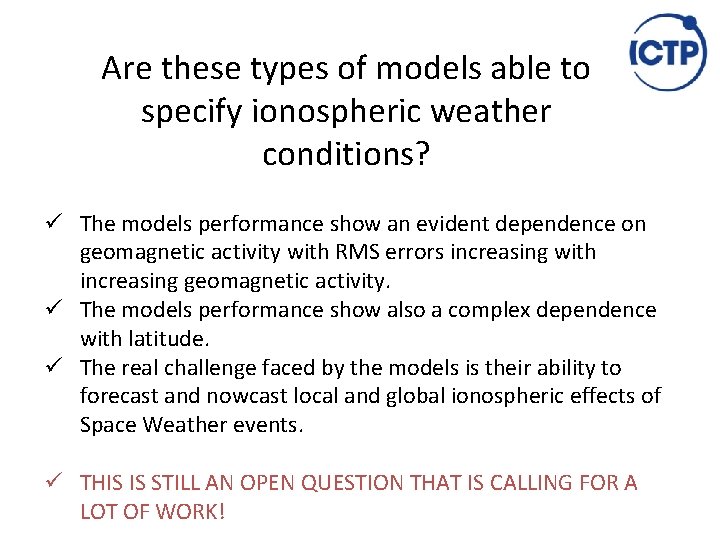
Are these types of models able to specify ionospheric weather conditions? ü The models performance show an evident dependence on geomagnetic activity with RMS errors increasing with increasing geomagnetic activity. ü The models performance show also a complex dependence with latitude. ü The real challenge faced by the models is their ability to forecast and nowcast local and global ionospheric effects of Space Weather events. ü THIS IS STILL AN OPEN QUESTION THAT IS CALLING FOR A LOT OF WORK!
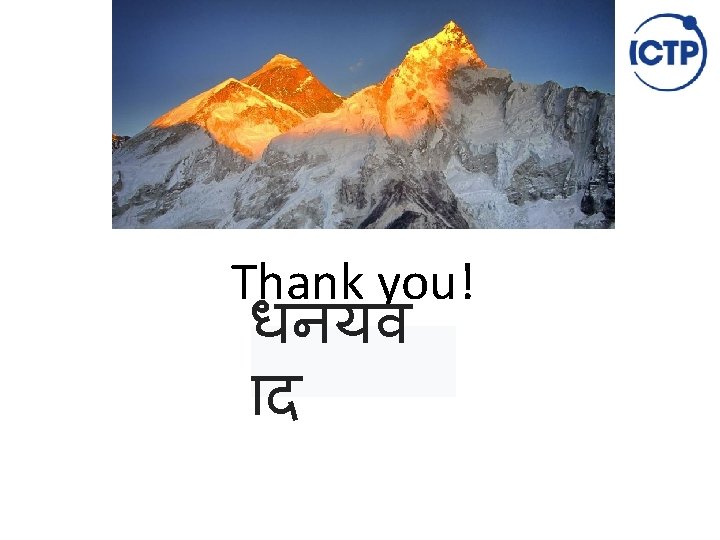