DISTORTION PRODUCT OTOACOUSTIC EMISSIONS PROVIDE CLUES TO HEARING
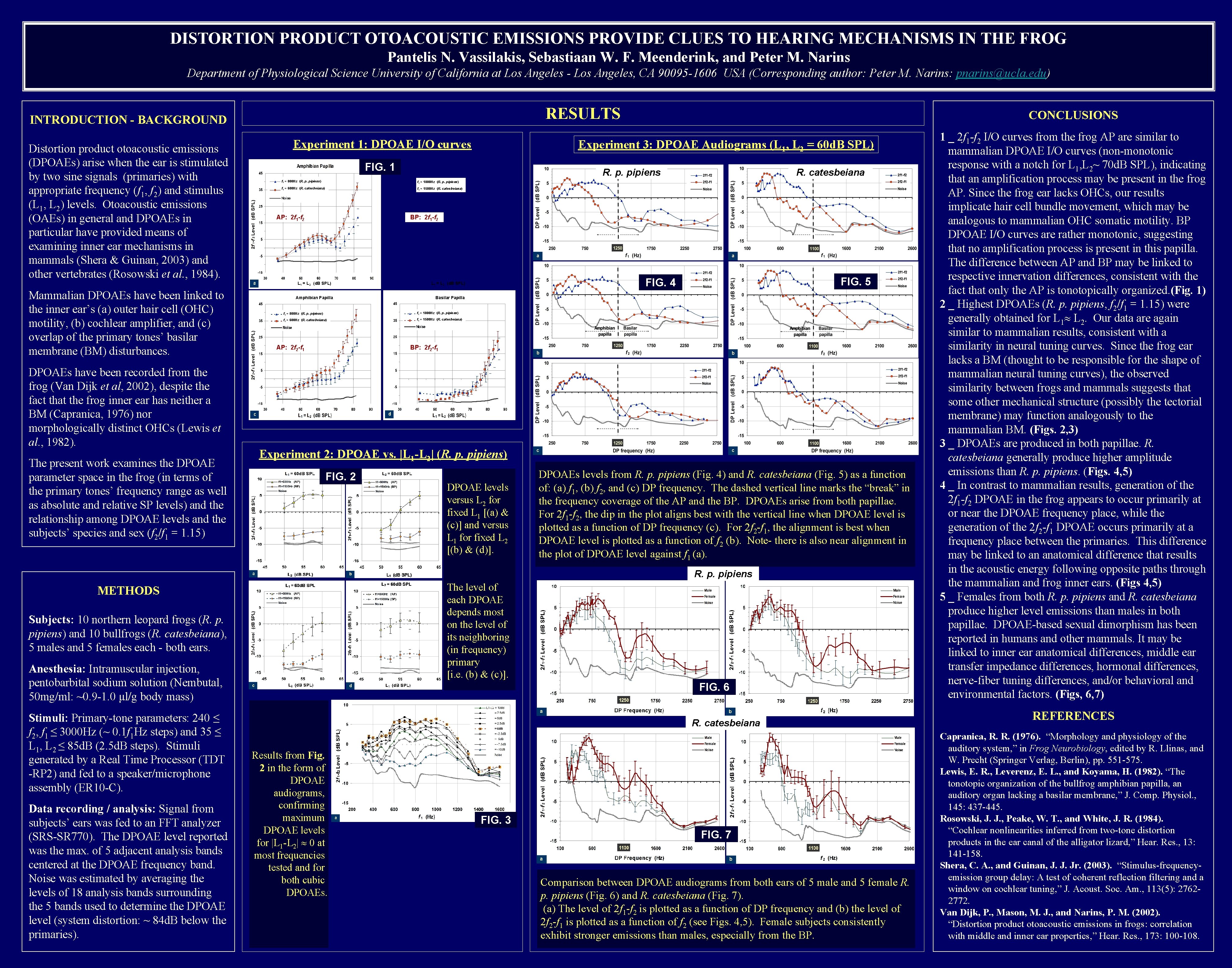
- Slides: 1
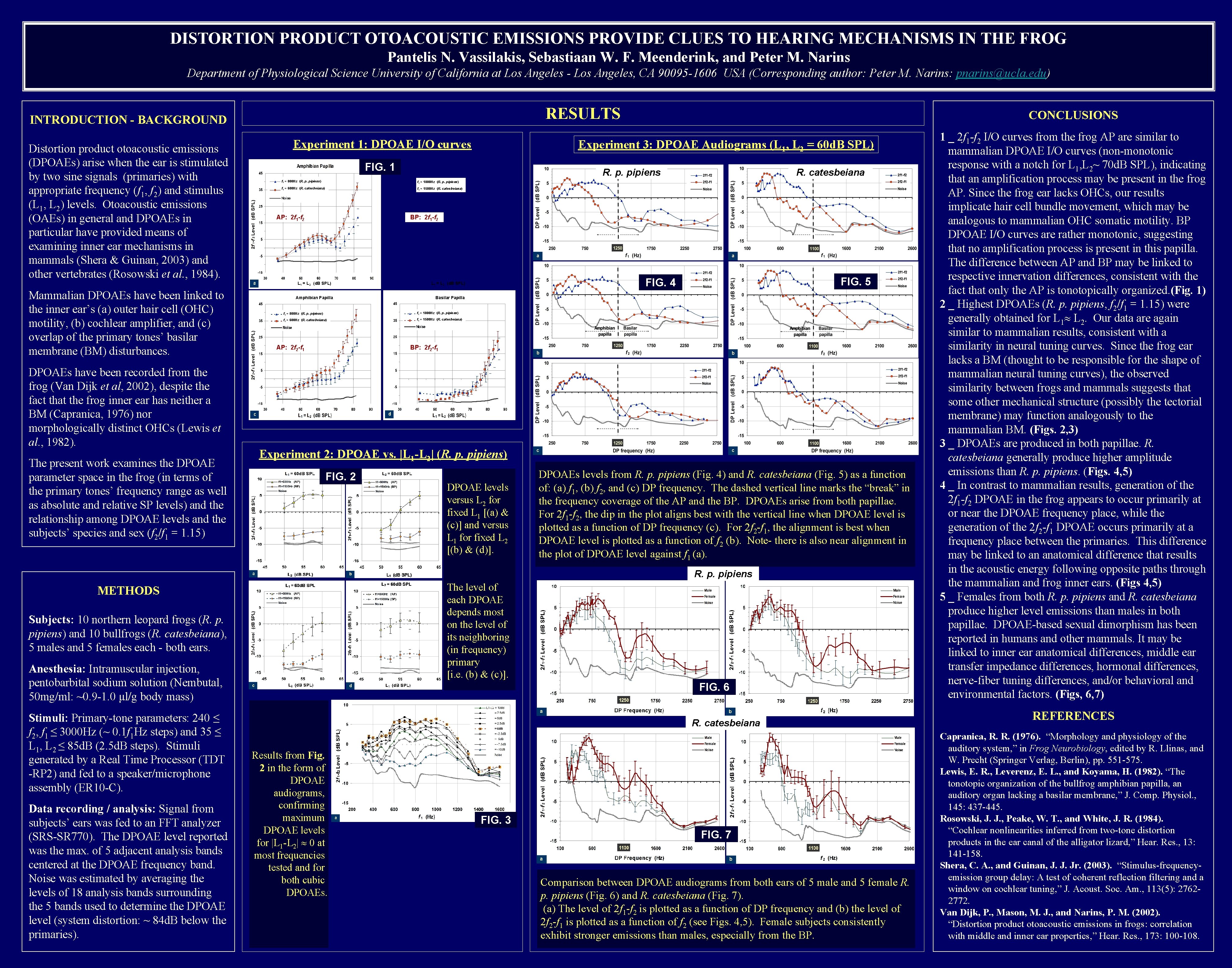
DISTORTION PRODUCT OTOACOUSTIC EMISSIONS PROVIDE CLUES TO HEARING MECHANISMS IN THE FROG Pantelis N. Vassilakis, Sebastiaan W. F. Meenderink, and Peter M. Narins Department of Physiological Science University of California at Los Angeles - Los Angeles, CA 90095 -1606 USA (Corresponding author: Peter M. Narins: pnarins@ucla. edu) RESULTS INTRODUCTION - BACKGROUND Distortion product otoacoustic emissions (DPOAEs) arise when the ear is stimulated by two sine signals (primaries) with appropriate frequency (f 1, f 2) and stimulus (L 1, L 2) levels. Otoacoustic emissions (OAEs) in general and DPOAEs in particular have provided means of examining inner ear mechanisms in mammals (Shera & Guinan, 2003) and other vertebrates (Rosowski et al. , 1984). Experiment 1: DPOAE I/O curves DPOAEs have been recorded from the frog (Van Dijk et al, 2002), despite the fact that the frog inner ear has neither a BM (Capranica, 1976) nor morphologically distinct OHCs (Lewis et al. , 1982). The present work examines the DPOAE parameter space in the frog (in terms of the primary tones’ frequency range as well as absolute and relative SP levels) and the relationship among DPOAE levels and the subjects’ species and sex (f 2/f 1 = 1. 15) R. p. pipiens f 1 = 800 Hz (R. p. pipiens) f 1 = 1800 Hz (R. p. pipiens) f 1 = 600 Hz (R. catesbeiana) f 1 = 1500 Hz (R. catesbeiana) R. catesbeiana BP: 2 f 1 -f 2 L 1 = L 2 (d. B SPL) Mammalian DPOAEs have been linked to the inner ear’s (a) outer hair cell (OHC) motility, (b) cochlear amplifier, and (c) overlap of the primary tones’ basilar membrane (BM) disturbances. Experiment 3: DPOAE Audiograms (L 1, L 2 = 60 d. B SPL) FIG. 1 AP: 2 f 1 -f 2 CONCLUSIONS Amphibian Papilla Basilar Papilla f 1 = 800 Hz (R. p. pipiens) f 1 = 1800 Hz (R. p. pipiens) f 1 = 600 Hz (R. catesbeiana) f 1 = 1500 Hz (R. catesbeiana) AP: 2 f 2 -f 1 FIG. 5 FIG. 4 L 1 = L 2 (d. B SPL) BP: 2 f 2 -f 1 Experiment 2: DPOAE vs. |L 1 -L 2| (R. p. pipiens) FIG. 2 DPOAE levels versus L 2 for fixed L 1 [(a) & (c)] and versus L 1 for fixed L 2 [(b) & (d)]. DPOAEs levels from R. p. pipiens (Fig. 4) and R. catesbeiana (Fig. 5) as a function of: (a) f 1, (b) f 2, and (c) DP frequency. The dashed vertical line marks the “break” in the frequency coverage of the AP and the BP. DPOAEs arise from both papillae. For 2 f 1 -f 2, the dip in the plot aligns best with the vertical line when DPOAE level is plotted as a function of DP frequency (c). For 2 f 2 -f 1, the alignment is best when DPOAE level is plotted as a function of f 2 (b). Note- there is also near alignment in the plot of DPOAE level against f 1 (a). R. p. pipiens The level of each DPOAE depends most on the level of its neighboring (in frequency) primary [i. e. (b) & (c)]. METHODS Subjects: 10 northern leopard frogs (R. p. pipiens) and 10 bullfrogs (R. catesbeiana), 5 males and 5 females each - both ears. Anesthesia: Intramuscular injection, pentobarbital sodium solution (Nembutal, 50 mg/ml: ~0. 9 -1. 0 μl/g body mass) Stimuli: Primary-tone parameters: 240 ≤ f 2, f 1 ≤ 3000 Hz (~ 0. 1 f 1 Hz steps) and 35 ≤ L 1, L 2 ≤ 85 d. B (2. 5 d. B steps). Stimuli generated by a Real Time Processor (TDT -RP 2) and fed to a speaker/microphone assembly (ER 10 -C). Data recording / analysis: Signal from subjects’ ears was fed to an FFT analyzer (SRS-SR 770). The DPOAE level reported was the max. of 5 adjacent analysis bands centered at the DPOAE frequency band. Noise was estimated by averaging the levels of 18 analysis bands surrounding the 5 bands used to determine the DPOAE level (system distortion: ~ 84 d. B below the primaries). FIG. 6 R. catesbeiana Results from Fig. 2 in the form of DPOAE audiograms, confirming maximum DPOAE levels for |L 1 -L 2| 0 at most frequencies tested and for both cubic DPOAEs. FIG. 3 FIG. 7 Comparison between DPOAE audiograms from both ears of 5 male and 5 female R. p. pipiens (Fig. 6) and R. catesbeiana (Fig. 7). (a) The level of 2 f 1 -f 2 is plotted as a function of DP frequency and (b) the level of 2 f 2 -f 1 is plotted as a function of f 2 (see Figs. 4, 5). Female subjects consistently exhibit stronger emissions than males, especially from the BP. 1 _ 2 f 1 -f 2 I/O curves from the frog AP are similar to mammalian DPOAE I/O curves (non-monotonic response with a notch for L 1, L 2~ 70 d. B SPL), indicating that an amplification process may be present in the frog AP. Since the frog ear lacks OHCs, our results implicate hair cell bundle movement, which may be analogous to mammalian OHC somatic motility. BP DPOAE I/O curves are rather monotonic, suggesting that no amplification process is present in this papilla. The difference between AP and BP may be linked to respective innervation differences, consistent with the fact that only the AP is tonotopically organized. (Fig. 1) 2 _ Highest DPOAEs (R. p. pipiens, f 2/f 1 = 1. 15) were generally obtained for L 1 L 2. Our data are again similar to mammalian results, consistent with a similarity in neural tuning curves. Since the frog ear lacks a BM (thought to be responsible for the shape of mammalian neural tuning curves), the observed similarity between frogs and mammals suggests that some other mechanical structure (possibly the tectorial membrane) may function analogously to the mammalian BM. (Figs. 2, 3) 3 _ DPOAEs are produced in both papillae. R. catesbeiana generally produce higher amplitude emissions than R. p. pipiens. (Figs. 4, 5) 4 _ In contrast to mammalian results, generation of the 2 f 1 -f 2 DPOAE in the frog appears to occur primarily at or near the DPOAE frequency place, while the generation of the 2 f 2 -f 1 DPOAE occurs primarily at a frequency place between the primaries. This difference may be linked to an anatomical difference that results in the acoustic energy following opposite paths through the mammalian and frog inner ears. (Figs 4, 5) 5 _ Females from both R. p. pipiens and R. catesbeiana produce higher level emissions than males in both papillae. DPOAE-based sexual dimorphism has been reported in humans and other mammals. It may be linked to inner ear anatomical differences, middle ear transfer impedance differences, hormonal differences, nerve-fiber tuning differences, and/or behavioral and environmental factors. (Figs, 6, 7) REFERENCES Capranica, R. R. (1976). “Morphology and physiology of the auditory system, ” in Frog Neurobiology, edited by R. Llinas, and W. Precht (Springer Verlag, Berlin), pp. 551 -575. Lewis, E. R. , Leverenz, E. L. , and Koyama, H. (1982). “The tonotopic organization of the bullfrog amphibian papilla, an auditory organ lacking a basilar membrane, ” J. Comp. Physiol. , 145: 437 -445. Rosowski, J. J. , Peake, W. T. , and White, J. R. (1984). “Cochlear nonlinearities inferred from two-tone distortion products in the ear canal of the alligator lizard, ” Hear. Res. , 13: 141 -158. Shera, C. A. , and Guinan, J. J. Jr. (2003). “Stimulus-frequencyemission group delay: A test of coherent reflection filtering and a window on cochlear tuning, ” J. Acoust. Soc. Am. , 113(5): 27622772. Van Dijk, P. , Mason, M. J. , and Narins, P. M. (2002). “Distortion product otoacoustic emissions in frogs: correlation with middle and inner ear properties, ” Hear. Res. , 173: 100 -108.