Exploiting Protein Cage Dynamics to Engineer Active Nanostructures
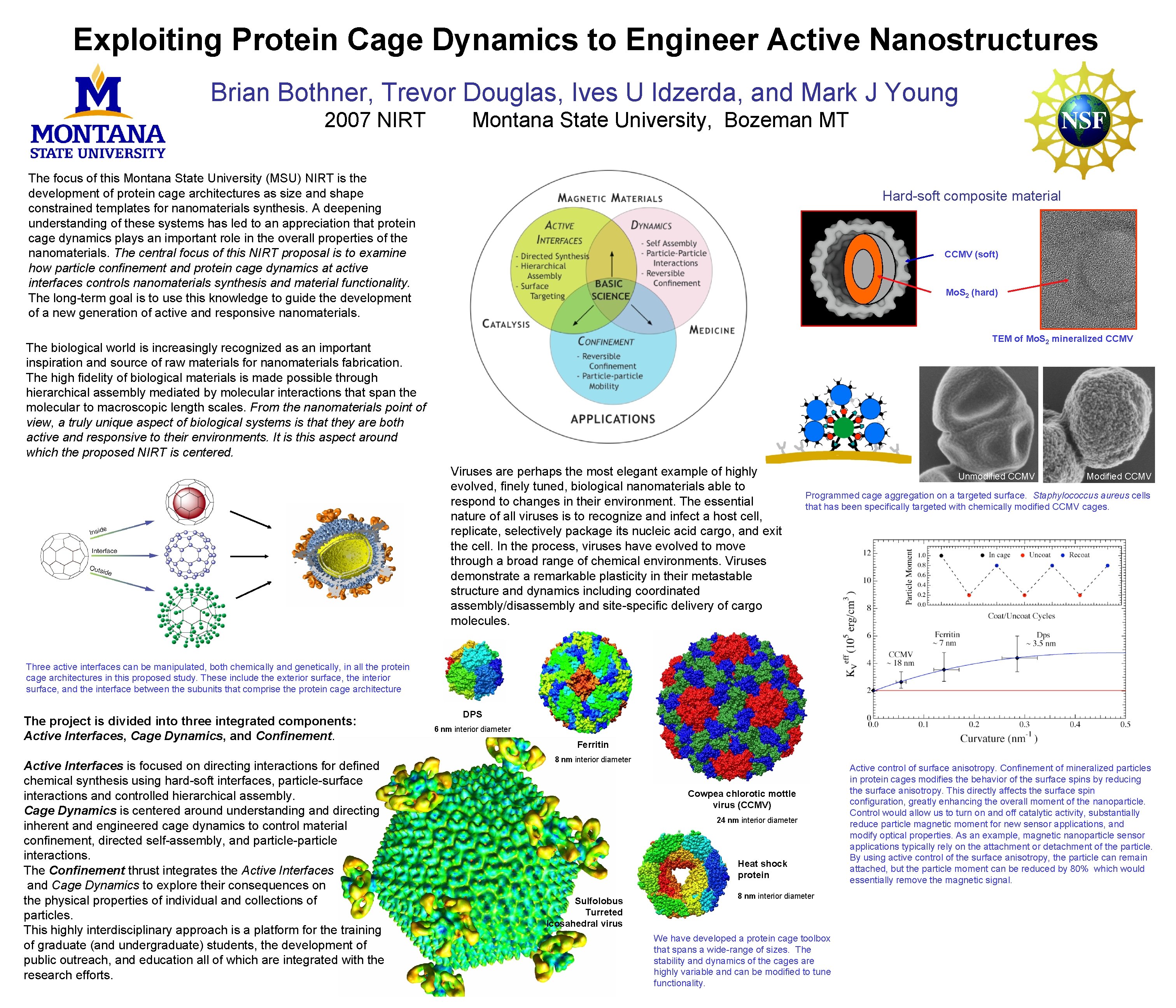
- Slides: 1
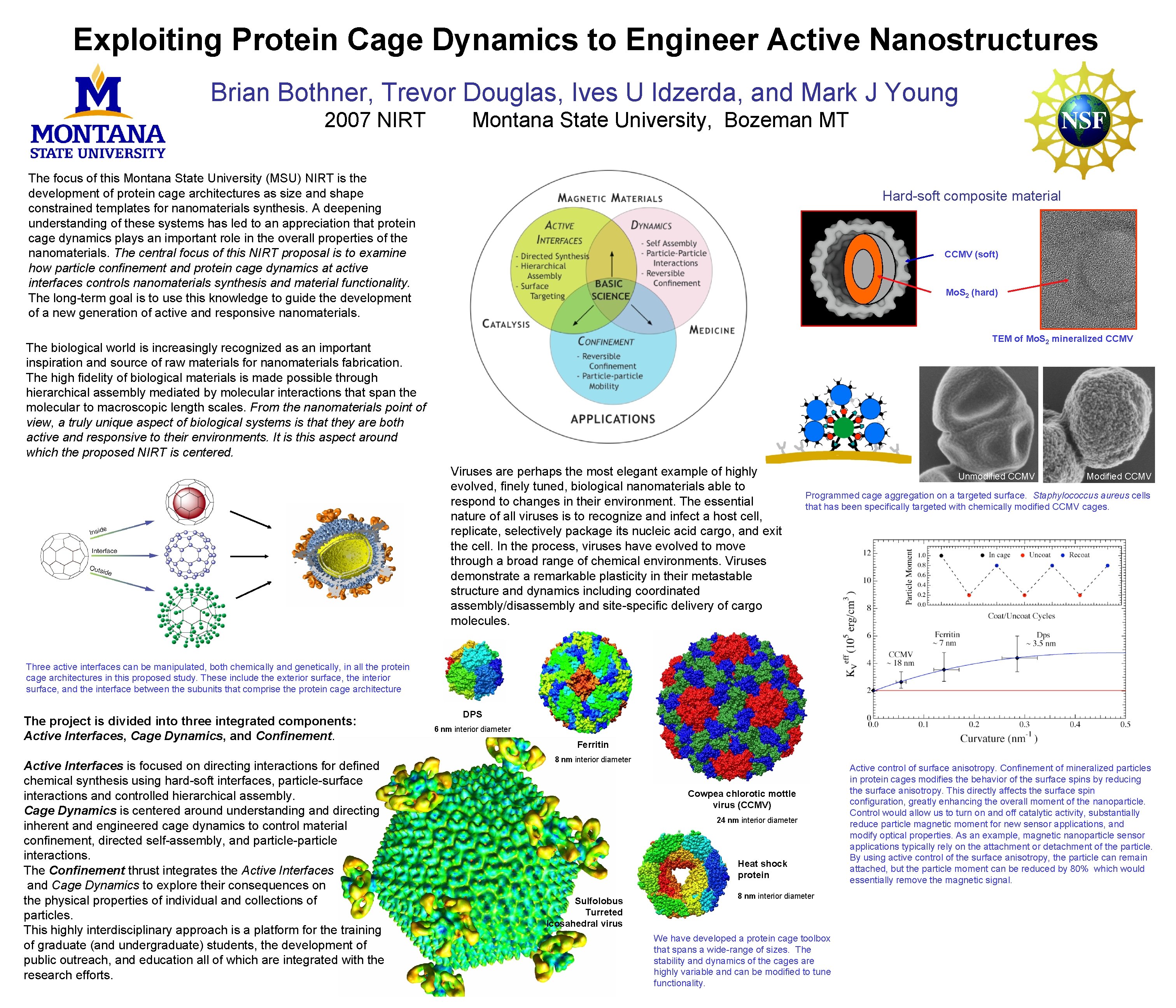
Exploiting Protein Cage Dynamics to Engineer Active Nanostructures Brian Bothner, Trevor Douglas, Ives U Idzerda, and Mark J Young 2007 NIRT Montana State University, Bozeman MT The focus of this Montana State University (MSU) NIRT is the development of protein cage architectures as size and shape constrained templates for nanomaterials synthesis. A deepening understanding of these systems has led to an appreciation that protein cage dynamics plays an important role in the overall properties of the nanomaterials. The central focus of this NIRT proposal is to examine how particle confinement and protein cage dynamics at active interfaces controls nanomaterials synthesis and material functionality. The long-term goal is to use this knowledge to guide the development of a new generation of active and responsive nanomaterials. Hard-soft composite material CCMV (soft) Mo. S 2 (hard) TEM of Mo. S 2 mineralized CCMV The biological world is increasingly recognized as an important inspiration and source of raw materials for nanomaterials fabrication. The high fidelity of biological materials is made possible through hierarchical assembly mediated by molecular interactions that span the molecular to macroscopic length scales. From the nanomaterials point of view, a truly unique aspect of biological systems is that they are both active and responsive to their environments. It is this aspect around which the proposed NIRT is centered. Viruses are perhaps the most elegant example of highly evolved, finely tuned, biological nanomaterials able to respond to changes in their environment. The essential nature of all viruses is to recognize and infect a host cell, replicate, selectively package its nucleic acid cargo, and exit the cell. In the process, viruses have evolved to move through a broad range of chemical environments. Viruses demonstrate a remarkable plasticity in their metastable structure and dynamics including coordinated assembly/disassembly and site-specific delivery of cargo molecules. Unmodified CCMV Modified CCMV Programmed cage aggregation on a targeted surface. Staphylococcus aureus cells that has been specifically targeted with chemically modified CCMV cages. Three active interfaces can be manipulated, both chemically and genetically, in all the protein cage architectures in this proposed study. These include the exterior surface, the interior surface, and the interface between the subunits that comprise the protein cage architecture The project is divided into three integrated components: Active Interfaces, Cage Dynamics, and Confinement. Active Interfaces is focused on directing interactions for defined chemical synthesis using hard-soft interfaces, particle-surface interactions and controlled hierarchical assembly. Cage Dynamics is centered around understanding and directing inherent and engineered cage dynamics to control material confinement, directed self-assembly, and particle-particle interactions. The Confinement thrust integrates the Active Interfaces and Cage Dynamics to explore their consequences on the physical properties of individual and collections of particles. This highly interdisciplinary approach is a platform for the training of graduate (and undergraduate) students, the development of public outreach, and education all of which are integrated with the research efforts. DPS 6 nm interior diameter Ferritin 8 nm interior diameter Cowpea chlorotic mottle virus (CCMV) 24 nm interior diameter Heat shock protein Sulfolobus Turreted Icosahedral virus 8 nm interior diameter We have developed a protein cage toolbox that spans a wide-range of sizes. The stability and dynamics of the cages are highly variable and can be modified to tune functionality. Active control of surface anisotropy. Confinement of mineralized particles in protein cages modifies the behavior of the surface spins by reducing the surface anisotropy. This directly affects the surface spin configuration, greatly enhancing the overall moment of the nanoparticle. Control would allow us to turn on and off catalytic activity, substantially reduce particle magnetic moment for new sensor applications, and modify optical properties. As an example, magnetic nanoparticle sensor applications typically rely on the attachment or detachment of the particle. By using active control of the surface anisotropy, the particle can remain attached, but the particle moment can be reduced by 80% which would essentially remove the magnetic signal.