HEART CARDIAC CYCLE CARDIAC CYCLE A single cardiac
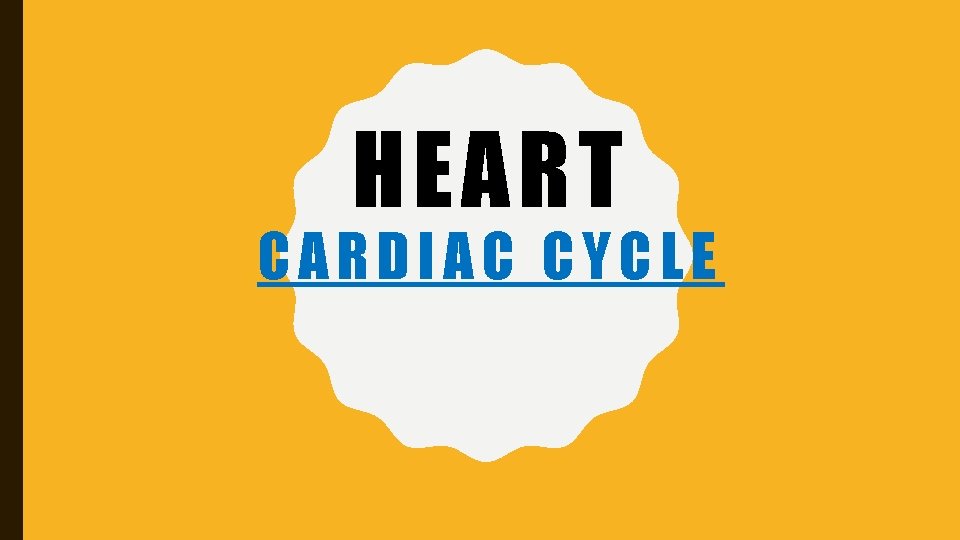
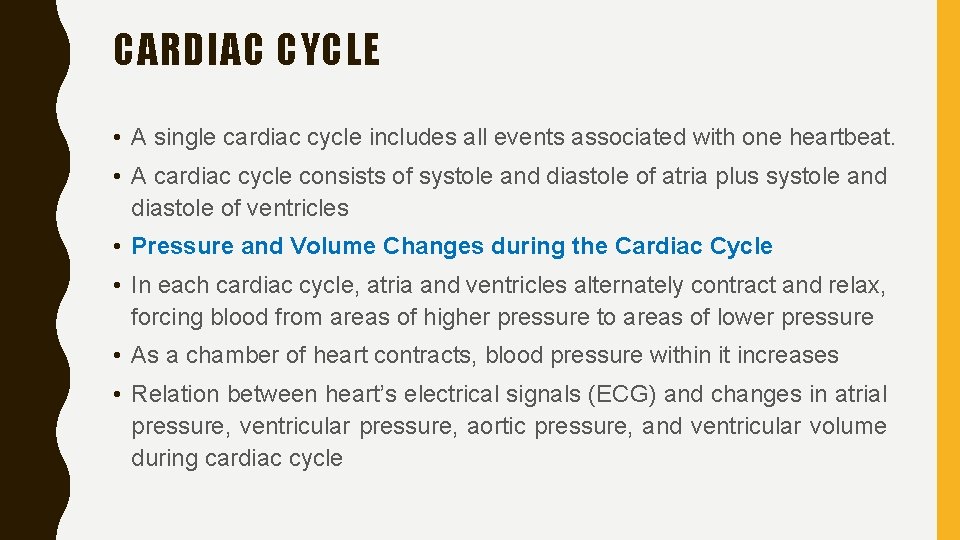
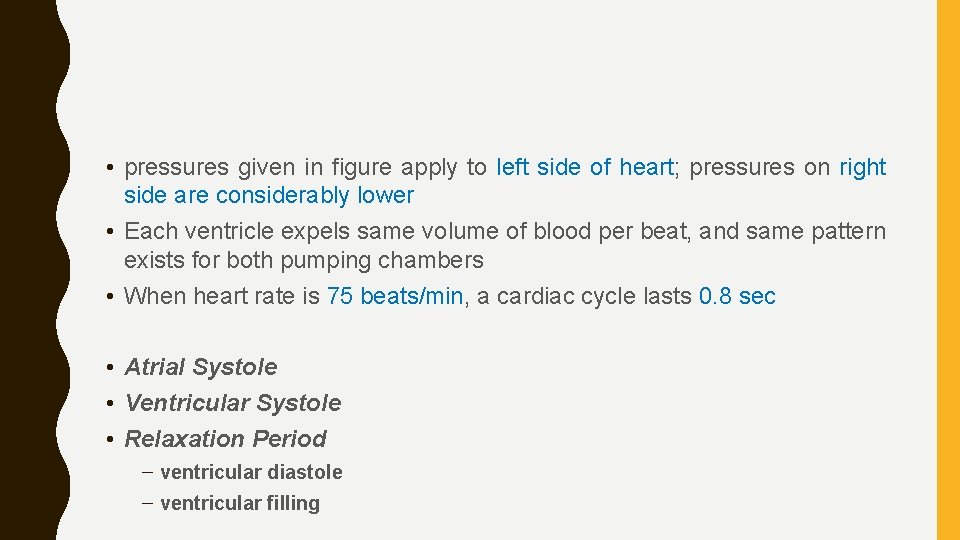
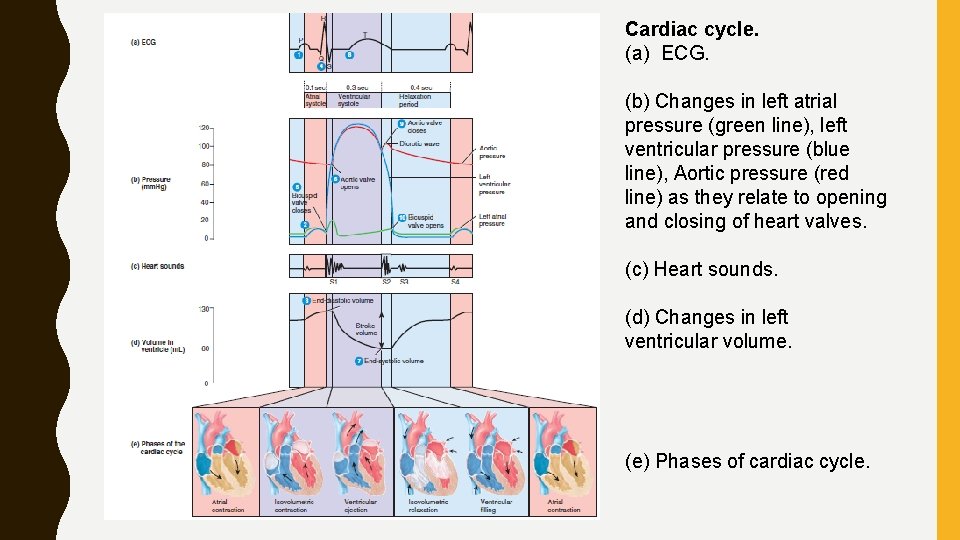
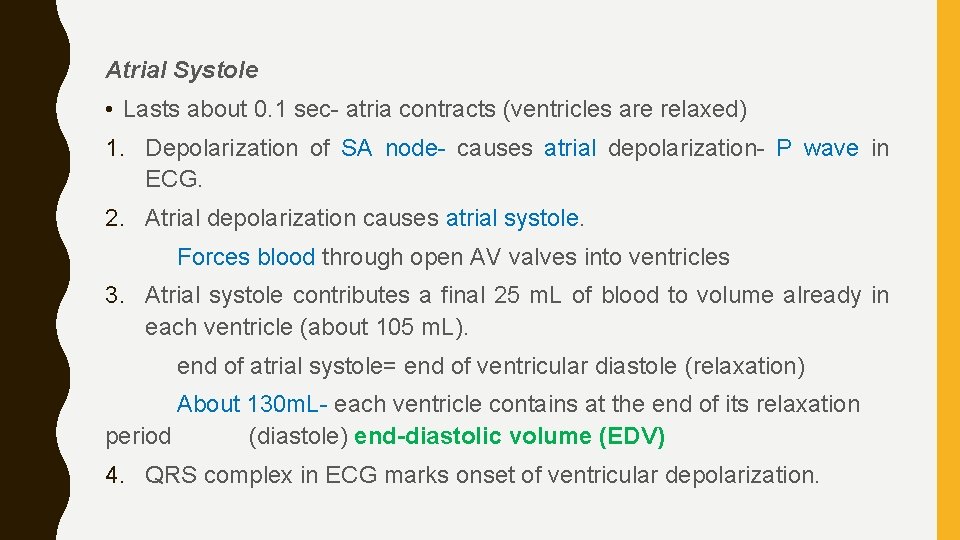
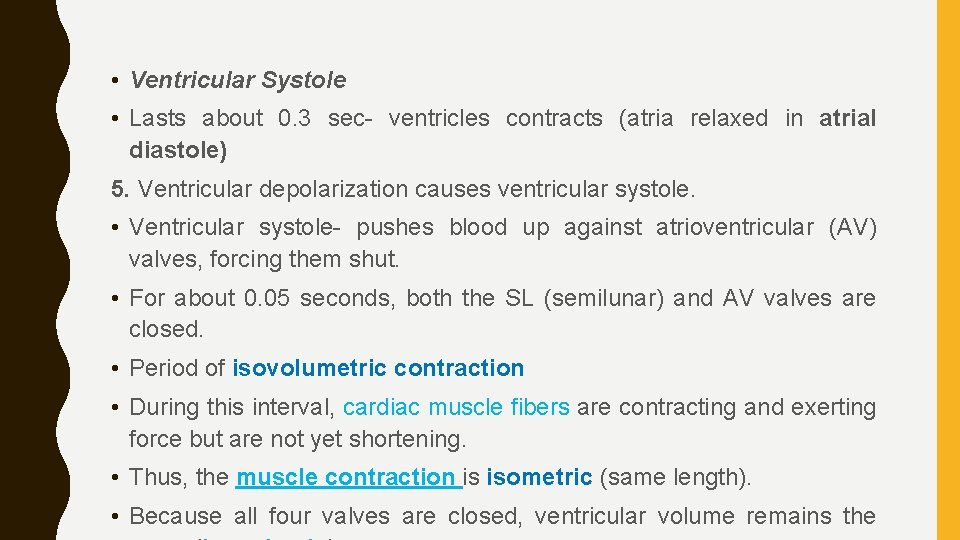
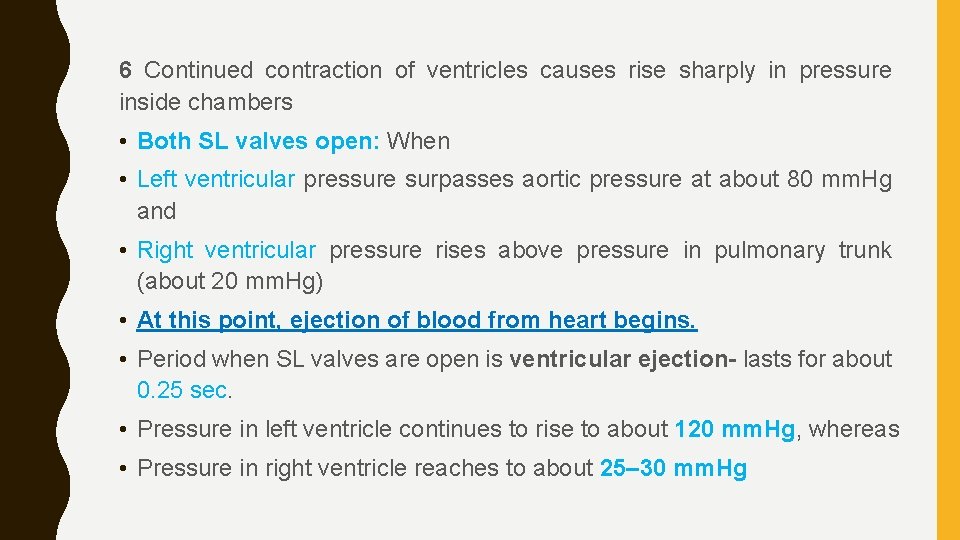
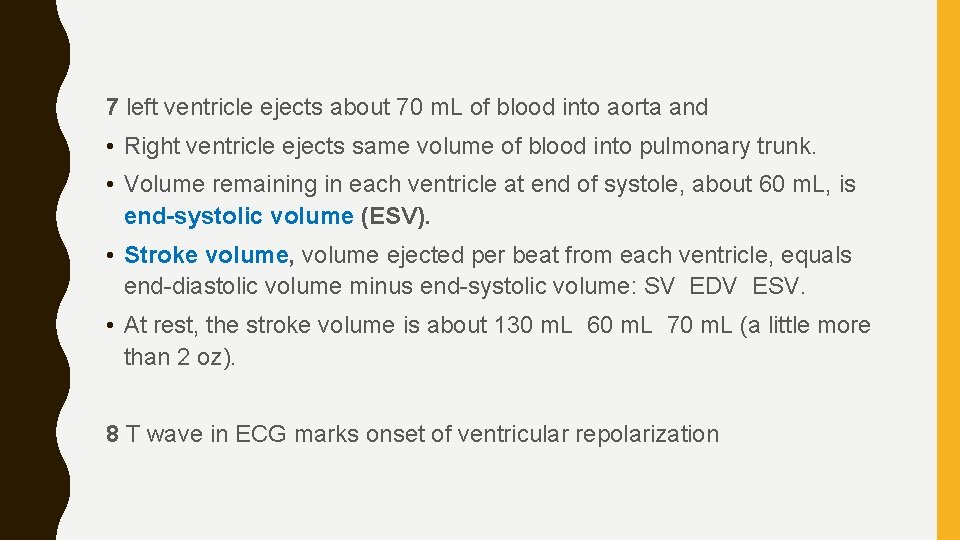
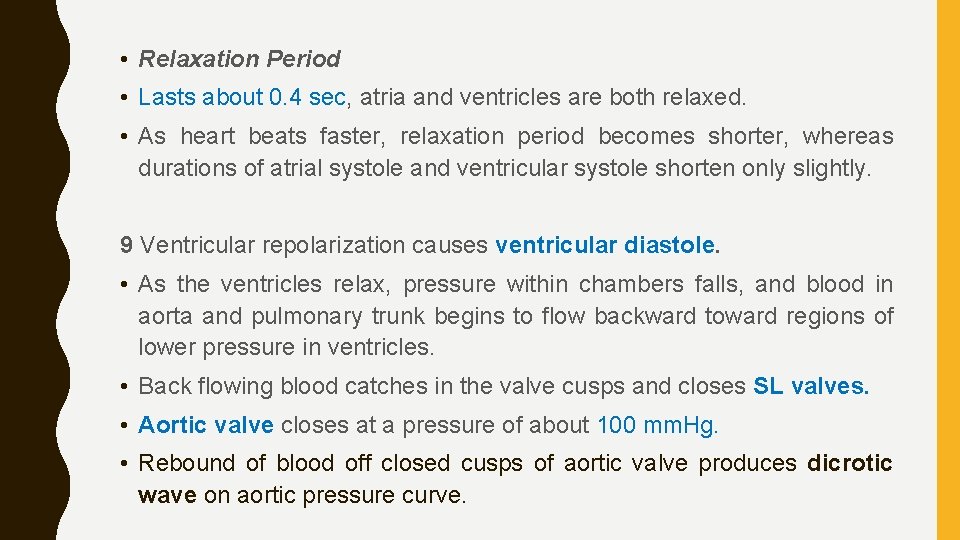
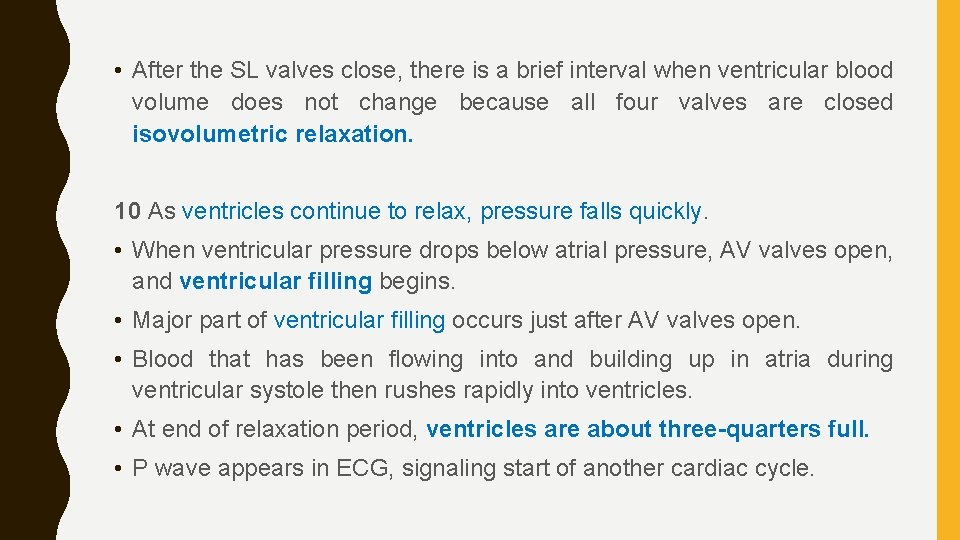
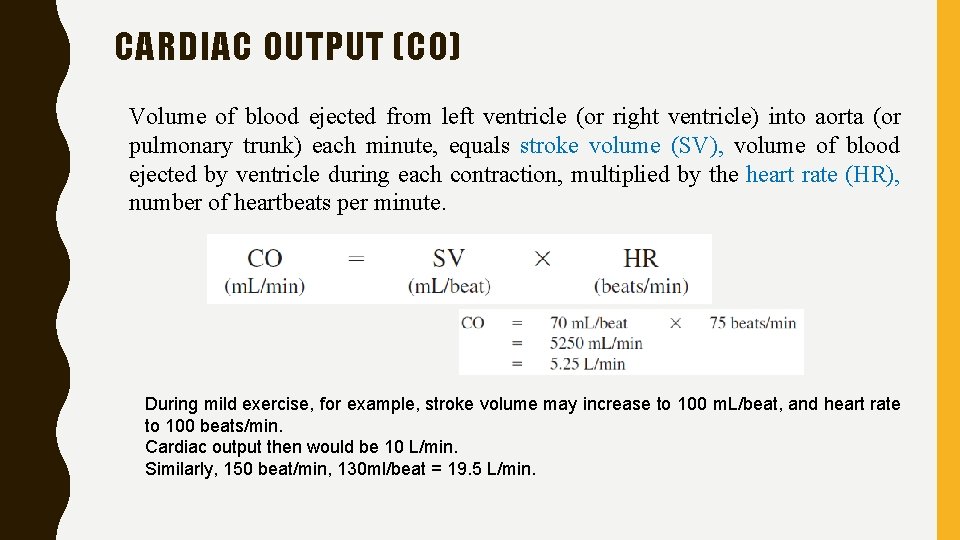
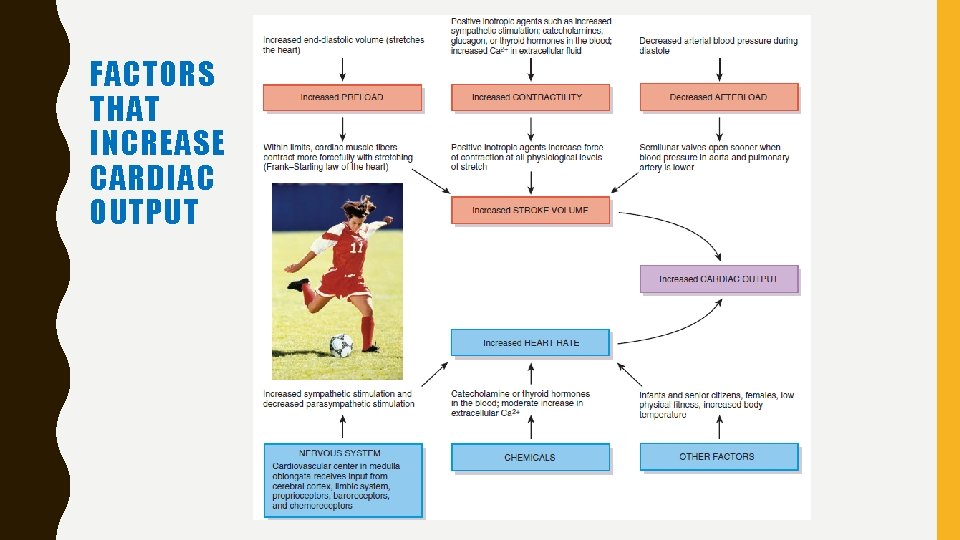
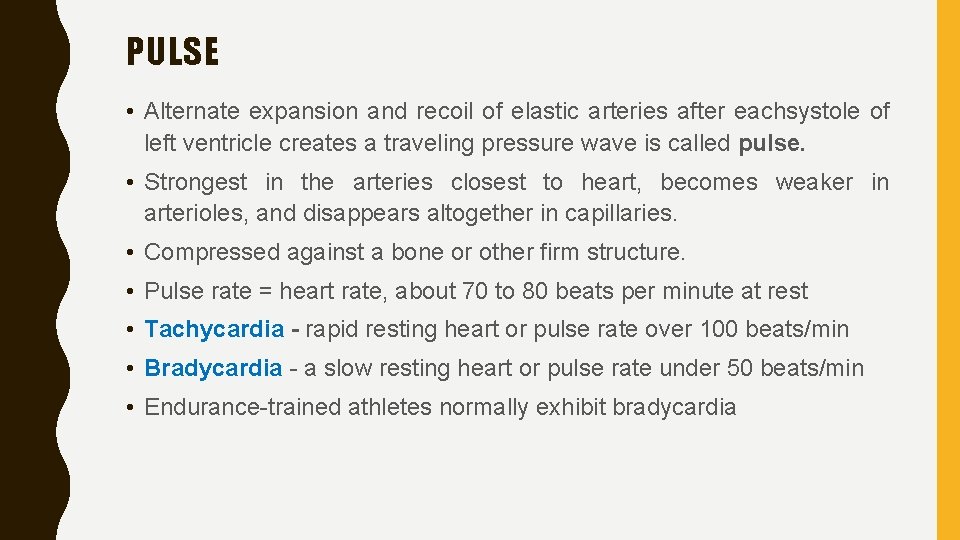
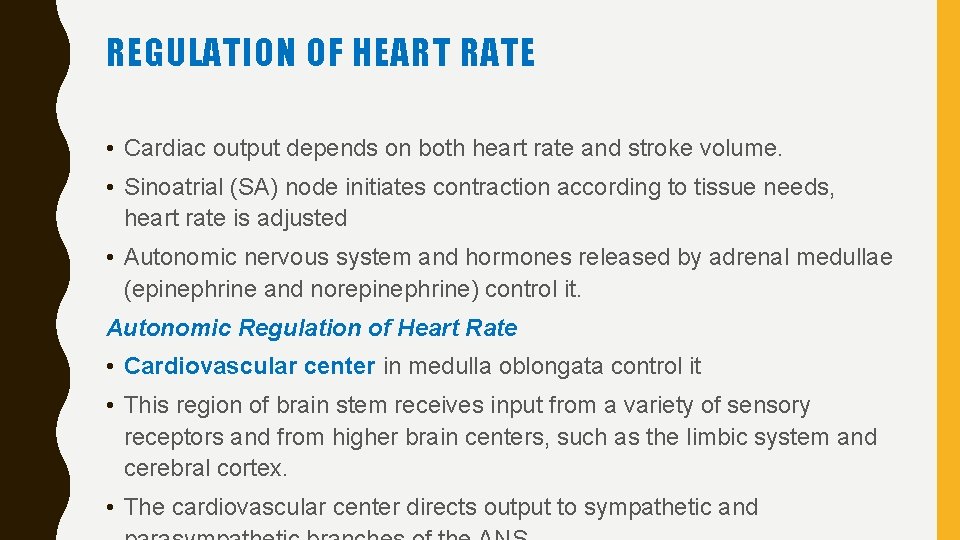
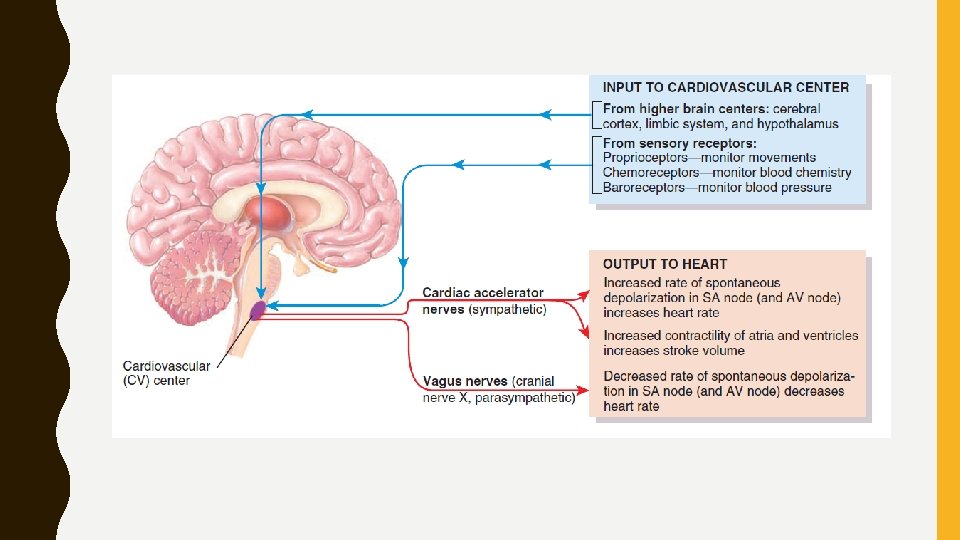
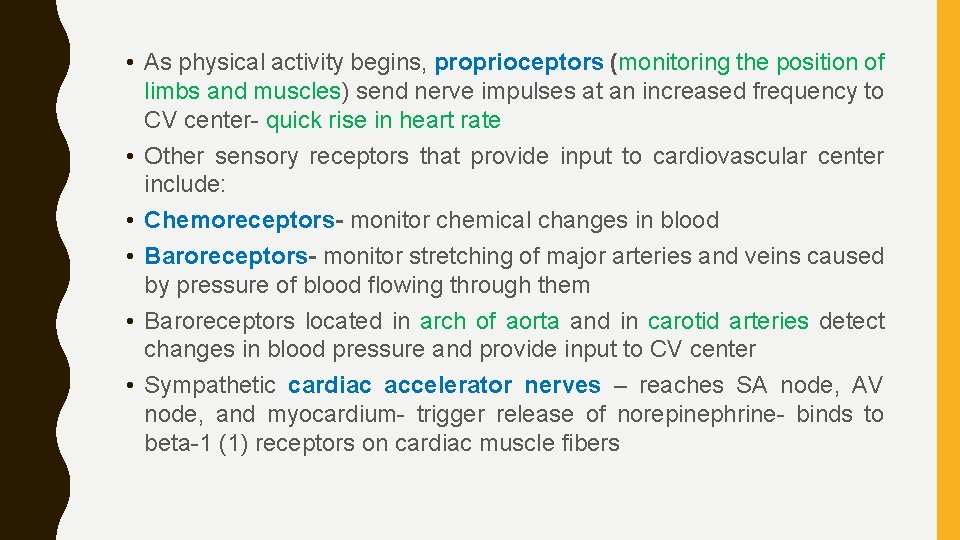
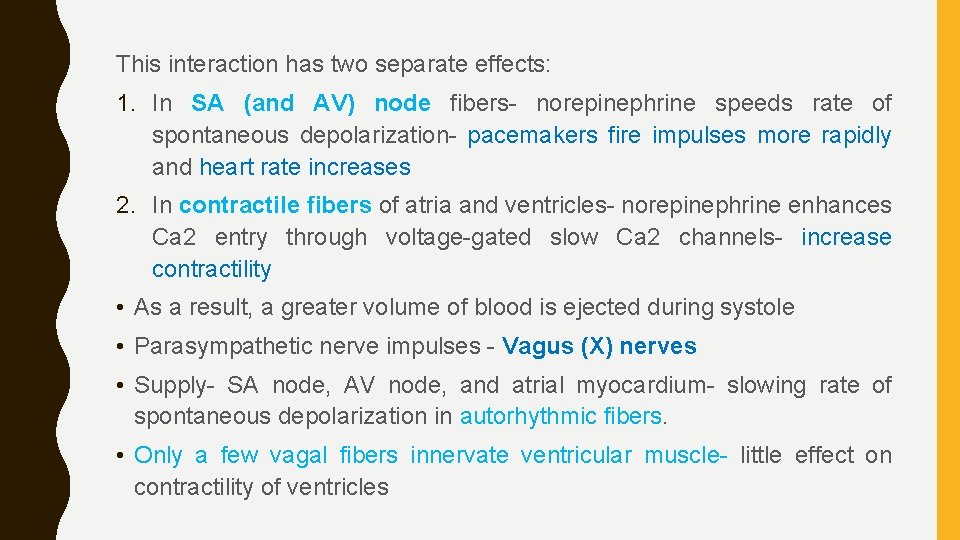
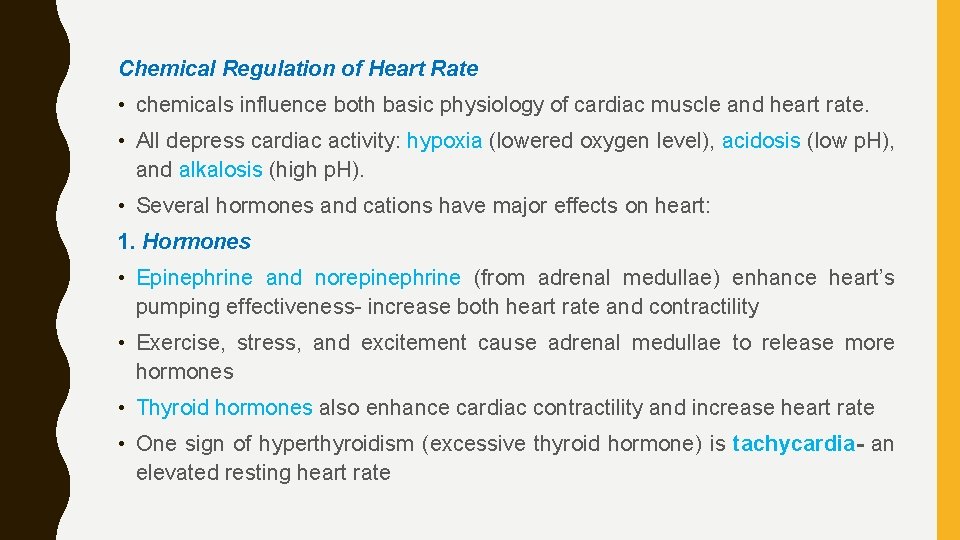
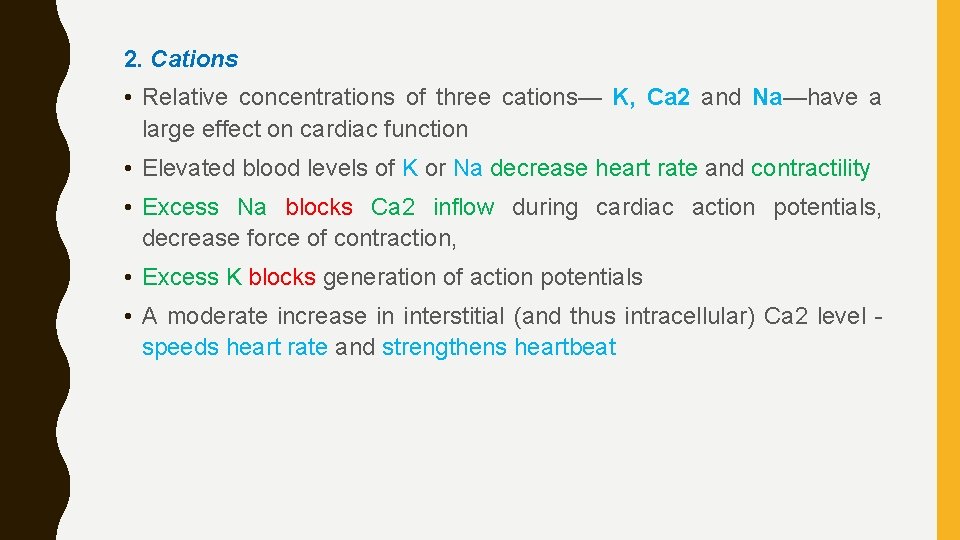
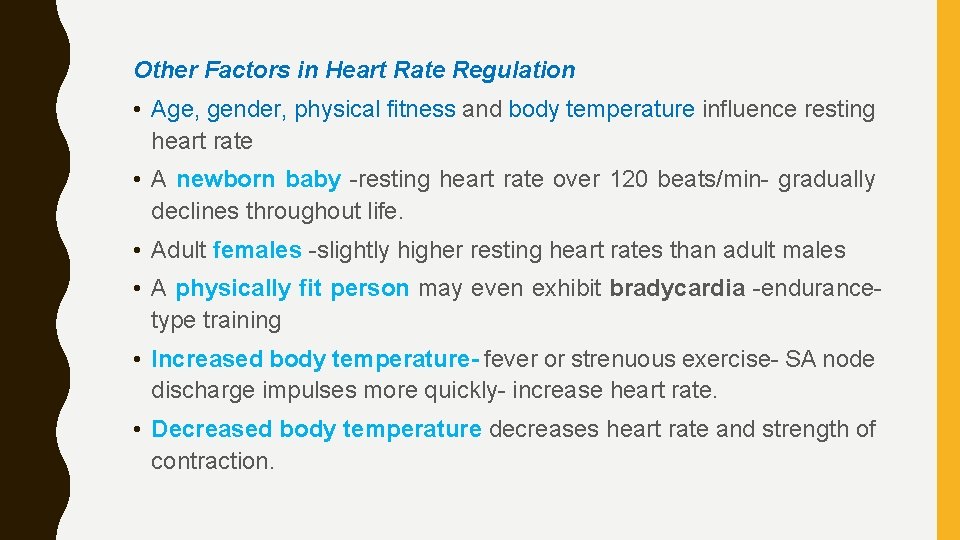
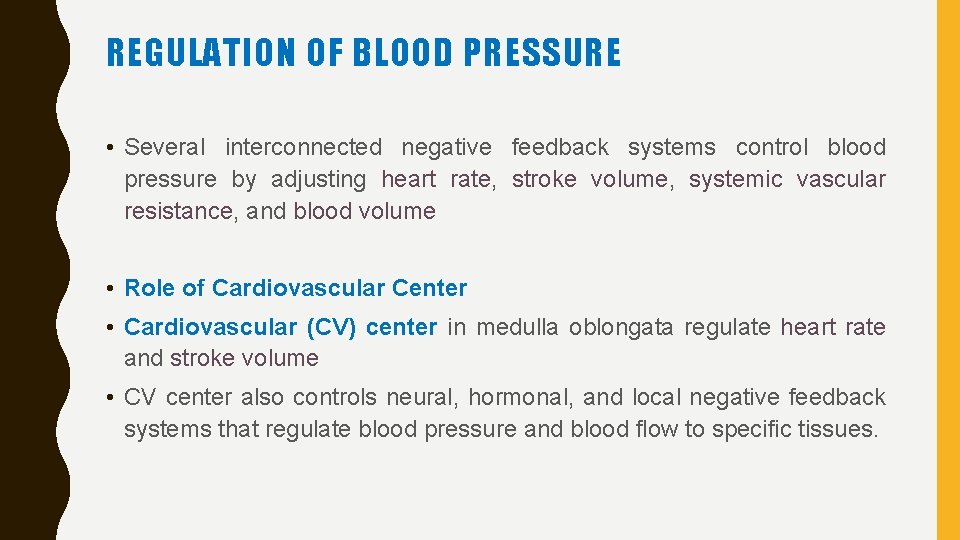
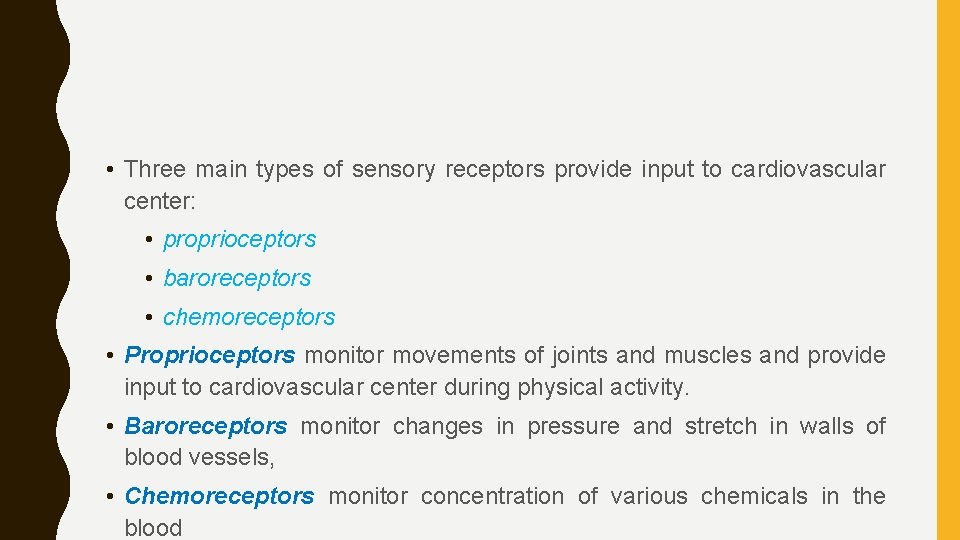
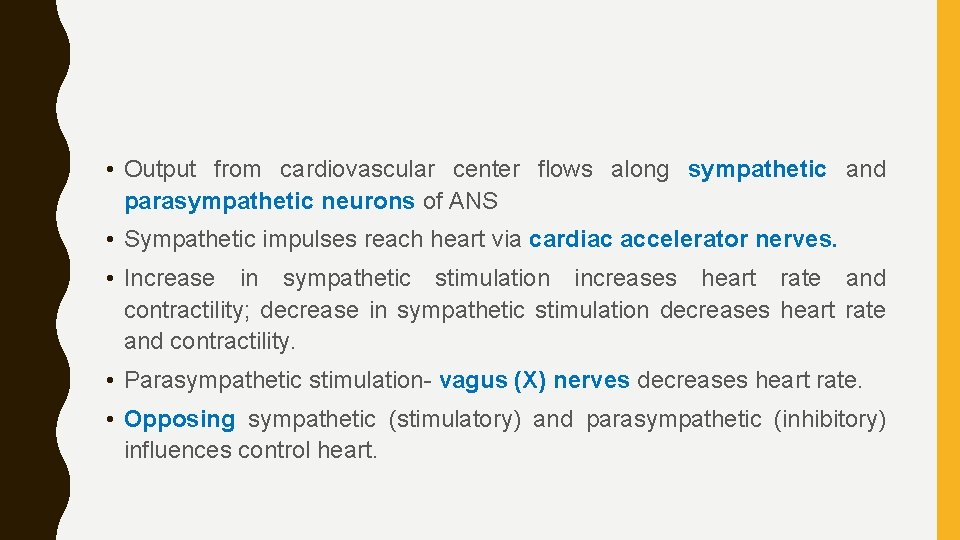
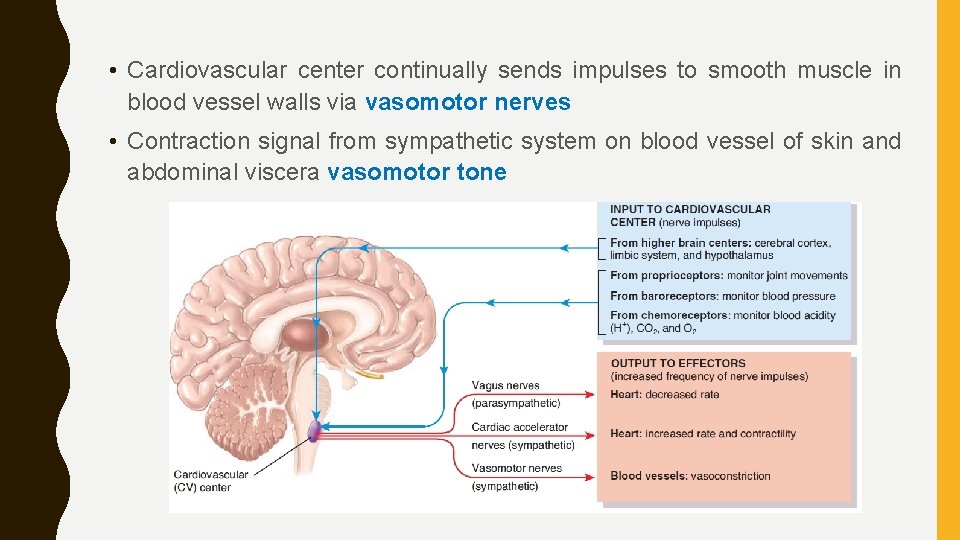
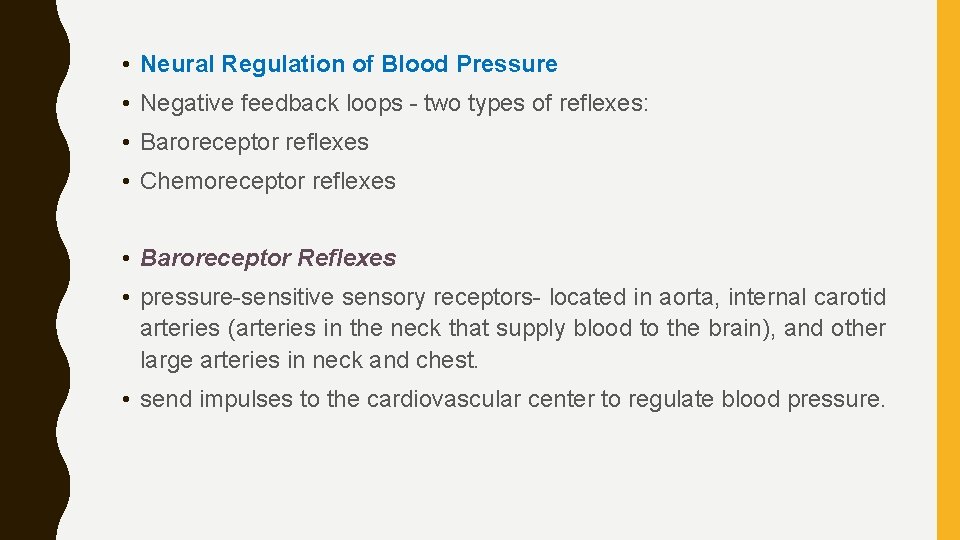
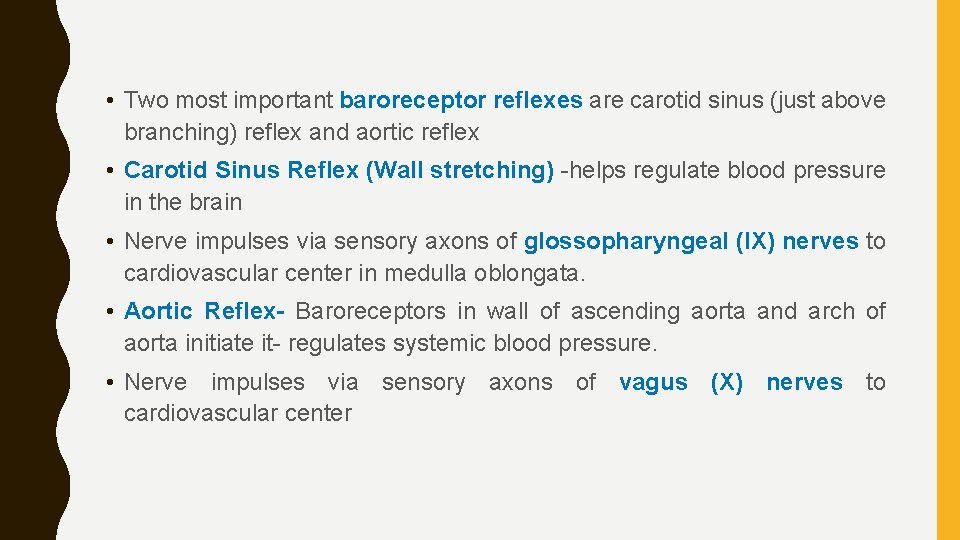
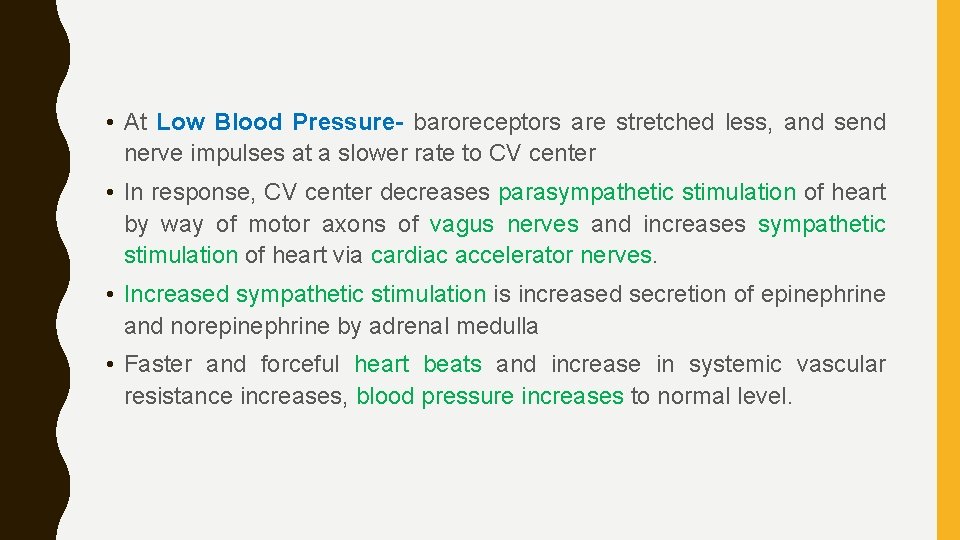
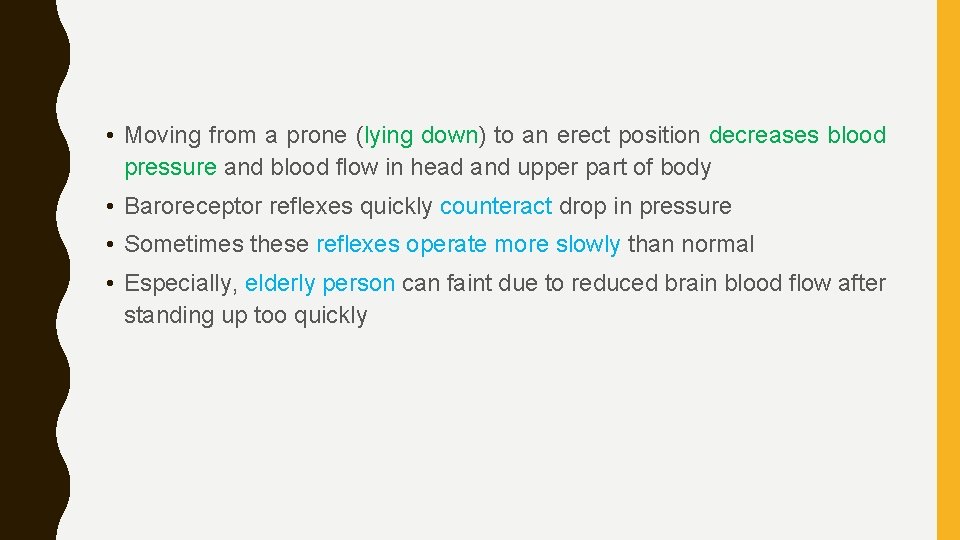
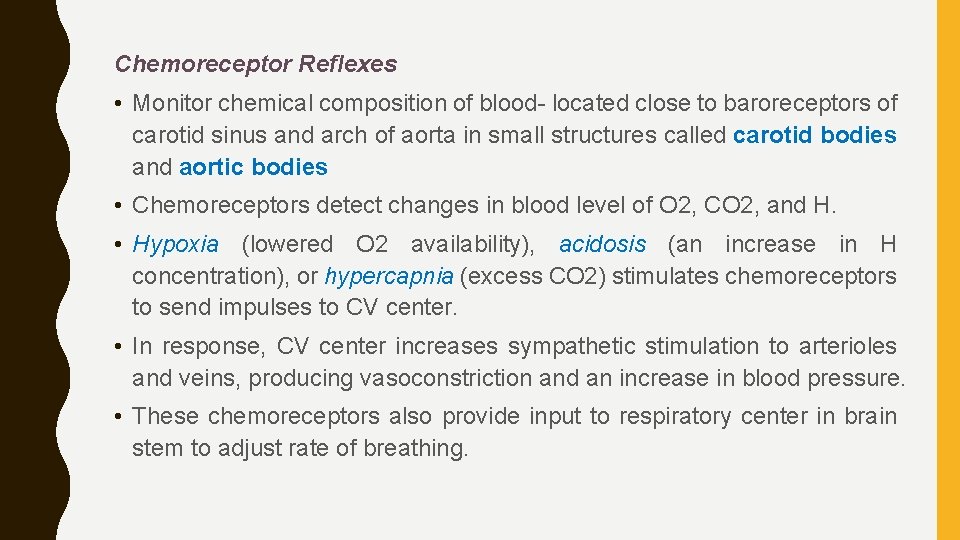
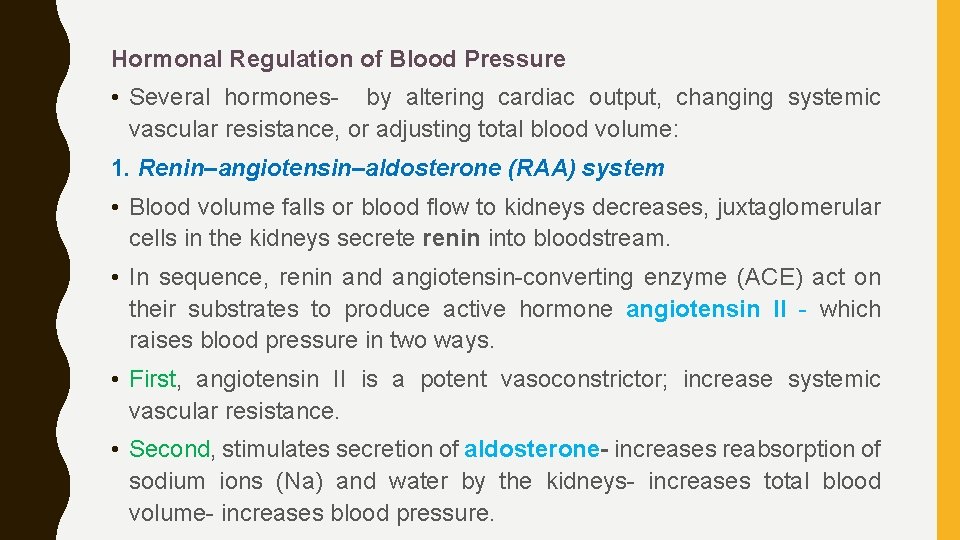
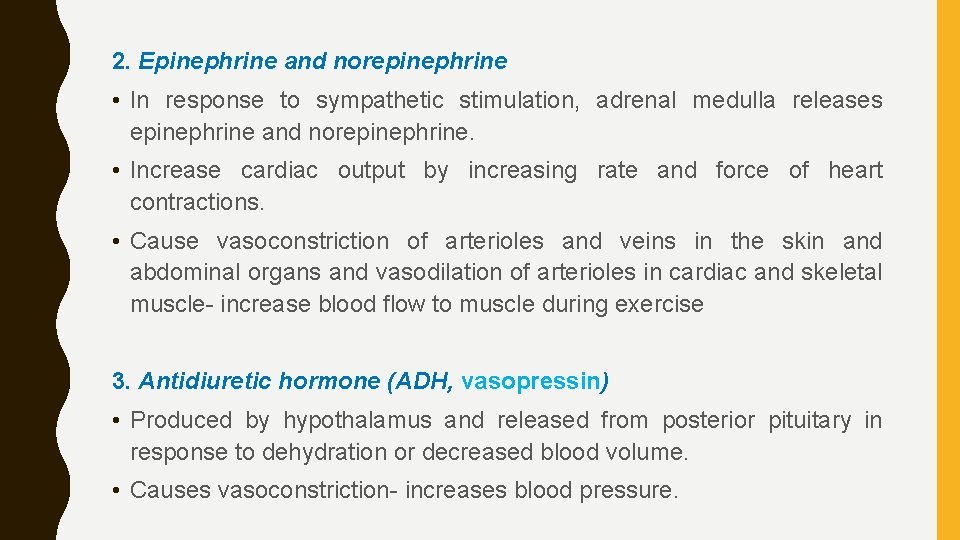
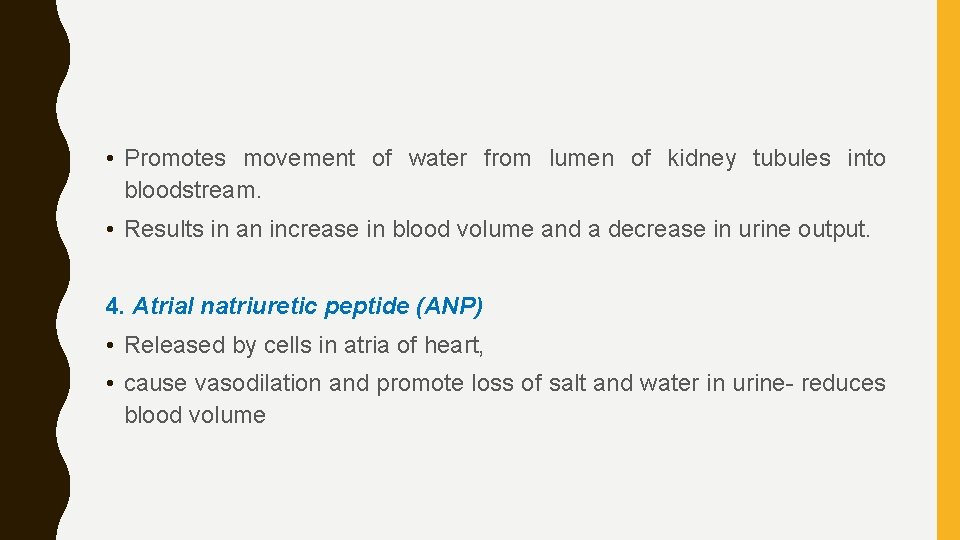
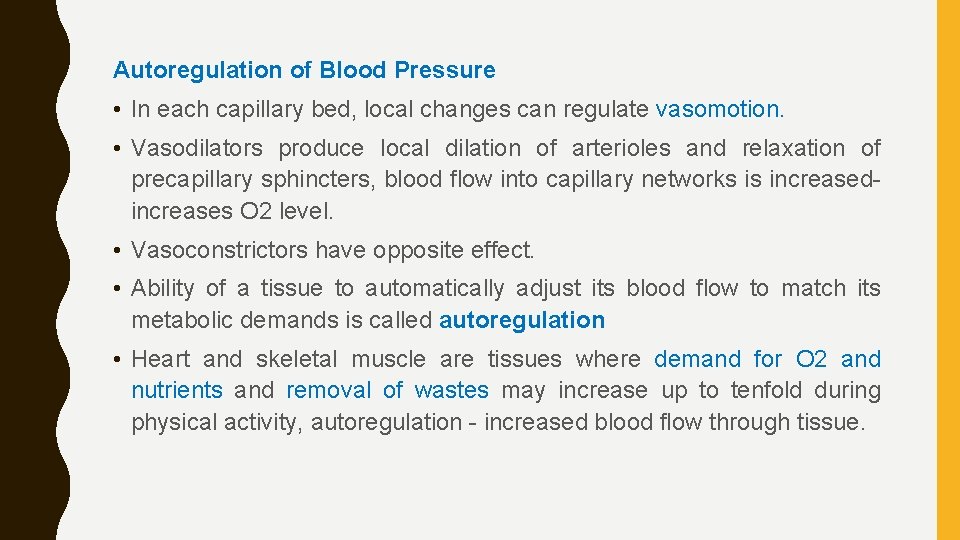
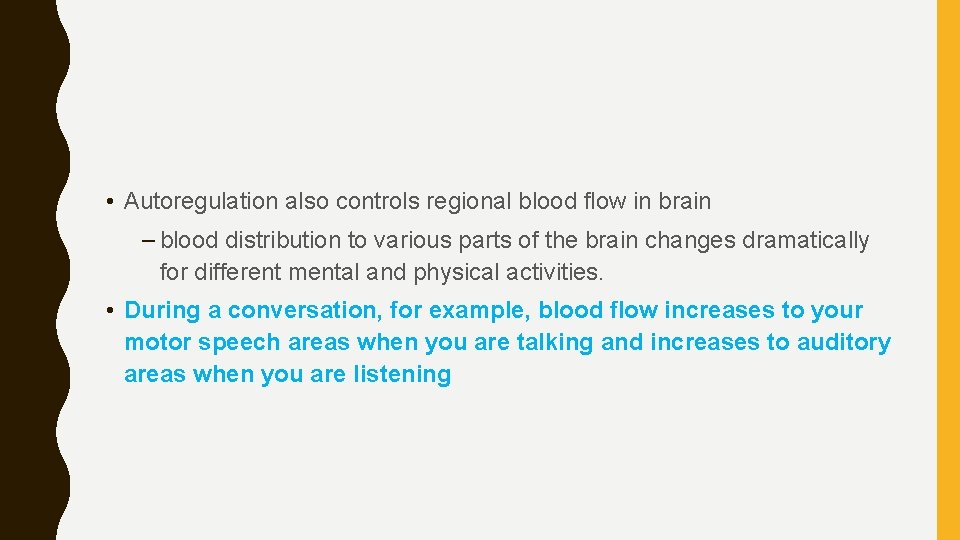
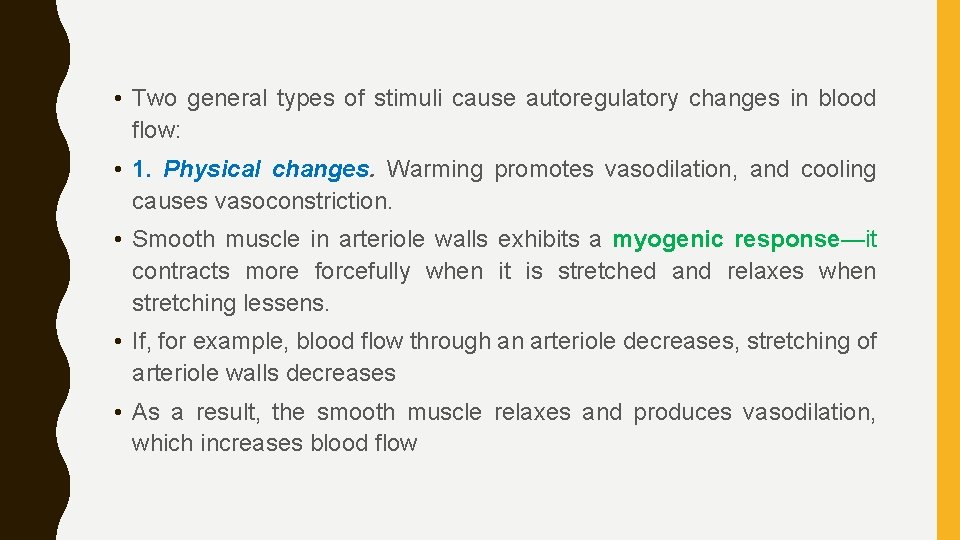
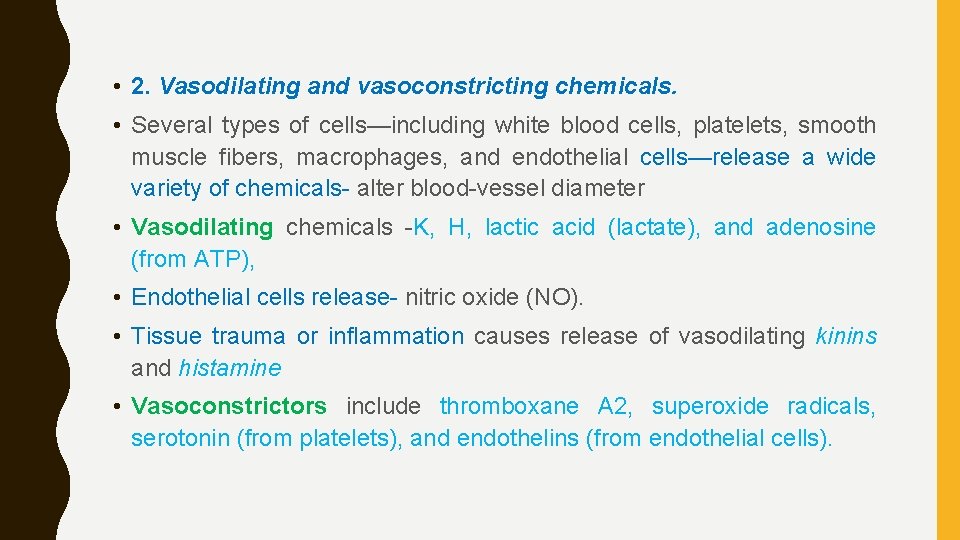
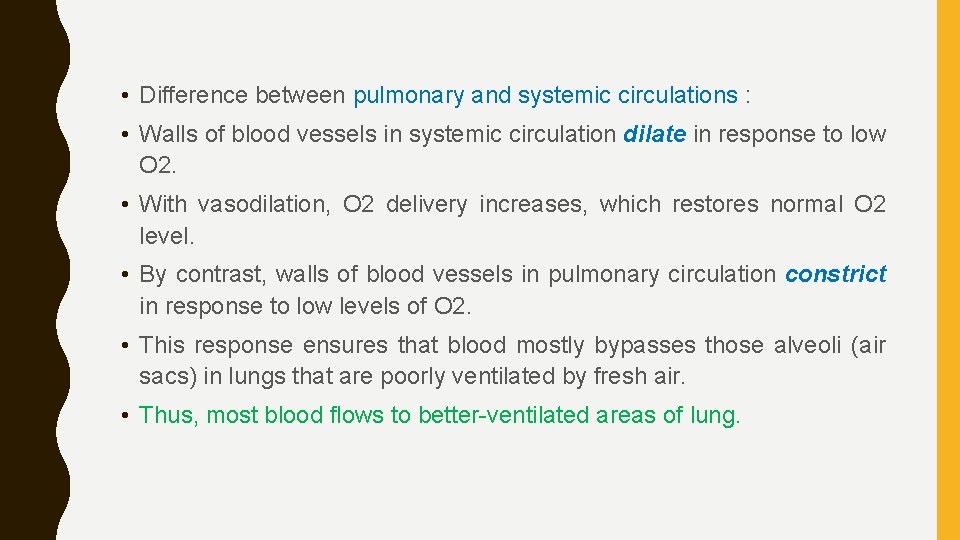
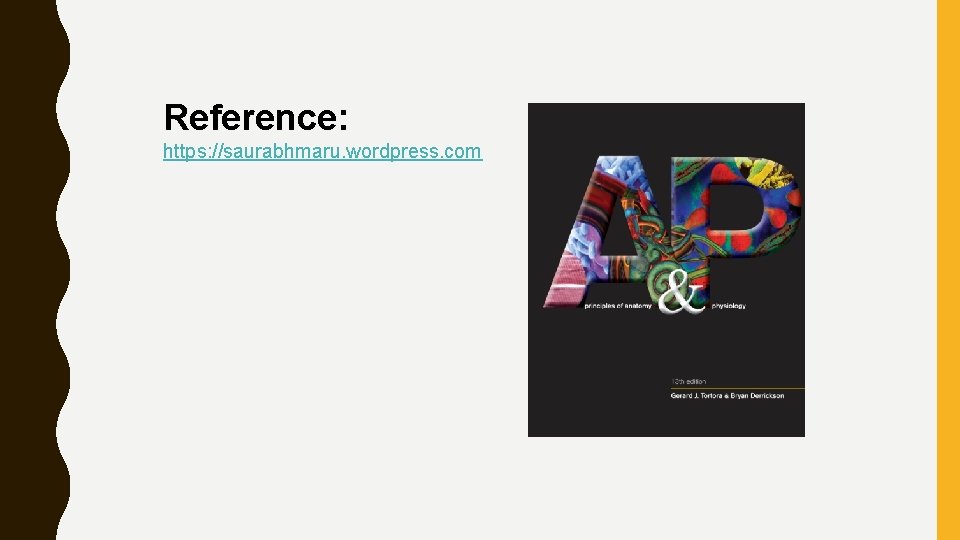
- Slides: 38
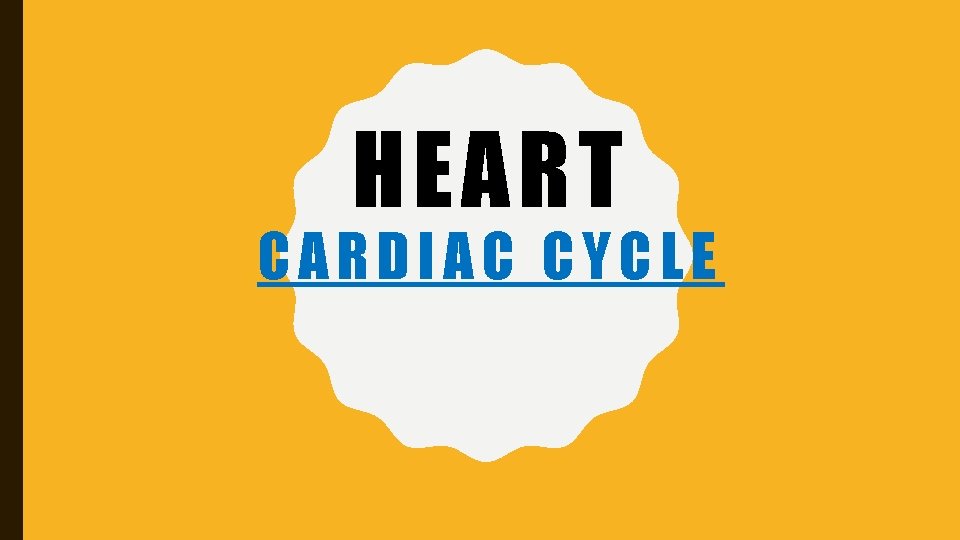
HEART CARDIAC CYCLE
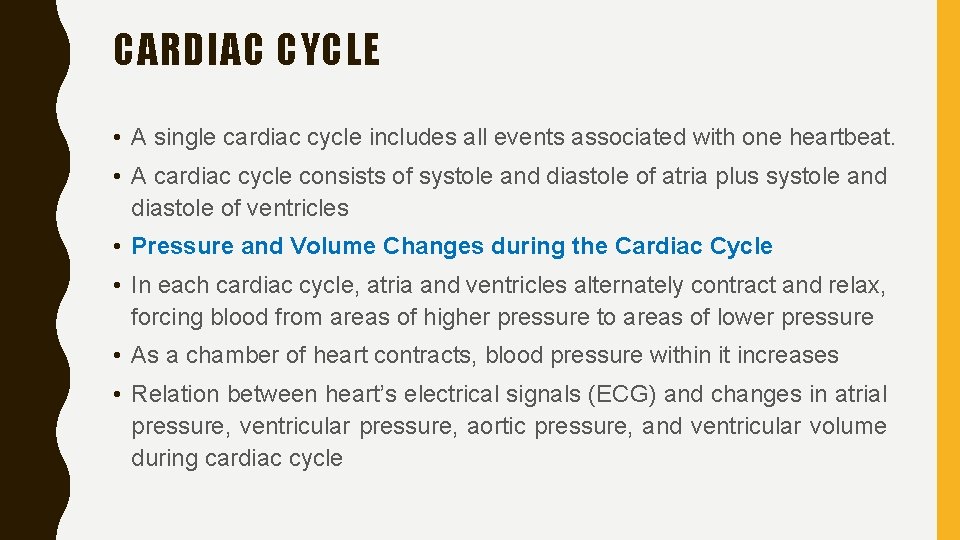
CARDIAC CYCLE • A single cardiac cycle includes all events associated with one heartbeat. • A cardiac cycle consists of systole and diastole of atria plus systole and diastole of ventricles • Pressure and Volume Changes during the Cardiac Cycle • In each cardiac cycle, atria and ventricles alternately contract and relax, forcing blood from areas of higher pressure to areas of lower pressure • As a chamber of heart contracts, blood pressure within it increases • Relation between heart’s electrical signals (ECG) and changes in atrial pressure, ventricular pressure, aortic pressure, and ventricular volume during cardiac cycle
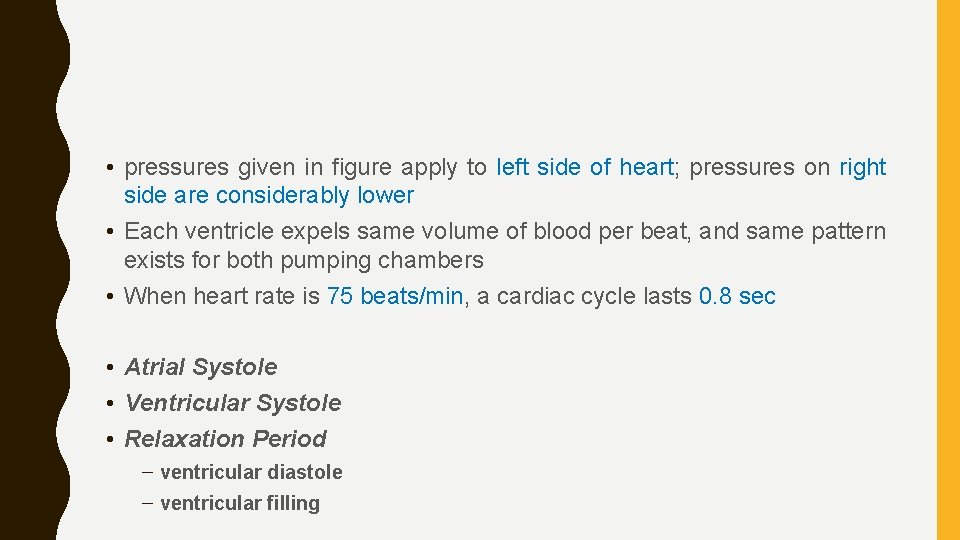
• pressures given in figure apply to left side of heart; pressures on right side are considerably lower • Each ventricle expels same volume of blood per beat, and same pattern exists for both pumping chambers • When heart rate is 75 beats/min, a cardiac cycle lasts 0. 8 sec • Atrial Systole • Ventricular Systole • Relaxation Period – ventricular diastole – ventricular filling
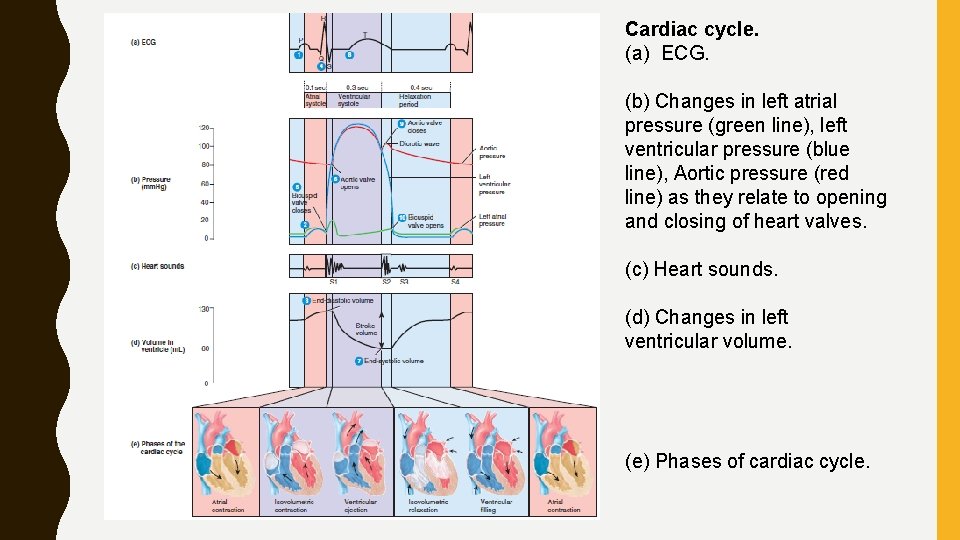
Cardiac cycle. (a) ECG. (b) Changes in left atrial pressure (green line), left ventricular pressure (blue line), Aortic pressure (red line) as they relate to opening and closing of heart valves. (c) Heart sounds. (d) Changes in left ventricular volume. (e) Phases of cardiac cycle.
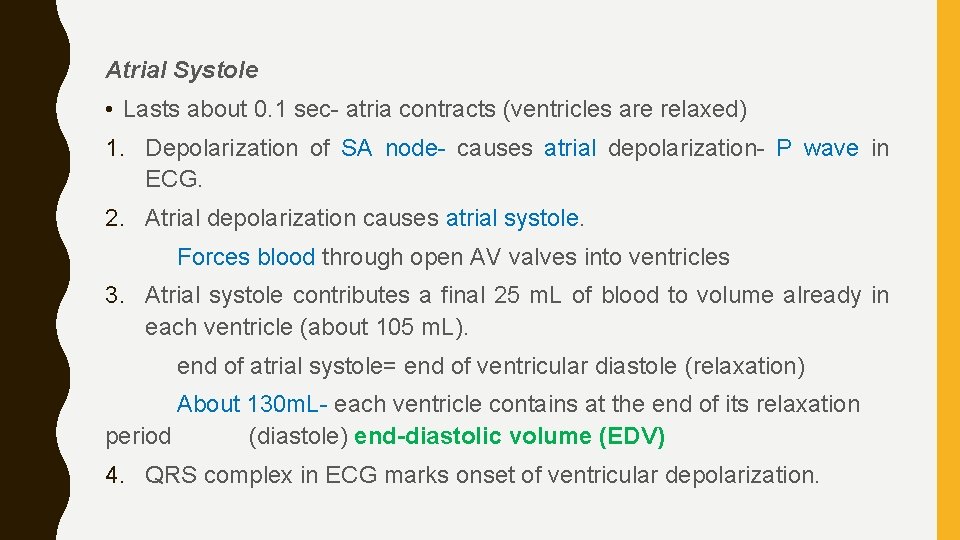
Atrial Systole • Lasts about 0. 1 sec- atria contracts (ventricles are relaxed) 1. Depolarization of SA node- causes atrial depolarization- P wave in ECG. 2. Atrial depolarization causes atrial systole. Forces blood through open AV valves into ventricles 3. Atrial systole contributes a final 25 m. L of blood to volume already in each ventricle (about 105 m. L). end of atrial systole= end of ventricular diastole (relaxation) About 130 m. L- each ventricle contains at the end of its relaxation period (diastole) end-diastolic volume (EDV) 4. QRS complex in ECG marks onset of ventricular depolarization.
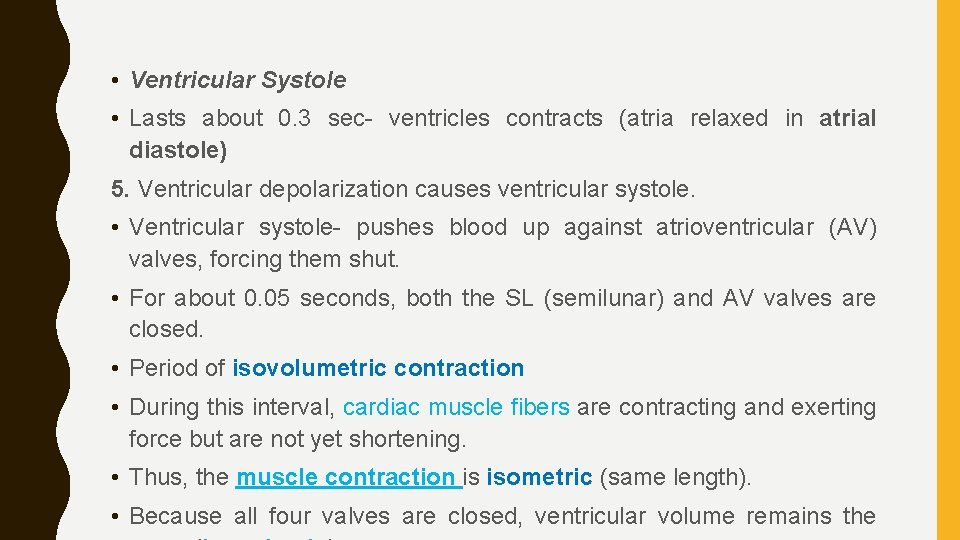
• Ventricular Systole • Lasts about 0. 3 sec- ventricles contracts (atria relaxed in atrial diastole) 5. Ventricular depolarization causes ventricular systole. • Ventricular systole- pushes blood up against atrioventricular (AV) valves, forcing them shut. • For about 0. 05 seconds, both the SL (semilunar) and AV valves are closed. • Period of isovolumetric contraction • During this interval, cardiac muscle fibers are contracting and exerting force but are not yet shortening. • Thus, the muscle contraction is isometric (same length). • Because all four valves are closed, ventricular volume remains the
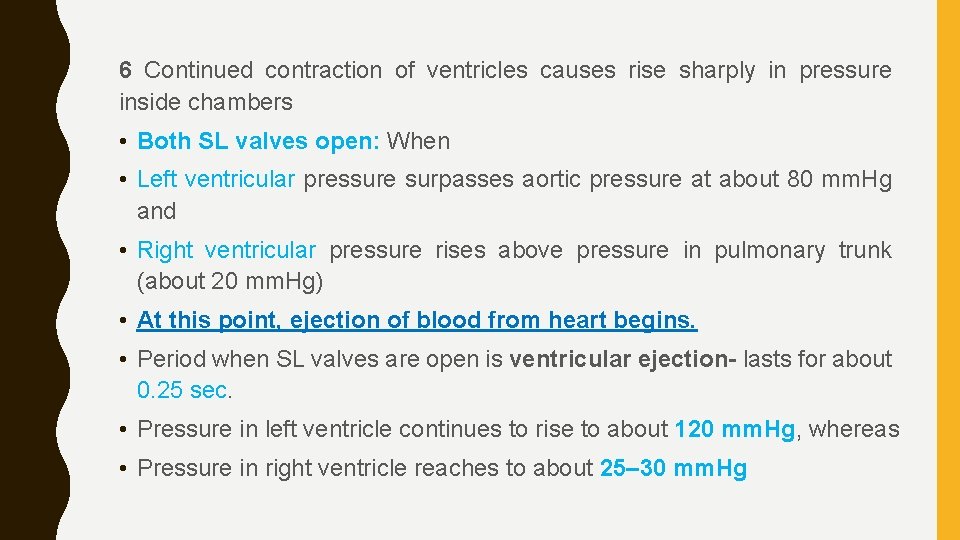
6 Continued contraction of ventricles causes rise sharply in pressure inside chambers • Both SL valves open: When • Left ventricular pressure surpasses aortic pressure at about 80 mm. Hg and • Right ventricular pressure rises above pressure in pulmonary trunk (about 20 mm. Hg) • At this point, ejection of blood from heart begins. • Period when SL valves are open is ventricular ejection- lasts for about 0. 25 sec. • Pressure in left ventricle continues to rise to about 120 mm. Hg, whereas • Pressure in right ventricle reaches to about 25– 30 mm. Hg
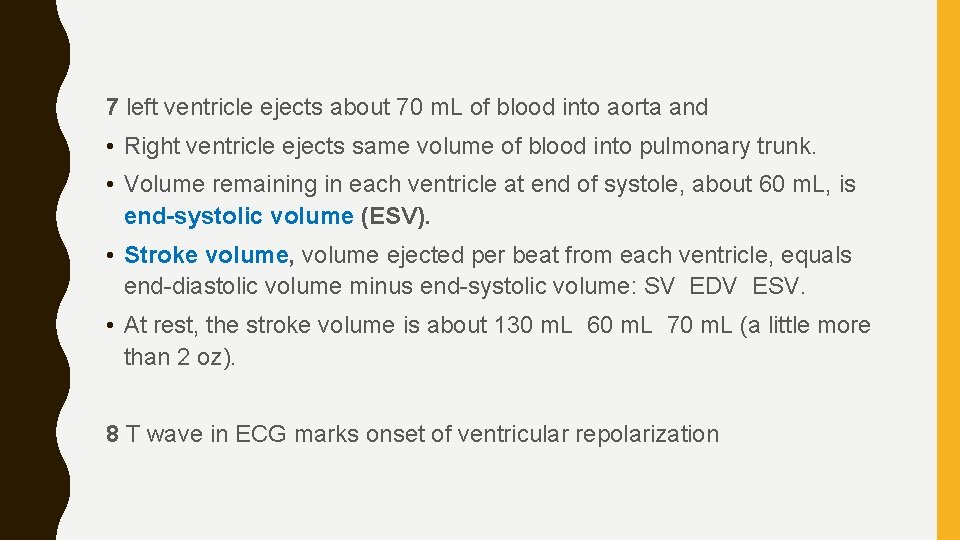
7 left ventricle ejects about 70 m. L of blood into aorta and • Right ventricle ejects same volume of blood into pulmonary trunk. • Volume remaining in each ventricle at end of systole, about 60 m. L, is end-systolic volume (ESV). • Stroke volume, volume ejected per beat from each ventricle, equals end-diastolic volume minus end-systolic volume: SV EDV ESV. • At rest, the stroke volume is about 130 m. L 60 m. L 70 m. L (a little more than 2 oz). 8 T wave in ECG marks onset of ventricular repolarization
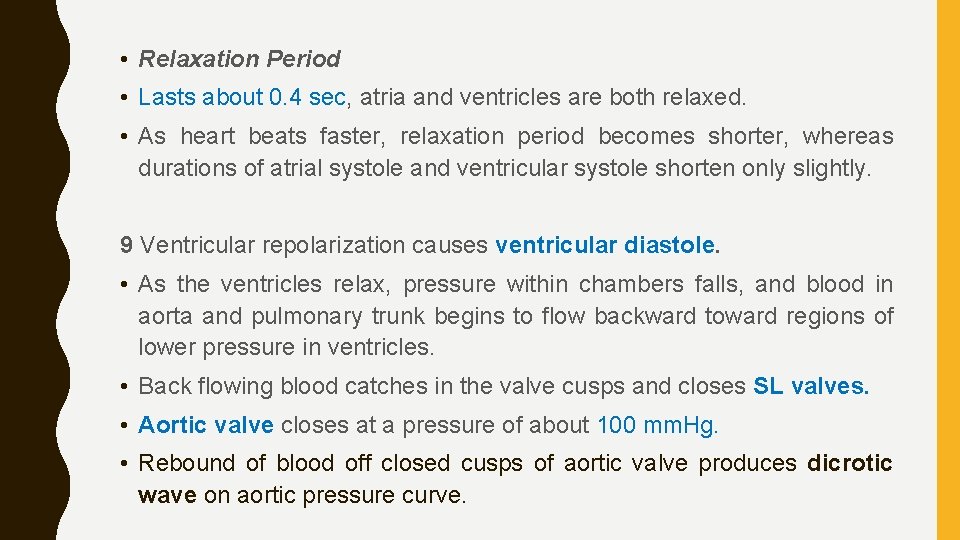
• Relaxation Period • Lasts about 0. 4 sec, atria and ventricles are both relaxed. • As heart beats faster, relaxation period becomes shorter, whereas durations of atrial systole and ventricular systole shorten only slightly. 9 Ventricular repolarization causes ventricular diastole. • As the ventricles relax, pressure within chambers falls, and blood in aorta and pulmonary trunk begins to flow backward toward regions of lower pressure in ventricles. • Back flowing blood catches in the valve cusps and closes SL valves. • Aortic valve closes at a pressure of about 100 mm. Hg. • Rebound of blood off closed cusps of aortic valve produces dicrotic wave on aortic pressure curve.
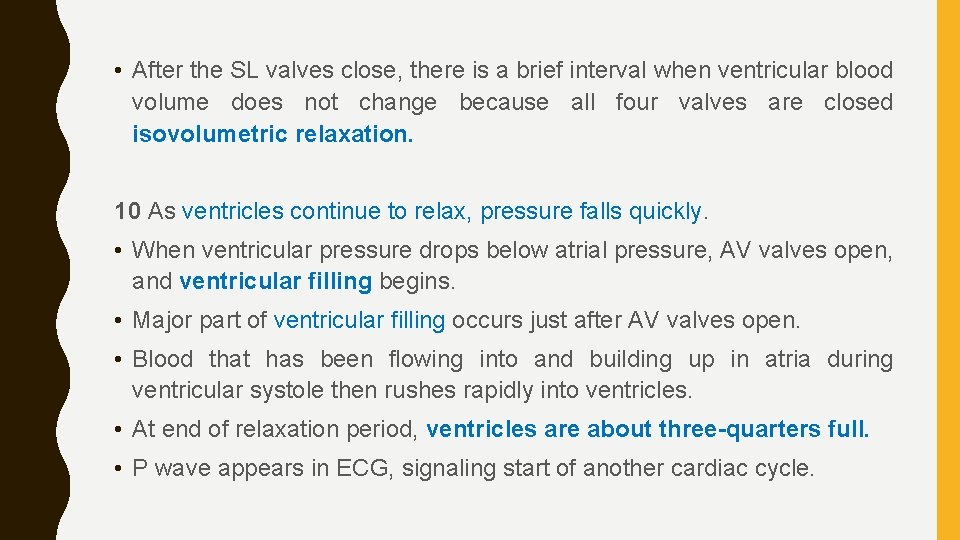
• After the SL valves close, there is a brief interval when ventricular blood volume does not change because all four valves are closed isovolumetric relaxation. 10 As ventricles continue to relax, pressure falls quickly. • When ventricular pressure drops below atrial pressure, AV valves open, and ventricular filling begins. • Major part of ventricular filling occurs just after AV valves open. • Blood that has been flowing into and building up in atria during ventricular systole then rushes rapidly into ventricles. • At end of relaxation period, ventricles are about three-quarters full. • P wave appears in ECG, signaling start of another cardiac cycle.
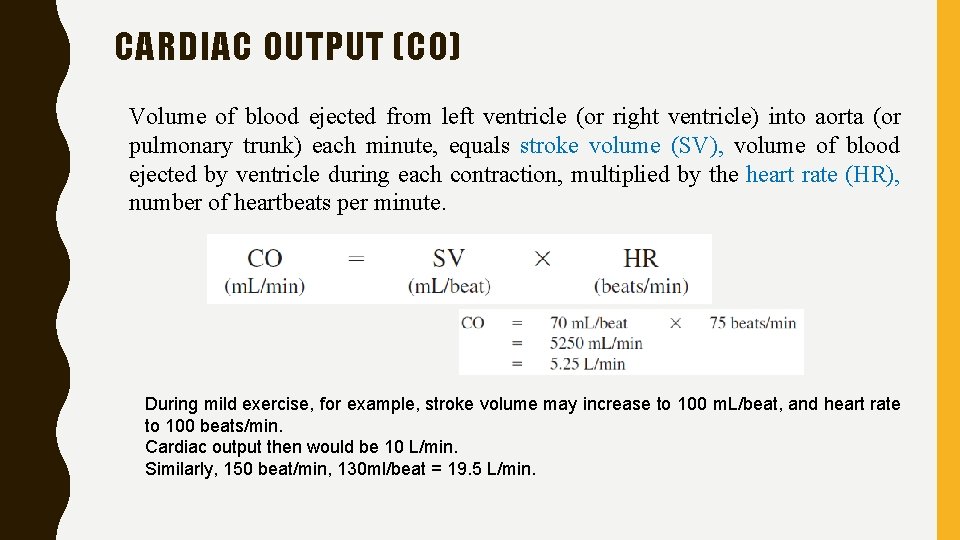
CARDIAC OUTPUT (CO) Volume of blood ejected from left ventricle (or right ventricle) into aorta (or pulmonary trunk) each minute, equals stroke volume (SV), volume of blood ejected by ventricle during each contraction, multiplied by the heart rate (HR), number of heartbeats per minute. During mild exercise, for example, stroke volume may increase to 100 m. L/beat, and heart rate to 100 beats/min. Cardiac output then would be 10 L/min. Similarly, 150 beat/min, 130 ml/beat = 19. 5 L/min.
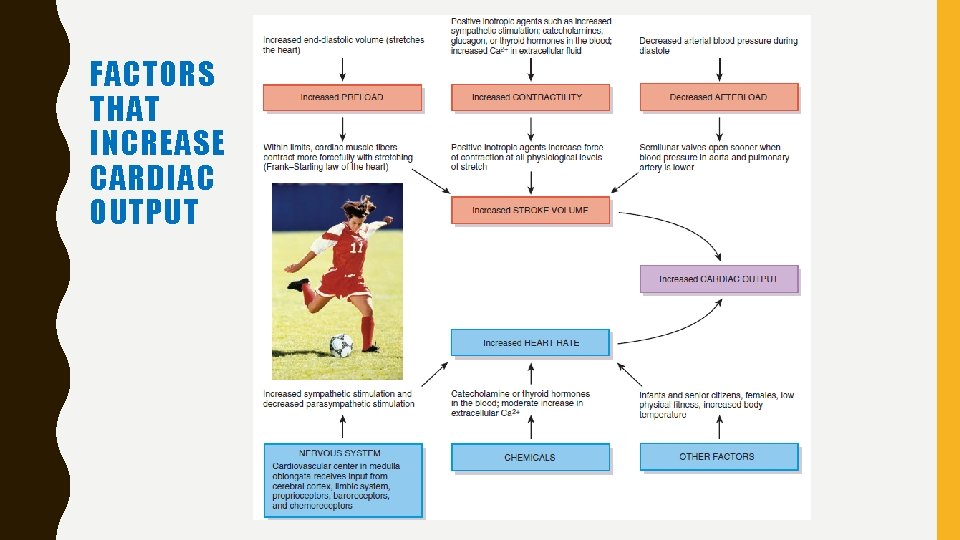
FACTORS THAT INCREASE CARDIAC OUTPUT
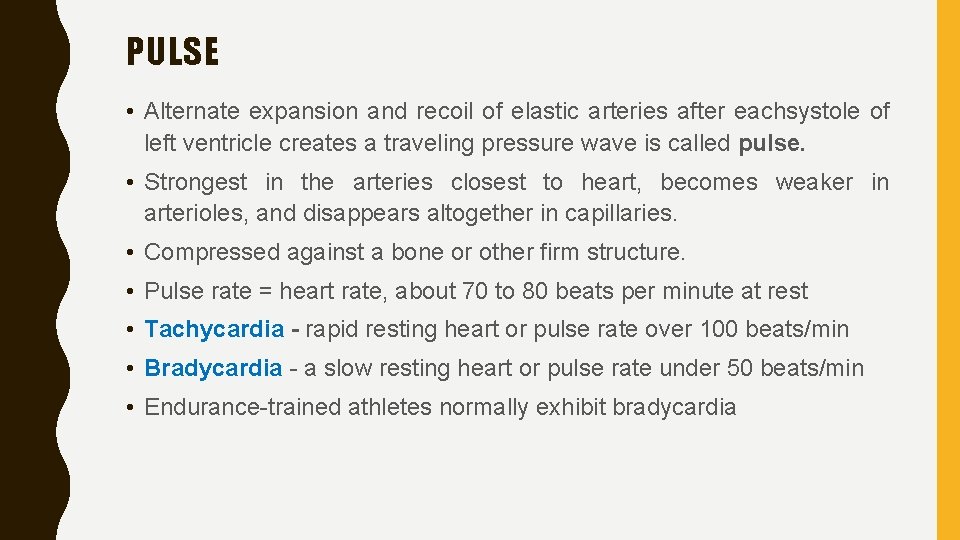
PULSE • Alternate expansion and recoil of elastic arteries after eachsystole of left ventricle creates a traveling pressure wave is called pulse. • Strongest in the arteries closest to heart, becomes weaker in arterioles, and disappears altogether in capillaries. • Compressed against a bone or other firm structure. • Pulse rate = heart rate, about 70 to 80 beats per minute at rest • Tachycardia - rapid resting heart or pulse rate over 100 beats/min • Bradycardia - a slow resting heart or pulse rate under 50 beats/min • Endurance-trained athletes normally exhibit bradycardia
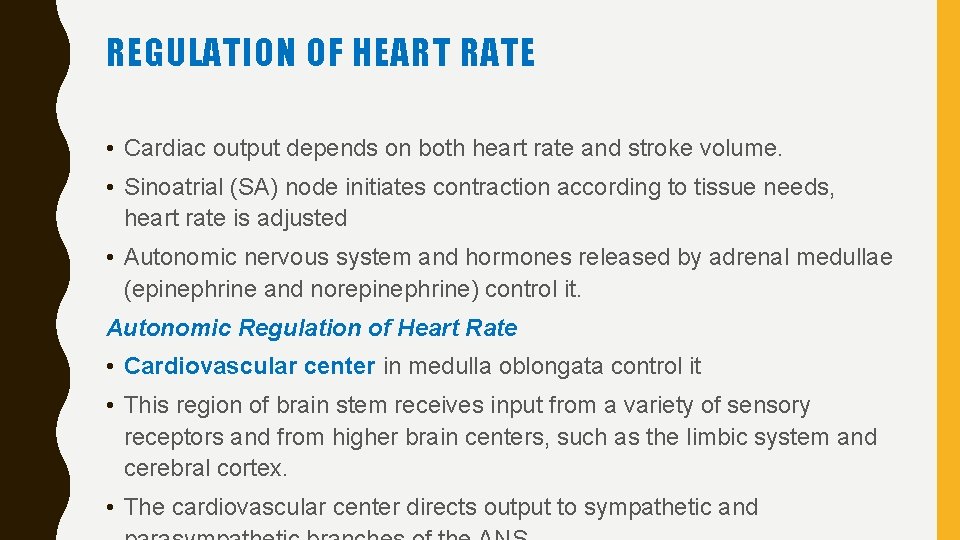
REGULATION OF HEART RATE • Cardiac output depends on both heart rate and stroke volume. • Sinoatrial (SA) node initiates contraction according to tissue needs, heart rate is adjusted • Autonomic nervous system and hormones released by adrenal medullae (epinephrine and norepinephrine) control it. Autonomic Regulation of Heart Rate • Cardiovascular center in medulla oblongata control it • This region of brain stem receives input from a variety of sensory receptors and from higher brain centers, such as the limbic system and cerebral cortex. • The cardiovascular center directs output to sympathetic and
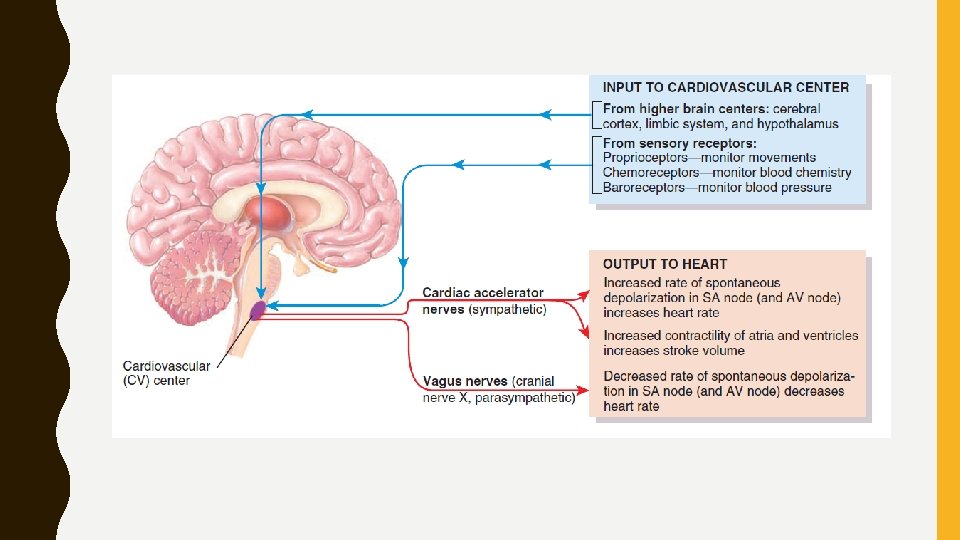
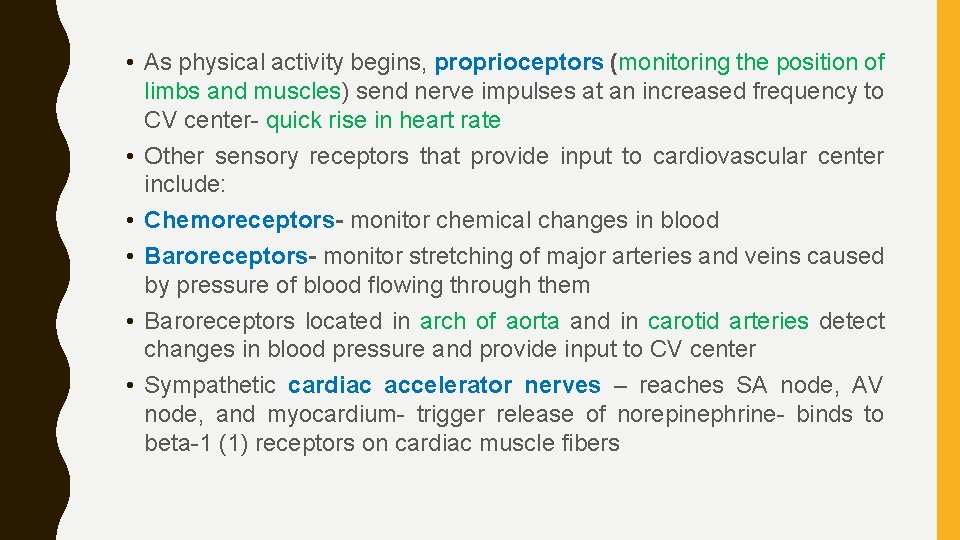
• As physical activity begins, proprioceptors (monitoring the position of limbs and muscles) send nerve impulses at an increased frequency to CV center- quick rise in heart rate • Other sensory receptors that provide input to cardiovascular center include: • Chemoreceptors- monitor chemical changes in blood • Baroreceptors- monitor stretching of major arteries and veins caused by pressure of blood flowing through them • Baroreceptors located in arch of aorta and in carotid arteries detect changes in blood pressure and provide input to CV center • Sympathetic cardiac accelerator nerves – reaches SA node, AV node, and myocardium- trigger release of norepinephrine- binds to beta-1 (1) receptors on cardiac muscle fibers
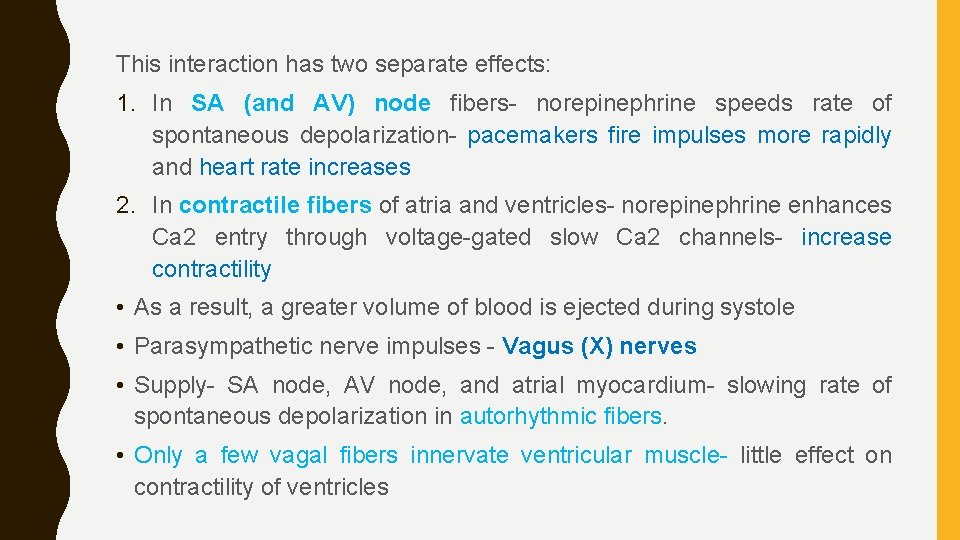
This interaction has two separate effects: 1. In SA (and AV) node fibers- norepinephrine speeds rate of spontaneous depolarization- pacemakers fire impulses more rapidly and heart rate increases 2. In contractile fibers of atria and ventricles- norepinephrine enhances Ca 2 entry through voltage-gated slow Ca 2 channels- increase contractility • As a result, a greater volume of blood is ejected during systole • Parasympathetic nerve impulses - Vagus (X) nerves • Supply- SA node, AV node, and atrial myocardium- slowing rate of spontaneous depolarization in autorhythmic fibers. • Only a few vagal fibers innervate ventricular muscle- little effect on contractility of ventricles
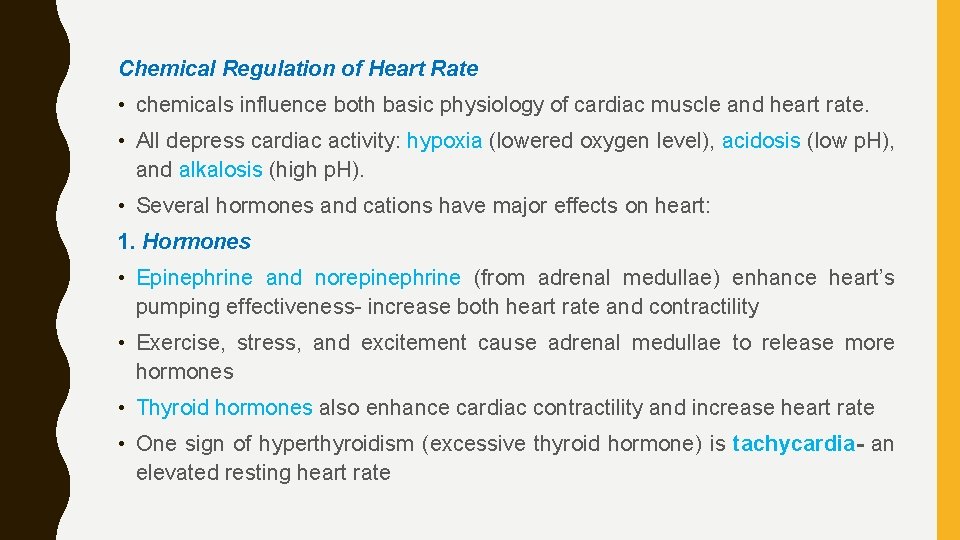
Chemical Regulation of Heart Rate • chemicals influence both basic physiology of cardiac muscle and heart rate. • All depress cardiac activity: hypoxia (lowered oxygen level), acidosis (low p. H), and alkalosis (high p. H). • Several hormones and cations have major effects on heart: 1. Hormones • Epinephrine and norepinephrine (from adrenal medullae) enhance heart’s pumping effectiveness- increase both heart rate and contractility • Exercise, stress, and excitement cause adrenal medullae to release more hormones • Thyroid hormones also enhance cardiac contractility and increase heart rate • One sign of hyperthyroidism (excessive thyroid hormone) is tachycardia- an elevated resting heart rate
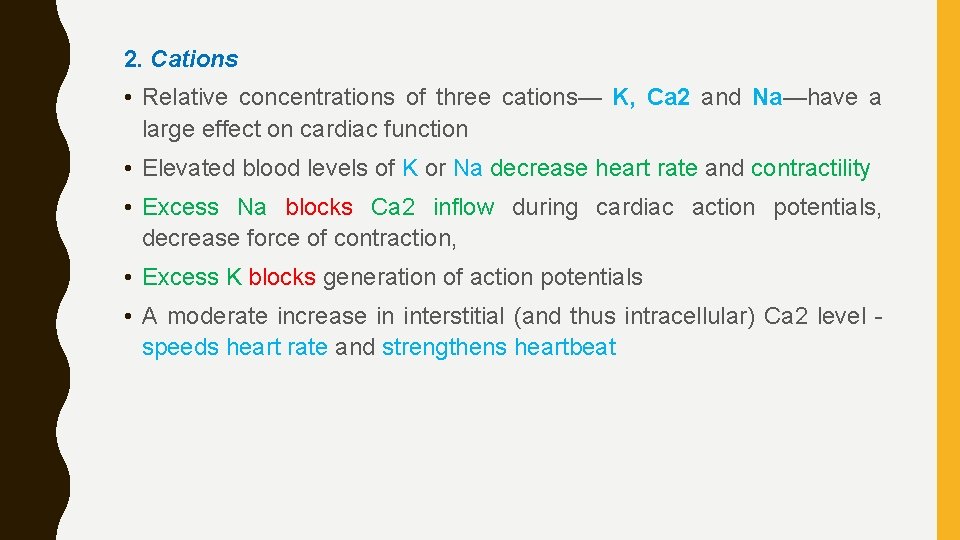
2. Cations • Relative concentrations of three cations— K, Ca 2 and Na—have a large effect on cardiac function • Elevated blood levels of K or Na decrease heart rate and contractility • Excess Na blocks Ca 2 inflow during cardiac action potentials, decrease force of contraction, • Excess K blocks generation of action potentials • A moderate increase in interstitial (and thus intracellular) Ca 2 level speeds heart rate and strengthens heartbeat
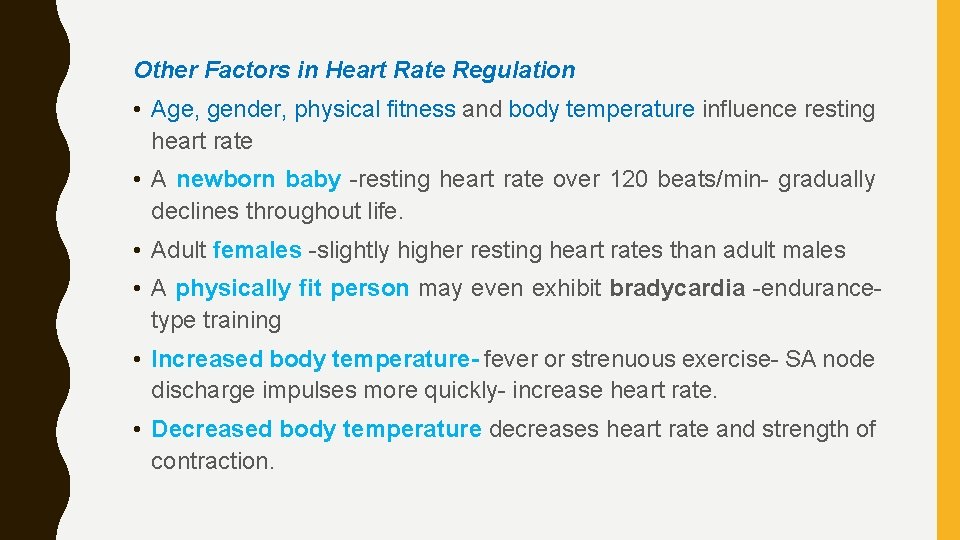
Other Factors in Heart Rate Regulation • Age, gender, physical fitness and body temperature influence resting heart rate • A newborn baby -resting heart rate over 120 beats/min- gradually declines throughout life. • Adult females -slightly higher resting heart rates than adult males • A physically fit person may even exhibit bradycardia -endurancetype training • Increased body temperature- fever or strenuous exercise- SA node discharge impulses more quickly- increase heart rate. • Decreased body temperature decreases heart rate and strength of contraction.
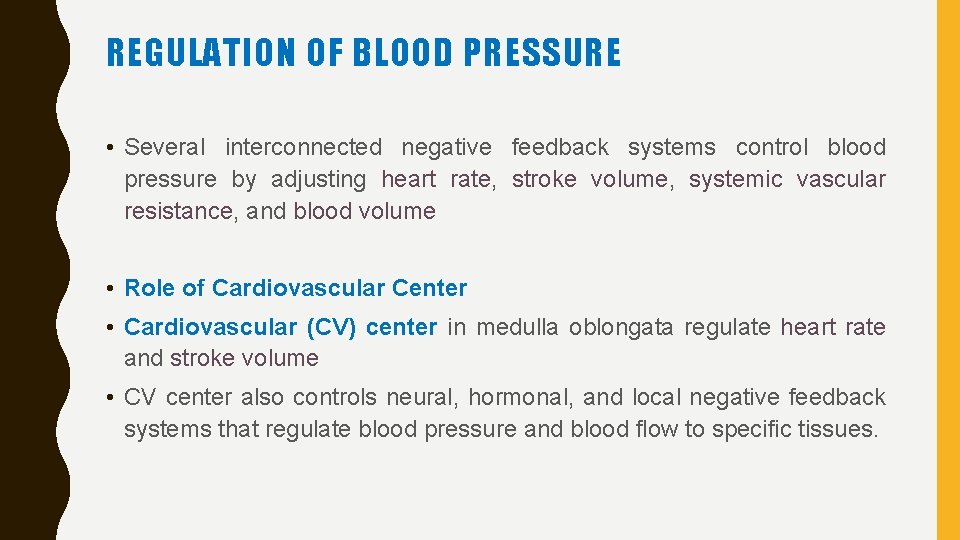
REGULATION OF BLOOD PRESSURE • Several interconnected negative feedback systems control blood pressure by adjusting heart rate, stroke volume, systemic vascular resistance, and blood volume • Role of Cardiovascular Center • Cardiovascular (CV) center in medulla oblongata regulate heart rate and stroke volume • CV center also controls neural, hormonal, and local negative feedback systems that regulate blood pressure and blood flow to specific tissues.
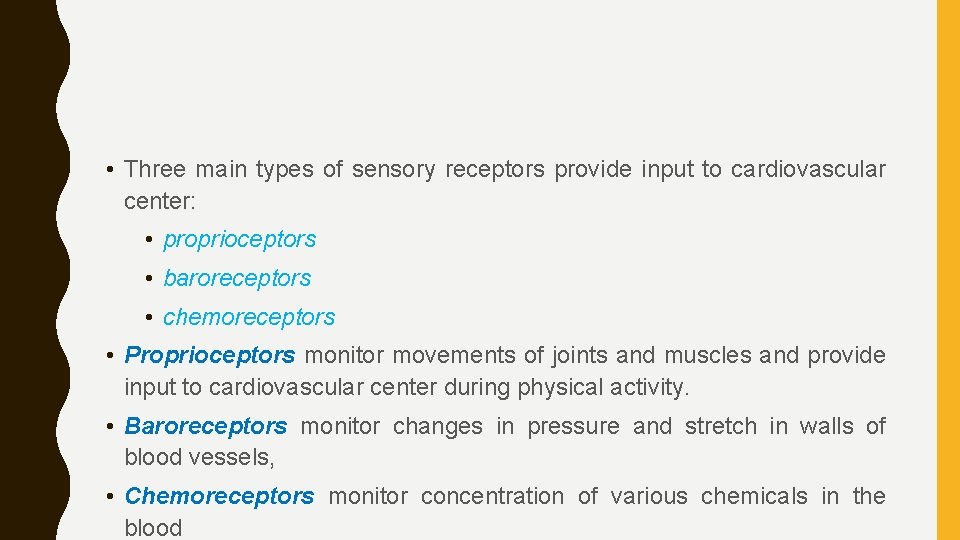
• Three main types of sensory receptors provide input to cardiovascular center: • proprioceptors • baroreceptors • chemoreceptors • Proprioceptors monitor movements of joints and muscles and provide input to cardiovascular center during physical activity. • Baroreceptors monitor changes in pressure and stretch in walls of blood vessels, • Chemoreceptors monitor concentration of various chemicals in the blood
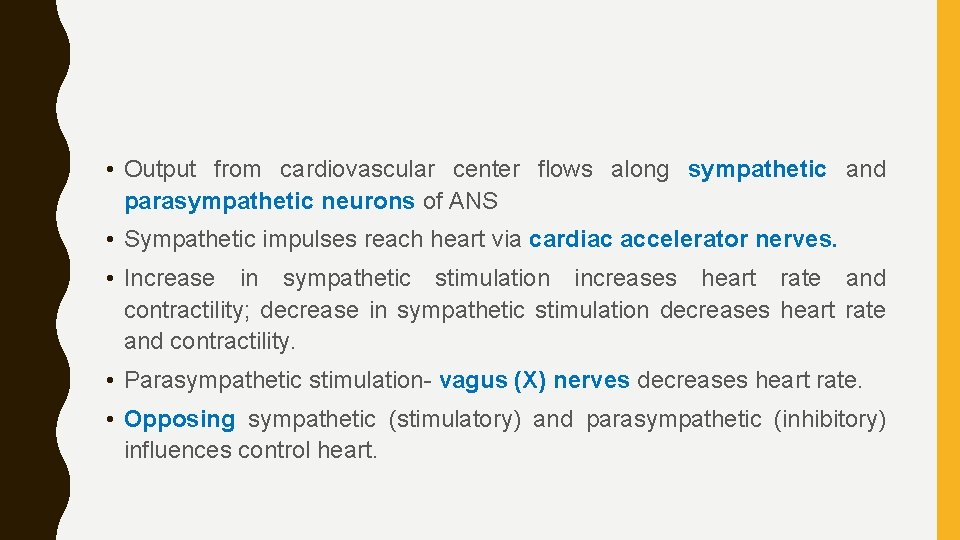
• Output from cardiovascular center flows along sympathetic and parasympathetic neurons of ANS • Sympathetic impulses reach heart via cardiac accelerator nerves. • Increase in sympathetic stimulation increases heart rate and contractility; decrease in sympathetic stimulation decreases heart rate and contractility. • Parasympathetic stimulation- vagus (X) nerves decreases heart rate. • Opposing sympathetic (stimulatory) and parasympathetic (inhibitory) influences control heart.
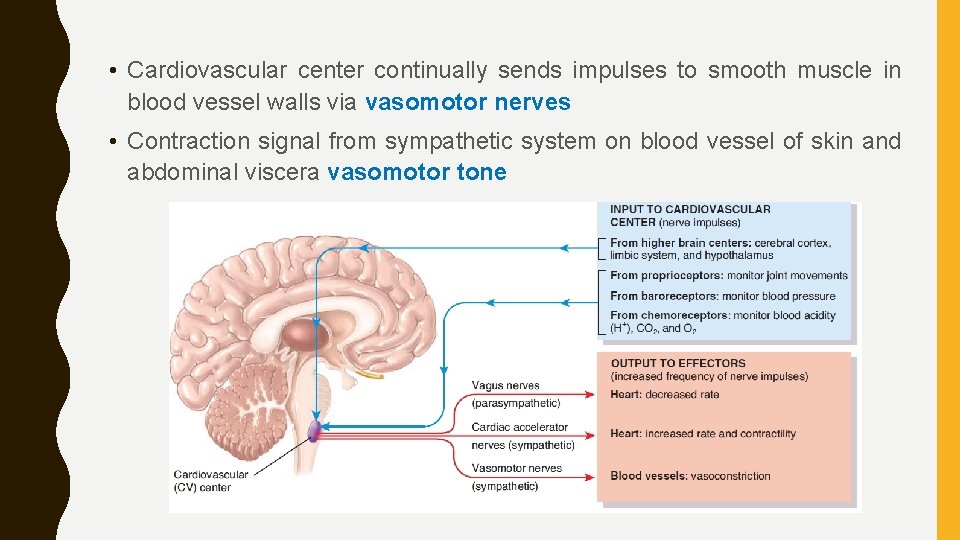
• Cardiovascular center continually sends impulses to smooth muscle in blood vessel walls via vasomotor nerves • Contraction signal from sympathetic system on blood vessel of skin and abdominal viscera vasomotor tone
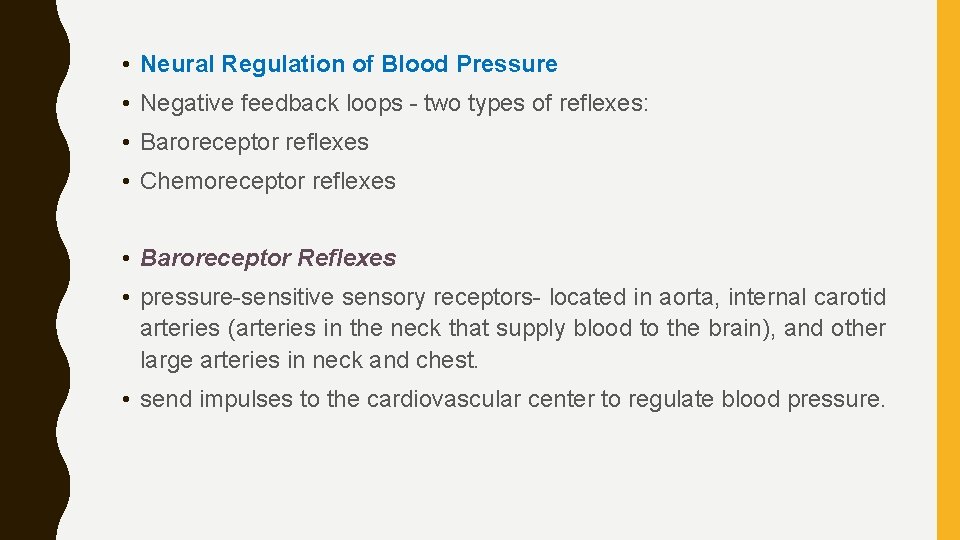
• Neural Regulation of Blood Pressure • Negative feedback loops - two types of reflexes: • Baroreceptor reflexes • Chemoreceptor reflexes • Baroreceptor Reflexes • pressure-sensitive sensory receptors- located in aorta, internal carotid arteries (arteries in the neck that supply blood to the brain), and other large arteries in neck and chest. • send impulses to the cardiovascular center to regulate blood pressure.
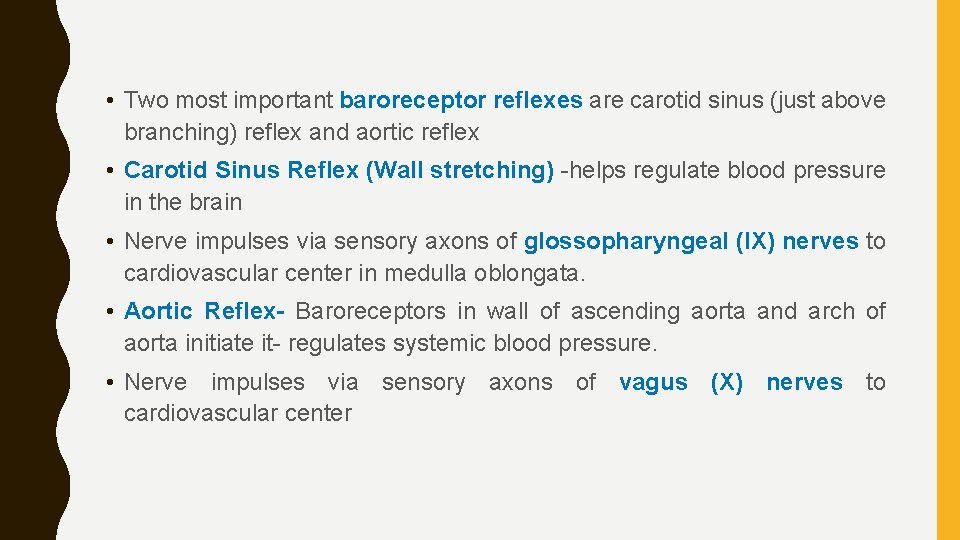
• Two most important baroreceptor reflexes are carotid sinus (just above branching) reflex and aortic reflex • Carotid Sinus Reflex (Wall stretching) -helps regulate blood pressure in the brain • Nerve impulses via sensory axons of glossopharyngeal (IX) nerves to cardiovascular center in medulla oblongata. • Aortic Reflex- Baroreceptors in wall of ascending aorta and arch of aorta initiate it- regulates systemic blood pressure. • Nerve impulses via sensory axons of vagus (X) nerves to cardiovascular center
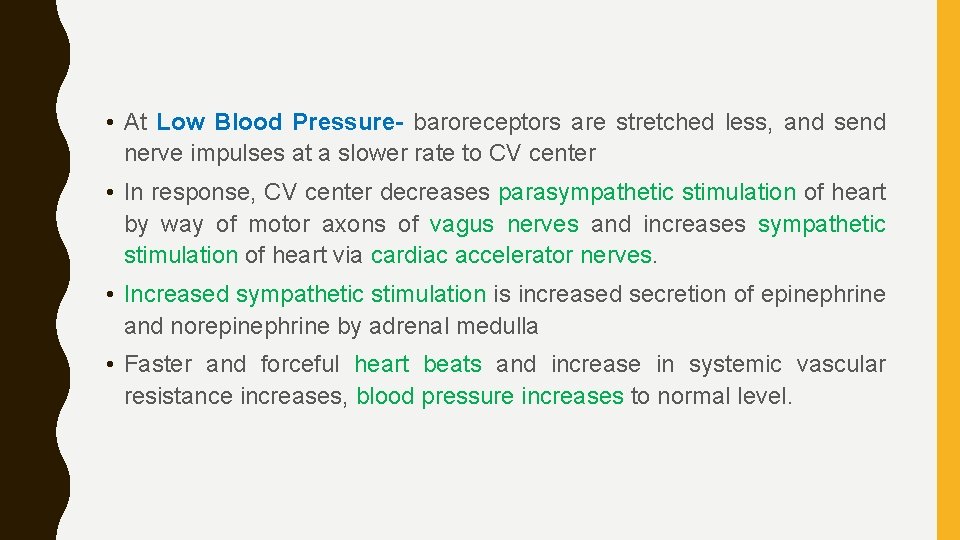
• At Low Blood Pressure- baroreceptors are stretched less, and send nerve impulses at a slower rate to CV center • In response, CV center decreases parasympathetic stimulation of heart by way of motor axons of vagus nerves and increases sympathetic stimulation of heart via cardiac accelerator nerves. • Increased sympathetic stimulation is increased secretion of epinephrine and norepinephrine by adrenal medulla • Faster and forceful heart beats and increase in systemic vascular resistance increases, blood pressure increases to normal level.
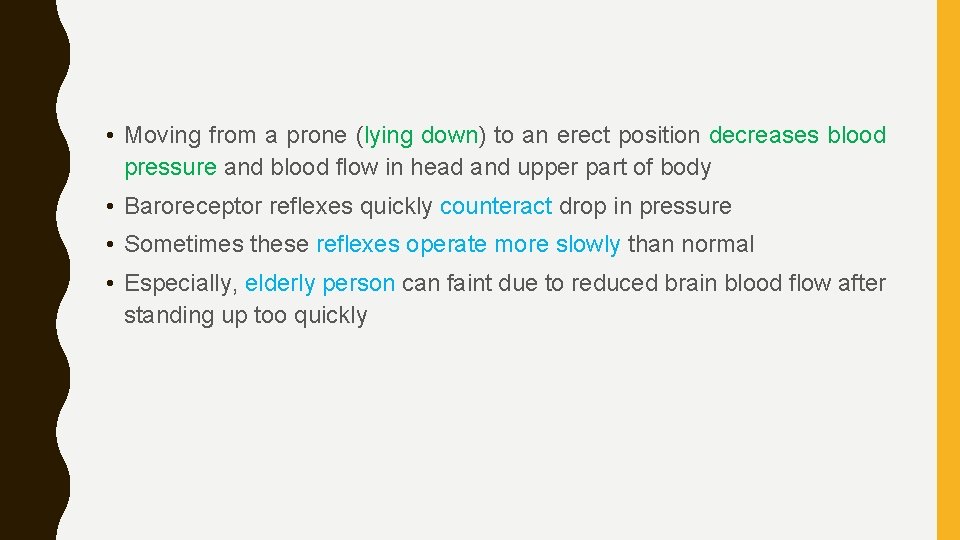
• Moving from a prone (lying down) to an erect position decreases blood pressure and blood flow in head and upper part of body • Baroreceptor reflexes quickly counteract drop in pressure • Sometimes these reflexes operate more slowly than normal • Especially, elderly person can faint due to reduced brain blood flow after standing up too quickly
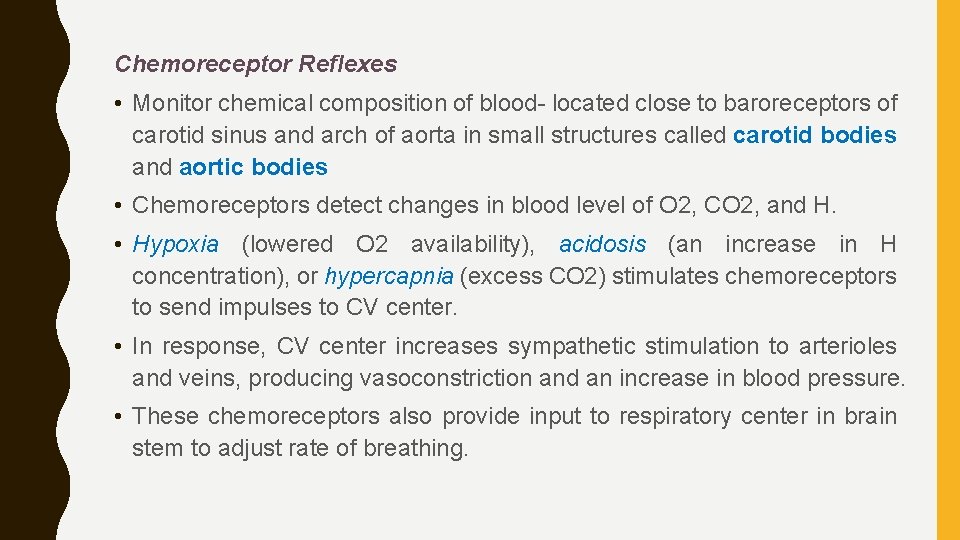
Chemoreceptor Reflexes • Monitor chemical composition of blood- located close to baroreceptors of carotid sinus and arch of aorta in small structures called carotid bodies and aortic bodies • Chemoreceptors detect changes in blood level of O 2, CO 2, and H. • Hypoxia (lowered O 2 availability), acidosis (an increase in H concentration), or hypercapnia (excess CO 2) stimulates chemoreceptors to send impulses to CV center. • In response, CV center increases sympathetic stimulation to arterioles and veins, producing vasoconstriction and an increase in blood pressure. • These chemoreceptors also provide input to respiratory center in brain stem to adjust rate of breathing.
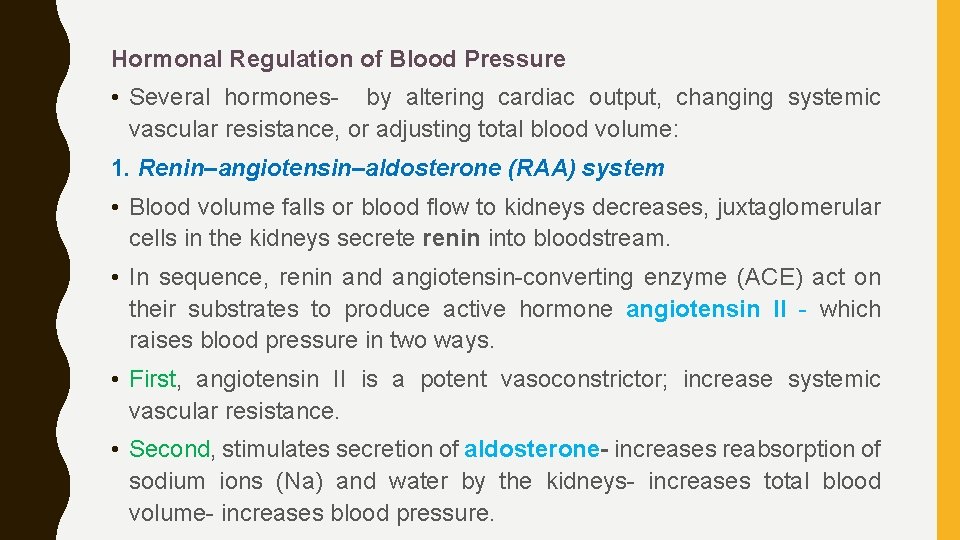
Hormonal Regulation of Blood Pressure • Several hormones- by altering cardiac output, changing systemic vascular resistance, or adjusting total blood volume: 1. Renin–angiotensin–aldosterone (RAA) system • Blood volume falls or blood flow to kidneys decreases, juxtaglomerular cells in the kidneys secrete renin into bloodstream. • In sequence, renin and angiotensin-converting enzyme (ACE) act on their substrates to produce active hormone angiotensin II - which raises blood pressure in two ways. • First, angiotensin II is a potent vasoconstrictor; increase systemic vascular resistance. • Second, stimulates secretion of aldosterone- increases reabsorption of sodium ions (Na) and water by the kidneys- increases total blood volume- increases blood pressure.
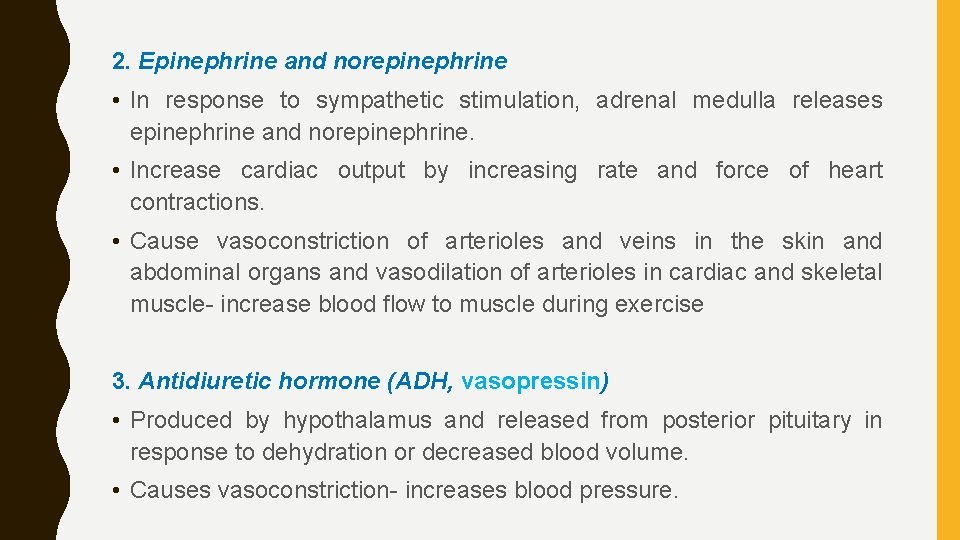
2. Epinephrine and norepinephrine • In response to sympathetic stimulation, adrenal medulla releases epinephrine and norepinephrine. • Increase cardiac output by increasing rate and force of heart contractions. • Cause vasoconstriction of arterioles and veins in the skin and abdominal organs and vasodilation of arterioles in cardiac and skeletal muscle- increase blood flow to muscle during exercise 3. Antidiuretic hormone (ADH, vasopressin) • Produced by hypothalamus and released from posterior pituitary in response to dehydration or decreased blood volume. • Causes vasoconstriction- increases blood pressure.
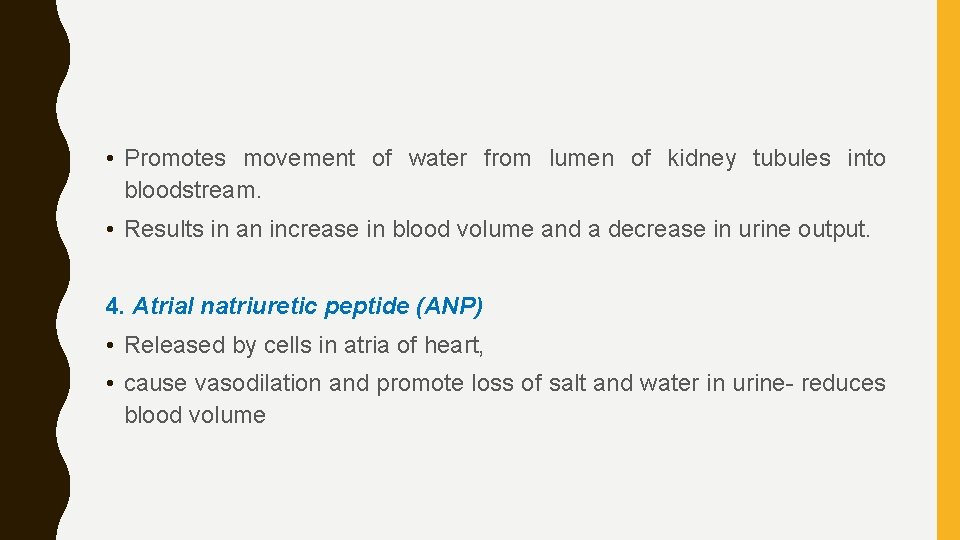
• Promotes movement of water from lumen of kidney tubules into bloodstream. • Results in an increase in blood volume and a decrease in urine output. 4. Atrial natriuretic peptide (ANP) • Released by cells in atria of heart, • cause vasodilation and promote loss of salt and water in urine- reduces blood volume
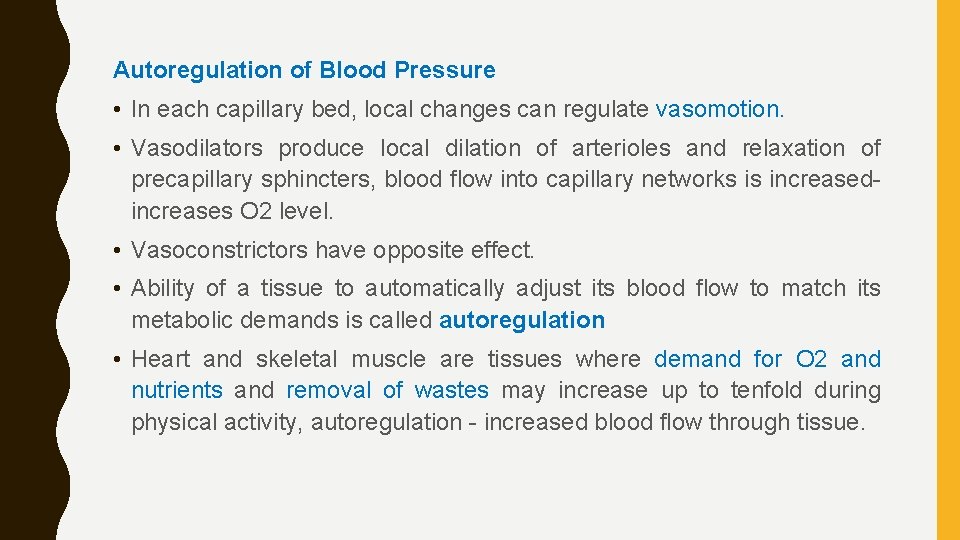
Autoregulation of Blood Pressure • In each capillary bed, local changes can regulate vasomotion. • Vasodilators produce local dilation of arterioles and relaxation of precapillary sphincters, blood flow into capillary networks is increasedincreases O 2 level. • Vasoconstrictors have opposite effect. • Ability of a tissue to automatically adjust its blood flow to match its metabolic demands is called autoregulation • Heart and skeletal muscle are tissues where demand for O 2 and nutrients and removal of wastes may increase up to tenfold during physical activity, autoregulation - increased blood flow through tissue.
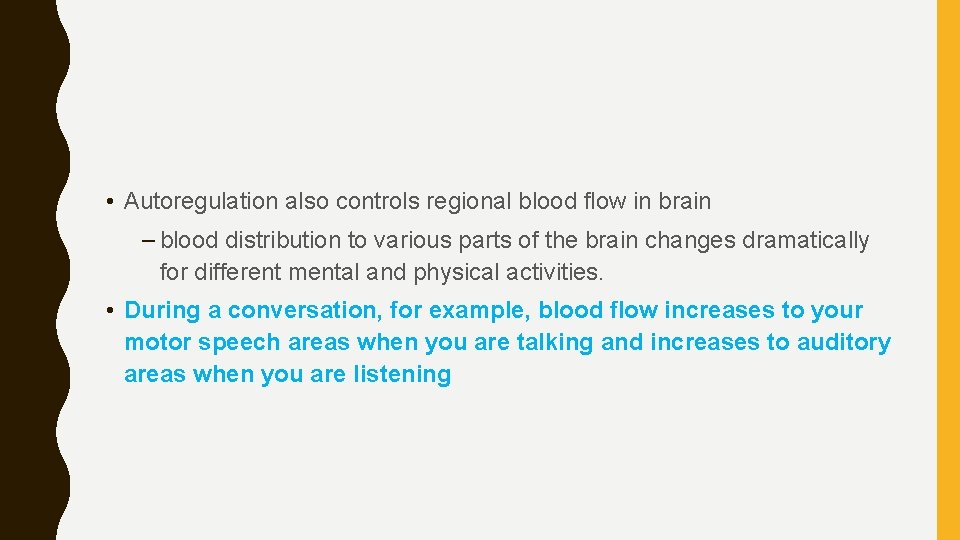
• Autoregulation also controls regional blood flow in brain – blood distribution to various parts of the brain changes dramatically for different mental and physical activities. • During a conversation, for example, blood flow increases to your motor speech areas when you are talking and increases to auditory areas when you are listening
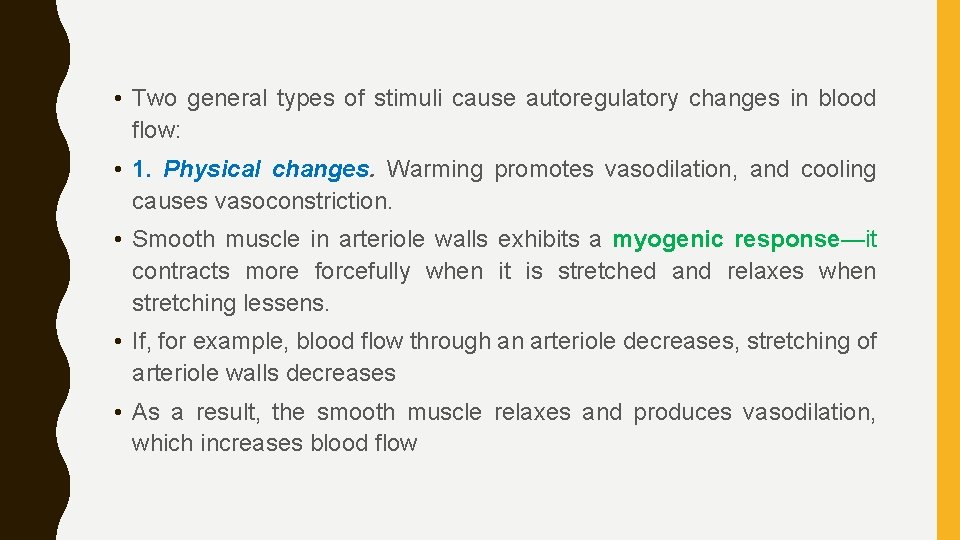
• Two general types of stimuli cause autoregulatory changes in blood flow: • 1. Physical changes. Warming promotes vasodilation, and cooling causes vasoconstriction. • Smooth muscle in arteriole walls exhibits a myogenic response—it contracts more forcefully when it is stretched and relaxes when stretching lessens. • If, for example, blood flow through an arteriole decreases, stretching of arteriole walls decreases • As a result, the smooth muscle relaxes and produces vasodilation, which increases blood flow
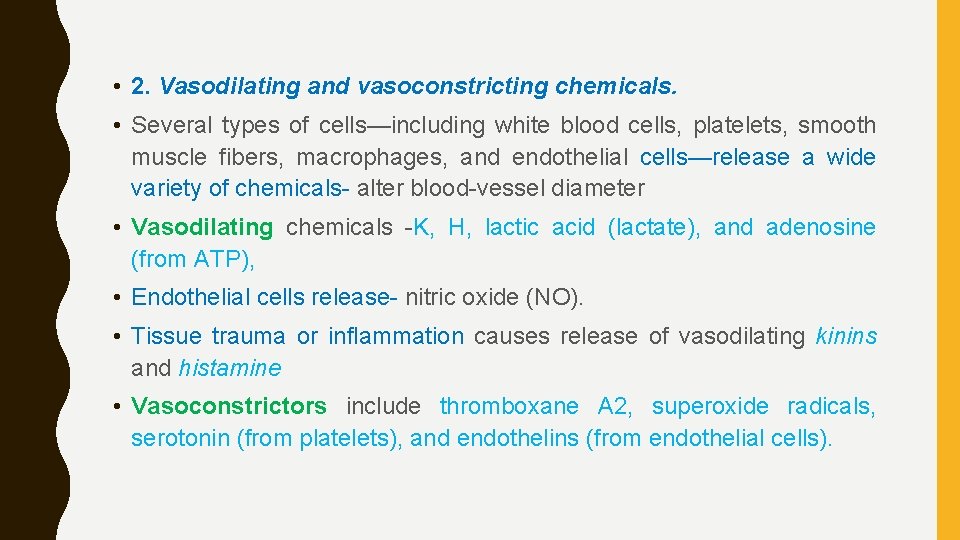
• 2. Vasodilating and vasoconstricting chemicals. • Several types of cells—including white blood cells, platelets, smooth muscle fibers, macrophages, and endothelial cells—release a wide variety of chemicals- alter blood-vessel diameter • Vasodilating chemicals -K, H, lactic acid (lactate), and adenosine (from ATP), • Endothelial cells release- nitric oxide (NO). • Tissue trauma or inflammation causes release of vasodilating kinins and histamine • Vasoconstrictors include thromboxane A 2, superoxide radicals, serotonin (from platelets), and endothelins (from endothelial cells).
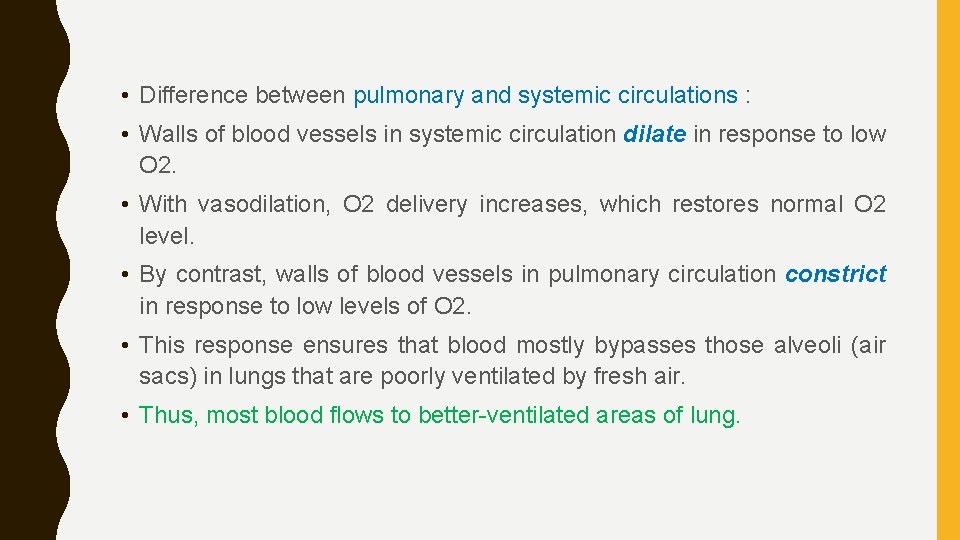
• Difference between pulmonary and systemic circulations : • Walls of blood vessels in systemic circulation dilate in response to low O 2. • With vasodilation, O 2 delivery increases, which restores normal O 2 level. • By contrast, walls of blood vessels in pulmonary circulation constrict in response to low levels of O 2. • This response ensures that blood mostly bypasses those alveoli (air sacs) in lungs that are poorly ventilated by fresh air. • Thus, most blood flows to better-ventilated areas of lung.
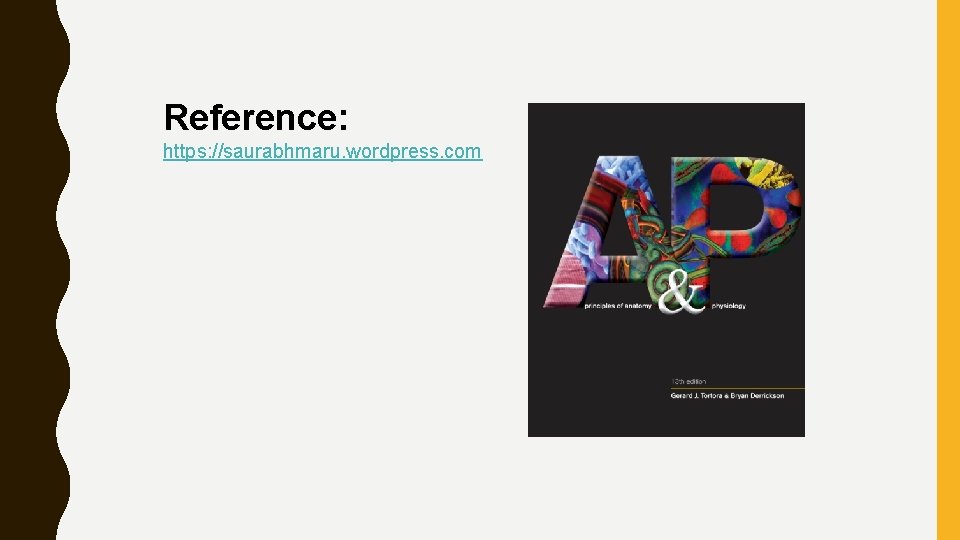
Reference: https: //saurabhmaru. wordpress. com