Design Considerations for a HighEfficiency HighGain FreeElectron Laser
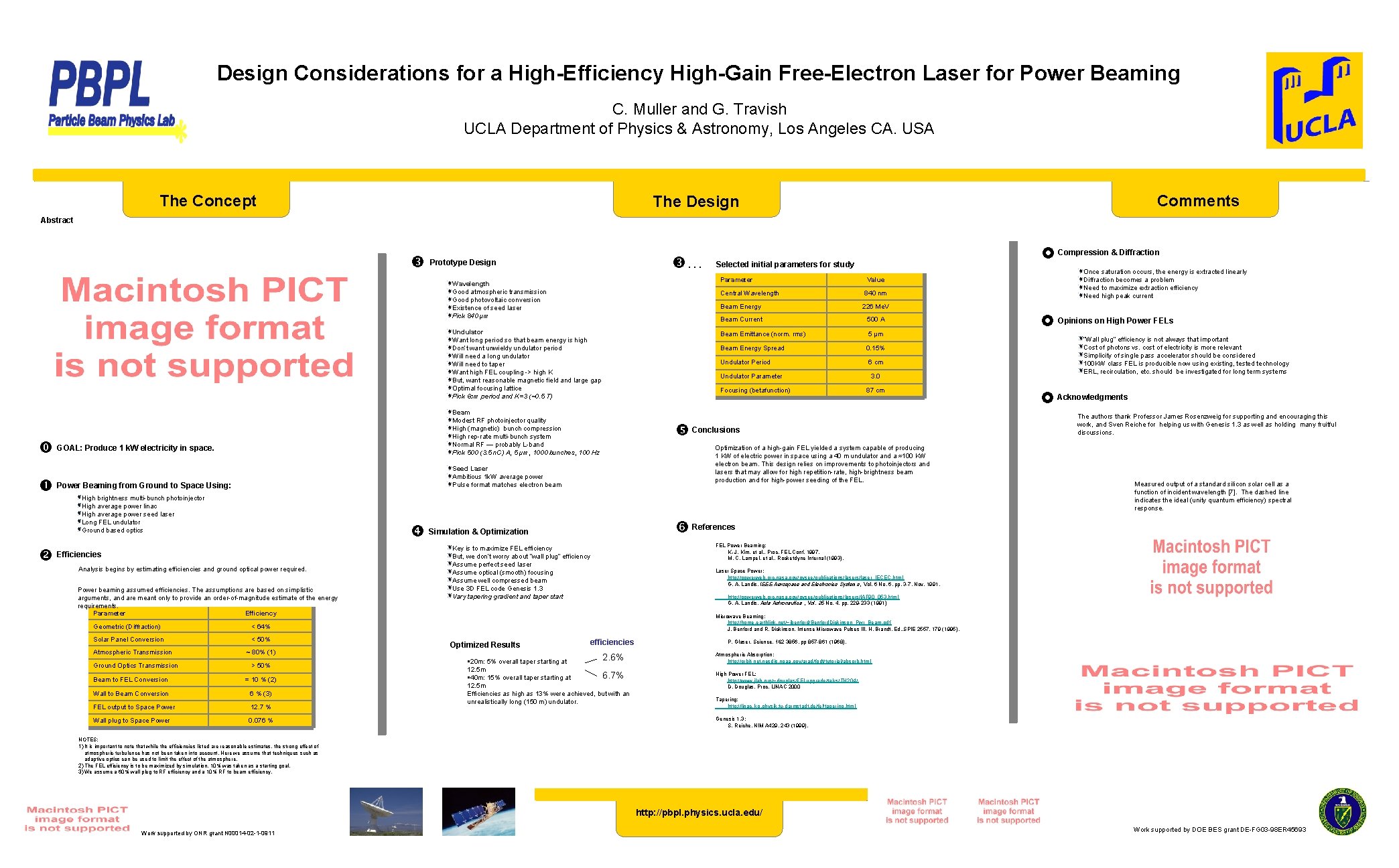
- Slides: 1
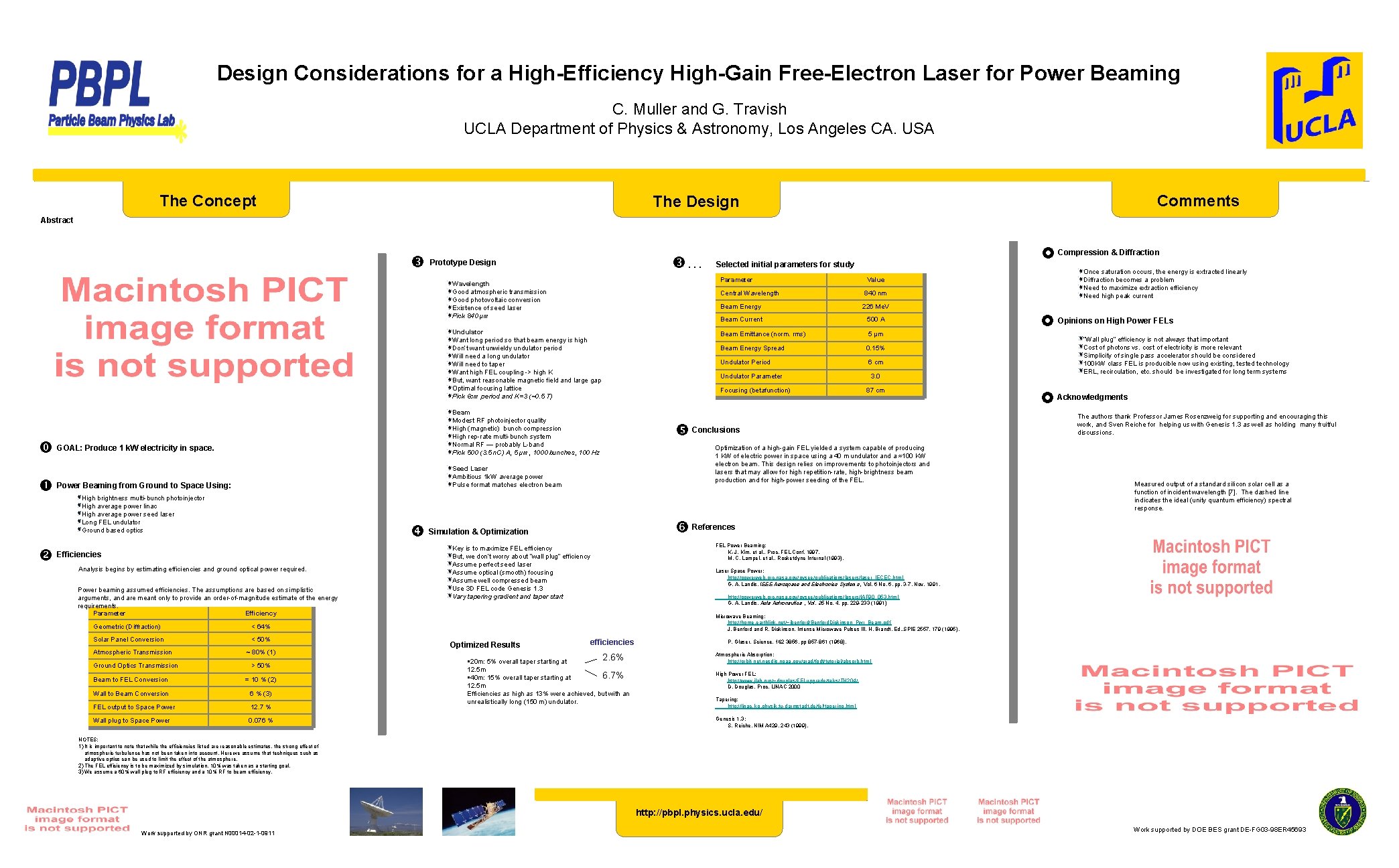
Design Considerations for a High-Efficiency High-Gain Free-Electron Laser for Power Beaming C. Muller and G. Travish UCLA Department of Physics & Astronomy, Los Angeles CA. USA The Concept Comments The Design Abstract … Prototype Design Central Wavelength Undulator Want long period so that beam energy is high Don’t want unwieldy undulator period Will need a long undulator Will need to taper Want high FEL coupling -> high K But, want reasonable magnetic field and large gap Optimal focusing lattice Pick 6 cm period and K=3 (~0. 5 T) Beam Modest RF photoinjector quality High (magnetic) bunch compression High rep-rate multi-bunch system Normal RF — probably L-band Pick 500 (3. 5 n. C) A, 5 µm, 1000 bunches, 100 Hz GOAL: Produce 1 k. W electricity in space. Seed Laser Ambitious 1 k. W average power Pulse format matches electron beam Power Beaming from Ground to Space Using: High brightness multi-bunch photoinjector High average power linac High average power seed laser Long FEL undulator Ground based optics Analysis begins by estimating efficiencies and ground optical power required. Power beaming assumed efficiencies. The assumptions are based on simplistic arguments, and are meant only to provide an order-of-magnitude estimate of the energy requirements. Parameter Efficiency Geometric (Diffraction) < 64% Solar Panel Conversion < 50% Atmospheric Transmission Ground Optics Transmission ~ 80% (1) > 50% Beam to FEL Conversion = 10 % (2) Wall to Beam Conversion 6 % (3) FEL output to Space Power 12. 7 % Wall plug to Space Power 0. 076 % Value 840 nm Beam Energy 226 Me. V Beam Current 500 A Beam Emittance (norm. rms) 5 µm Beam Energy Spread 0. 15% Undulator Period 6 cm Undulator Parameter Focusing (betafunction) 3. 0 87 cm Conclusions Optimization of a high-gain FEL yielded a system capable of producing 1 KW of electric power in space using a 40 m undulator and a ≈100 KW electron beam. This design relies on improvements to photoinjectors and lasers that may allow for high repetition-rate, high-brightness beam production and for high-power seeding of the FEL. Once saturation occurs, the energy is extracted linearly Diffraction becomes a problem Need to maximize extraction efficiency Need high peak current Opinions on High Power FELs “Wall plug” efficiency is not always that important Cost of photons vs. cost of electricity is more relevant Simplicity of single pass accelerator should be considered 100 KW class FEL is producible now using existing, tested technology ERL, recirculation, etc. should be investigated for long term systems Acknowledgments The authors thank Professor James Rosenzweig for supporting and encouraging this work, and Sven Reiche for helping us with Genesis 1. 3 as well as holding many fruitful discussions. Measured output of a standard silicon solar cell as a function of incident wavelength [7]. The dashed line indicates the ideal (unity quantum efficiency) spectral response. References Simulation & Optimization Efficiencies Selected initial parameters for study Parameter Wavelength Good atmospheric transmission Good photovoltaic conversion Existence of seed laser Pick 840 µm Compression & Diffraction FEL Power Beaming: K. -J. Kim, et al. , Proc. FEL Conf. 1997. M. C. Lampel, et al. , Rocketdyne Internal (1993). Key is to maximize FEL efficiency But, we don’t worry about “wall plug” efficiency Assume perfect seed laser Assume optical (smooth) focusing Assume well compressed beam Use 3 D FEL code Genesis 1. 3 Vary tapering gradient and taper start Laser Space Power: http: //powerweb. grc. nasa. gov/pvsee/publications/laser_IECEC. html G. A. Landis, IEEE Aerospace and Electronics Systems, Vol. 6 No. 6, pp. 3 -7, Nov. 1991. http: //powerweb. grc. nasa. gov/pvsee/publications/lasers/IAF 90_053. html G. A. Landis, Acta Astronautica , Vol. 25 No. 4, pp. 229 -233 (1991) Microwave Beaming: http: //home. earthlink. net/~jbenford/Benford. Dickinson_Pwr_Beam. pdf J. Benford and R. Dickinson, Intense Microwave Pulses III, H. Brandt, Ed. , SPIE 2557, 179 (1995). Optimized Results efficiencies 2. 6% § 20 m: 5% overall taper starting at 12. 5 m 6. 7% § 40 m: 15% overall taper starting at 12. 5 m Efficiencies as high as 13% were achieved, but with an unrealistically long (150 m) undulator. P. Glaser, Science, 162 3856, pp 857 -861 (1968). Atmospheric Absorption: http: //orbit-net. nesdis. noaa. gov/arad/fpdt/tutorial/absorb. html High Power FEL: http: //www. jlab. org/~douglas/FELupgrade/talks/TH 204/ D. Douglas, Proc. LINAC 2000 Tapering: http: //linac. ikp. physik. tu-darmstadt. de/fel/tapering. html Genesis 1. 3: S. Reiche, NIM A 429, 243 (1999). NOTES: 1) It is important to note that while the efficiencies listed are reasonable estimates, the strong effect of atmospheric turbulence has not been taken into account. Here we assume that techniques such as adaptive optics can be used to limit the effect of the atmosphere. 2) The FEL efficiency is to be maximized by simulation. 10% was taken as a starting goal. 3) We assume a 60% wall plug to RF efficiency and a 10% RF to beam efficiency. http: //pbpl. physics. ucla. edu/ Work supported by ONR grant N 00014 -02 -1 -0911 Work supported by DOE BES grant DE-FG 03 -98 ER 45693