Ethylene adsorption on crystalline and amorphous water ice
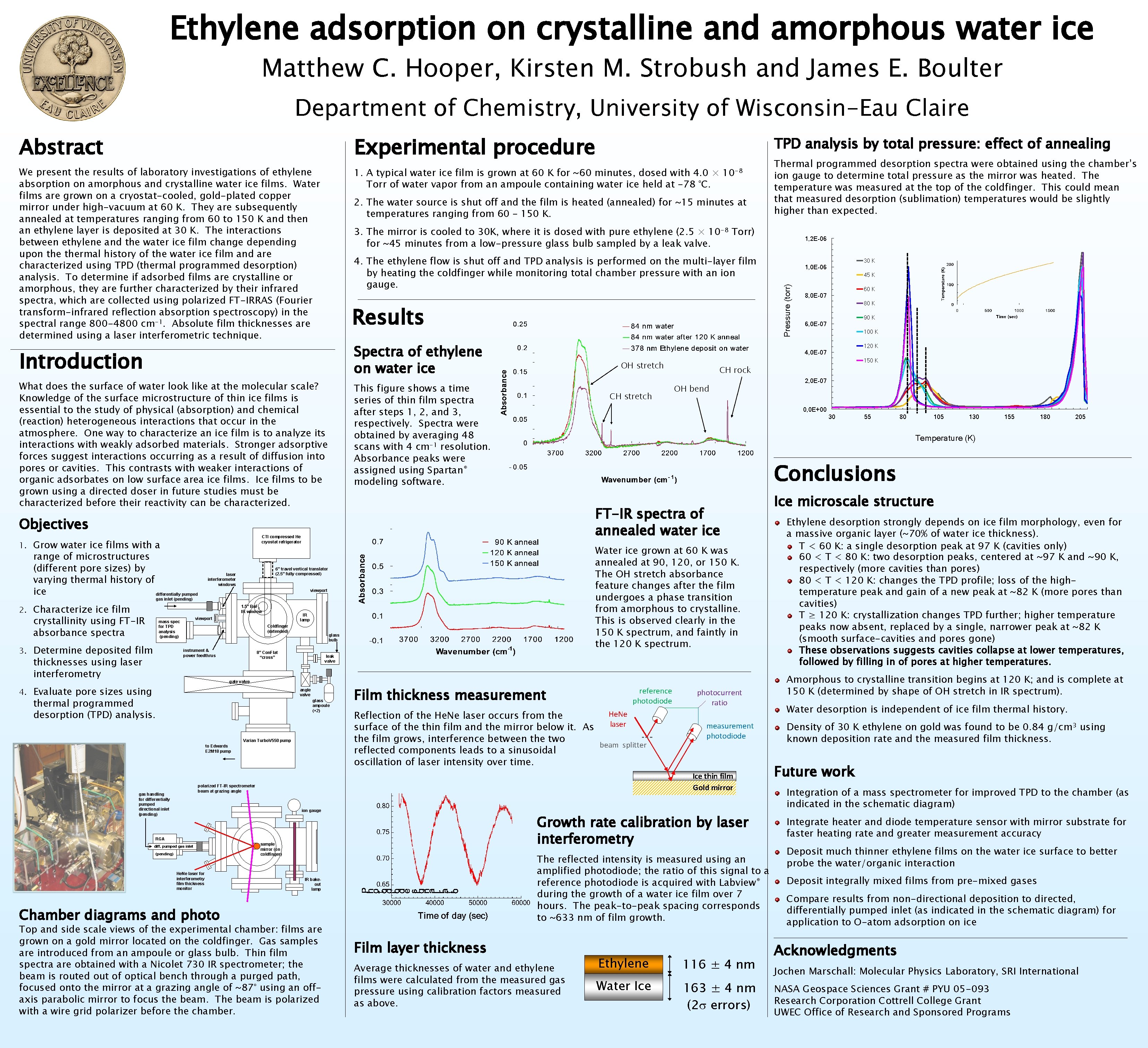
- Slides: 1
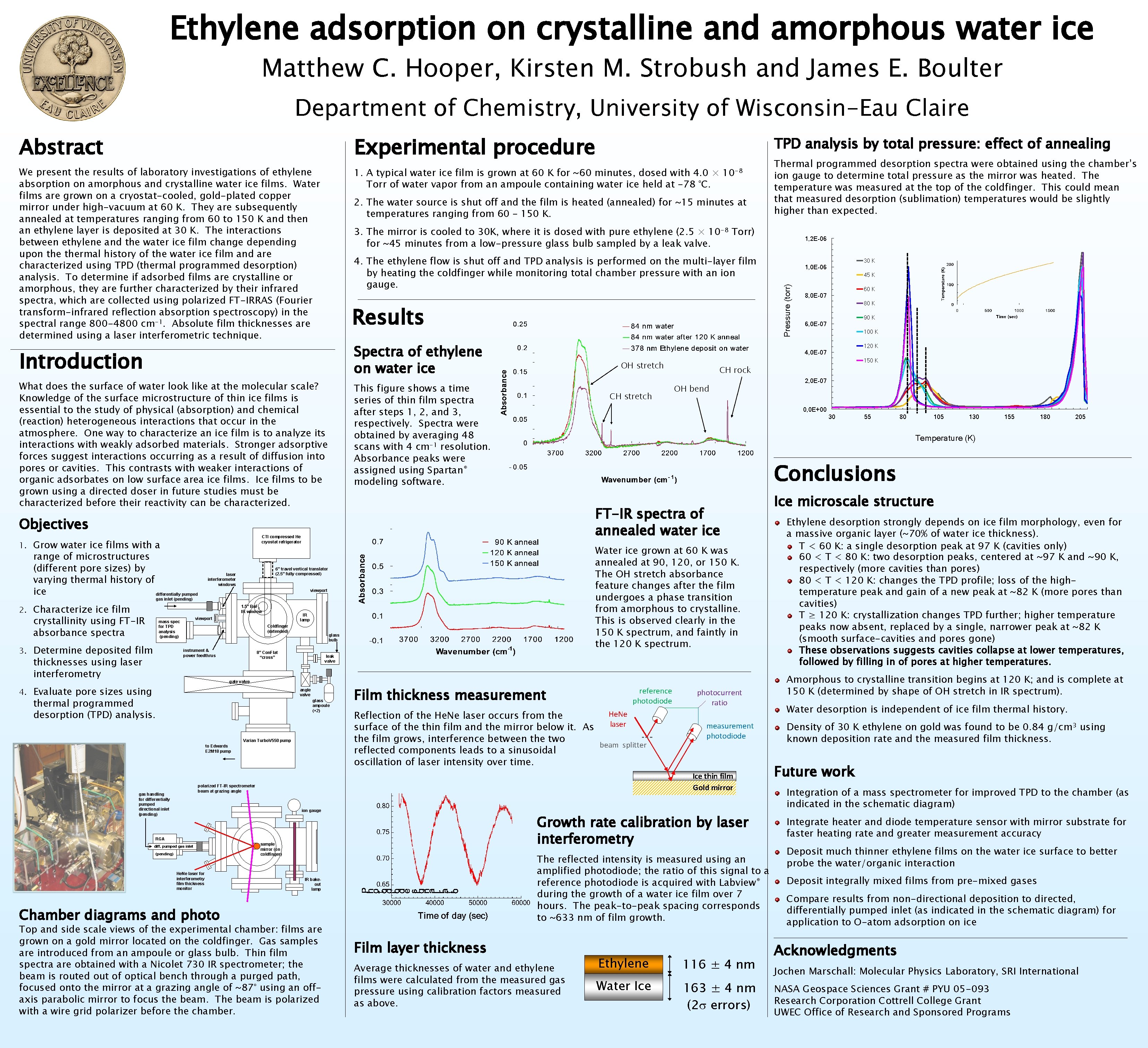
Ethylene adsorption on crystalline and amorphous water ice Matthew C. Hooper, Kirsten M. Strobush and James E. Boulter Department of Chemistry, University of Wisconsin-Eau Claire Abstract Experimental procedure We present the results of laboratory investigations of ethylene absorption on amorphous and crystalline water ice films. Water films are grown on a cryostat-cooled, gold-plated copper mirror under high-vacuum at 60 K. They are subsequently annealed at temperatures ranging from 60 to 150 K and then an ethylene layer is deposited at 30 K. The interactions between ethylene and the water ice film change depending upon thermal history of the water ice film and are characterized using TPD (thermal programmed desorption) analysis. To determine if adsorbed films are crystalline or amorphous, they are further characterized by their infrared spectra, which are collected using polarized FT-IRRAS (Fourier transform-infrared reflection absorption spectroscopy) in the spectral range 800 -4800 cm-1. Absolute film thicknesses are determined using a laser interferometric technique. 1. A typical water ice film is grown at 60 K for ~60 minutes, dosed with 4. 0 × 10 -8 Torr of water vapor from an ampoule containing water ice held at -78 °C. 2. The water source is shut off and the film is heated (annealed) for ~15 minutes at temperatures ranging from 60 – 150 K. Results What does the surface of water look like at the molecular scale? Knowledge of the surface microstructure of thin ice films is essential to the study of physical (absorption) and chemical (reaction) heterogeneous interactions that occur in the atmosphere. One way to characterize an ice film is to analyze its interactions with weakly adsorbed materials. Stronger adsorptive forces suggest interactions occurring as a result of diffusion into pores or cavities. This contrasts with weaker interactions of organic adsorbates on low surface area ice films. Ice films to be grown using a directed doser in future studies must be characterized before their reactivity can be characterized. This figure shows a time series of thin film spectra after steps 1, 2, and 3, respectively. Spectra were obtained by averaging 48 scans with 4 cm-1 resolution. Absorbance peaks were assigned using Spartan® modeling software. CH stretch 3. 4. Characterize ice film crystallinity using FT-IR absorbance spectra mass spec for TPD analysis (pending) Determine deposited film thicknesses using laser interferometry Coldfinger (extended) instrument & power feedthrus OH bend FT-IR spectra of annealed water ice Water ice grown at 60 K was annealed at 90, 120, or 150 K. The OH stretch absorbance feature changes after the film undergoes a phase transition from amorphous to crystalline. This is observed clearly in the 150 K spectrum, and faintly in the 120 K spectrum. leak valve angle valve glass ampoule (× 2) Varian Turbo. V 550 pump to Edwards E 2 M 18 pump Film thickness measurement reference photodiode He. Ne Reflection of the He. Ne laser occurs from the laser surface of the thin film and the mirror below it. As the film grows, interference between the two beam splitter reflected components leads to a sinusoidal oscillation of laser intensity over time. ion gauge (pending) He. Ne laser for interferometry film thickness monitor measurement photodiode Growth rate calibration by laser interferometry RGA diff. pumped gas inlet photocurrent ratio Ice thin film Gold mirror polarized FT-IR spectrometer beam at grazing angle gas handling for differentially pumped directional inlet (pending) sample mirror (on coldfinger) The reflected intensity is measured using an amplified photodiode; the ratio of this signal to a reference photodiode is acquired with Labview® during the growth of a water ice film over 7 hours. The peak-to-peak spacing corresponds to ~633 nm of film growth. IR bakeout lamp Chamber diagrams and photo Top and side scale views of the experimental chamber: films are grown on a gold mirror located on the coldfinger. Gas samples are introduced from an ampoule or glass bulb. Thin film spectra are obtained with a Nicolet 730 IR spectrometer; the beam is routed out of optical bench through a purged path, focused onto the mirror at a grazing angle of ~87° using an offaxis parabolic mirror to focus the beam. The beam is polarized with a wire grid polarizer before the chamber. 90 K 6, 0 E-07 100 K 120 K CH rock gate valve Evaluate pore sizes using thermal programmed desorption (TPD) analysis. 80 K 2, 0 E-07 55 80 105 130 155 180 205 Conclusions glass bulb 8” Con. Flat “cross” 8, 0 E-07 Temperature (K) IR lamp viewport 60 K 30 viewport 1. 5” Ba. F 2 IR window 45 K 0, 0 E+00 gas inlet (pending) 2. 1, 0 E-06 150 K OH stretch 6” travel vertical translator (2. 5” fully compressed) laser interferometer windows 30 K 4, 0 E-07 CTI compressed He cryostat refrigerator Grow water ice films with a range of microstructures (different pore sizes) by varying thermal history of ice differentially pumped 1, 2 E-06 Pressure (torr) 4. The ethylene flow is shut off and TPD analysis is performed on the multi-layer film by heating the coldfinger while monitoring total chamber pressure with an ion gauge. Introduction 1. Thermal programmed desorption spectra were obtained using the chamber’s ion gauge to determine total pressure as the mirror was heated. The temperature was measured at the top of the coldfinger. This could mean that measured desorption (sublimation) temperatures would be slightly higher than expected. 3. The mirror is cooled to 30 K, where it is dosed with pure ethylene (2. 5 × 10 -8 Torr) for ~45 minutes from a low-pressure glass bulb sampled by a leak valve. Spectra of ethylene on water ice Objectives TPD analysis by total pressure: effect of annealing Film layer thickness Average thicknesses of water and ethylene films were calculated from the measured gas pressure using calibration factors measured as above. Ethylene 116 ± 4 nm Water Ice 163 ± 4 nm (2 s errors) Ice microscale structure Ethylene desorption strongly depends on ice film morphology, even for a massive organic layer (~70% of water ice thickness). T < 60 K: a single desorption peak at 97 K (cavities only) 60 < T < 80 K: two desorption peaks, centered at ~97 K and ~90 K, respectively (more cavities than pores) 80 < T < 120 K: changes the TPD profile; loss of the hightemperature peak and gain of a new peak at ~82 K (more pores than cavities) T ≥ 120 K: crystallization changes TPD further; higher temperature peaks now absent, replaced by a single, narrower peak at ~82 K (smooth surface-cavities and pores gone) These observations suggests cavities collapse at lower temperatures, followed by filling in of pores at higher temperatures. Amorphous to crystalline transition begins at 120 K; and is complete at 150 K (determined by shape of OH stretch in IR spectrum). Water desorption is independent of ice film thermal history. Density of 30 K ethylene on gold was found to be 0. 84 g/cm 3 using known deposition rate and the measured film thickness. Future work Integration of a mass spectrometer for improved TPD to the chamber (as indicated in the schematic diagram) Integrate heater and diode temperature sensor with mirror substrate for faster heating rate and greater measurement accuracy Deposit much thinner ethylene films on the water ice surface to better probe the water/organic interaction Deposit integrally mixed films from pre-mixed gases Compare results from non-directional deposition to directed, differentially pumped inlet (as indicated in the schematic diagram) for application to O-atom adsorption on ice Acknowledgments Jochen Marschall: Molecular Physics Laboratory, SRI International NASA Geospace Sciences Grant # PYU 05 -093 Research Corporation Cottrell College Grant UWEC Office of Research and Sponsored Programs