Delineating floodplains of rivers in Maryland using HECRAS
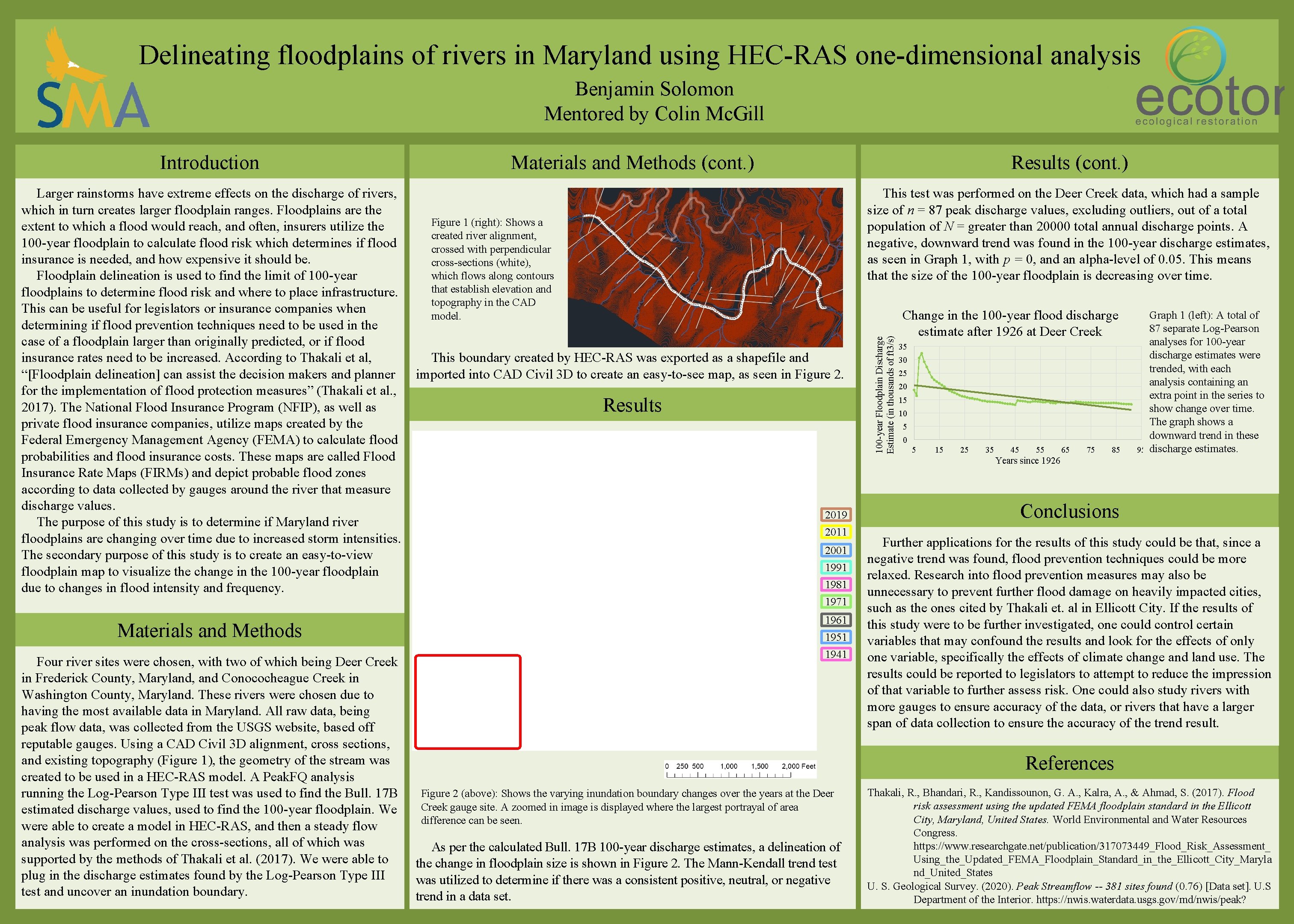
- Slides: 1
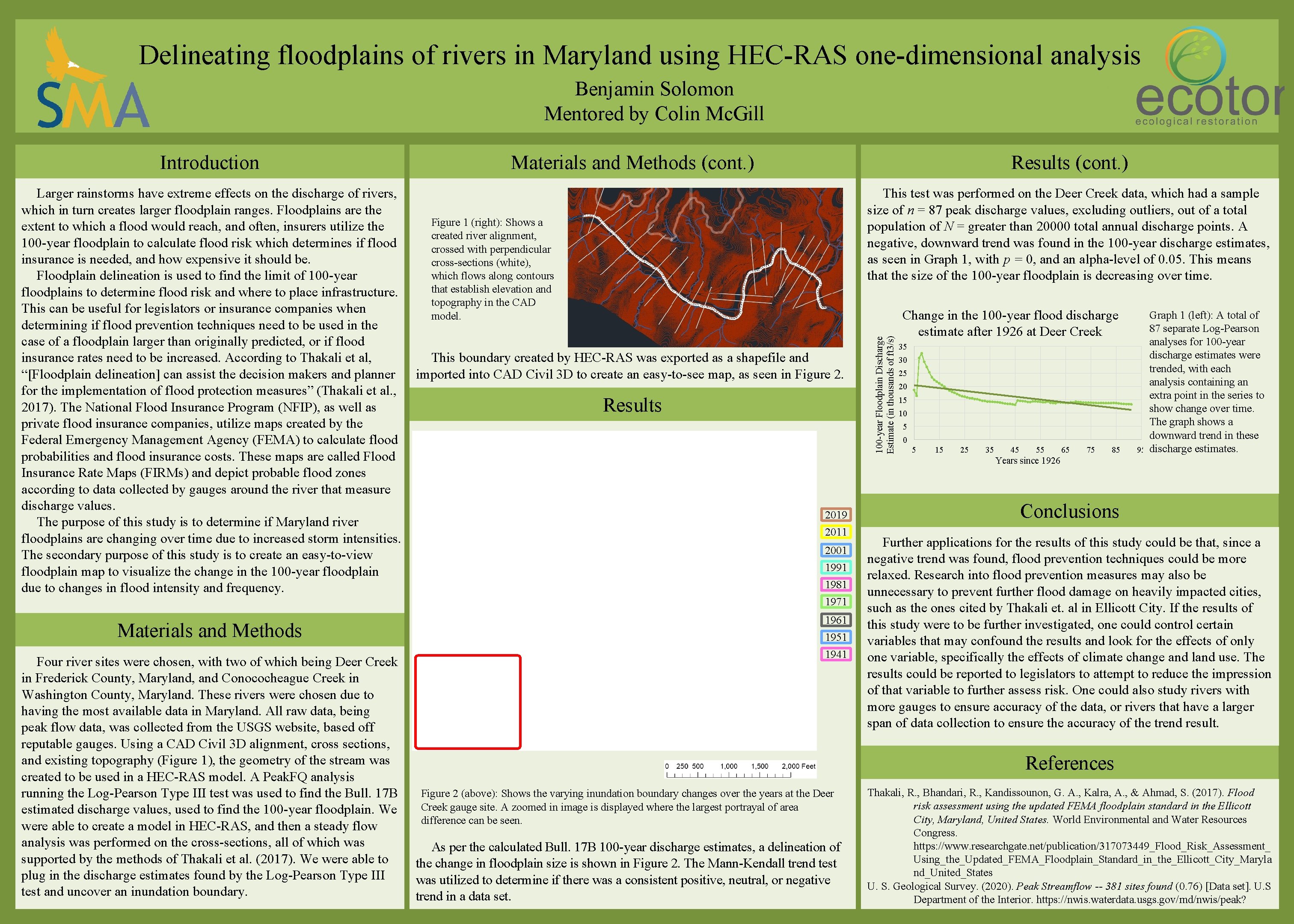
Delineating floodplains of rivers in Maryland using HEC-RAS one-dimensional analysis Benjamin Solomon Mentored by Colin Mc. Gill Larger rainstorms have extreme effects on the discharge of rivers, which in turn creates larger floodplain ranges. Floodplains are the extent to which a flood would reach, and often, insurers utilize the 100 -year floodplain to calculate flood risk which determines if flood insurance is needed, and how expensive it should be. Floodplain delineation is used to find the limit of 100 -year floodplains to determine flood risk and where to place infrastructure. This can be useful for legislators or insurance companies when determining if flood prevention techniques need to be used in the case of a floodplain larger than originally predicted, or if flood insurance rates need to be increased. According to Thakali et al, “[Floodplain delineation] can assist the decision makers and planner for the implementation of flood protection measures” (Thakali et al. , 2017). The National Flood Insurance Program (NFIP), as well as private flood insurance companies, utilize maps created by the Federal Emergency Management Agency (FEMA) to calculate flood probabilities and flood insurance costs. These maps are called Flood Insurance Rate Maps (FIRMs) and depict probable flood zones according to data collected by gauges around the river that measure discharge values. The purpose of this study is to determine if Maryland river floodplains are changing over time due to increased storm intensities. The secondary purpose of this study is to create an easy-to-view floodplain map to visualize the change in the 100 -year floodplain due to changes in flood intensity and frequency. Materials and Methods (cont. ) Results (cont. ) This test was performed on the Deer Creek data, which had a sample size of n = 87 peak discharge values, excluding outliers, out of a total population of N = greater than 20000 total annual discharge points. A negative, downward trend was found in the 100 -year discharge estimates, as seen in Graph 1, with p = 0, and an alpha-level of 0. 05. This means that the size of the 100 -year floodplain is decreasing over time. Figure 1 (right): Shows a created river alignment, crossed with perpendicular cross-sections (white), which flows along contours that establish elevation and topography in the CAD model. This boundary created by HEC-RAS was exported as a shapefile and imported into CAD Civil 3 D to create an easy-to-see map, as seen in Figure 2. Results 100 -year Floodplain Discharge Estimate (in thousands of ft 3/s) Introduction Change in the 100 -year flood discharge estimate after 1926 at Deer Creek 35 30 25 20 15 10 5 15 25 35 45 55 65 75 85 95 Graph 1 (left): A total of 87 separate Log-Pearson analyses for 100 -year discharge estimates were trended, with each analysis containing an extra point in the series to show change over time. The graph shows a downward trend in these discharge estimates. Years since 1926 2019 2011 2001 1991 1981 1971 Materials and Methods 1961 Four river sites were chosen, with two of which being Deer Creek in Frederick County, Maryland, and Conococheague Creek in Washington County, Maryland. These rivers were chosen due to having the most available data in Maryland. All raw data, being peak flow data, was collected from the USGS website, based off reputable gauges. Using a CAD Civil 3 D alignment, cross sections, and existing topography (Figure 1), the geometry of the stream was created to be used in a HEC-RAS model. A Peak. FQ analysis running the Log-Pearson Type III test was used to find the Bull. 17 B estimated discharge values, used to find the 100 -year floodplain. We were able to create a model in HEC-RAS, and then a steady flow analysis was performed on the cross-sections, all of which was supported by the methods of Thakali et al. (2017). We were able to plug in the discharge estimates found by the Log-Pearson Type III test and uncover an inundation boundary. 1941 1951 Conclusions Further applications for the results of this study could be that, since a negative trend was found, flood prevention techniques could be more relaxed. Research into flood prevention measures may also be unnecessary to prevent further flood damage on heavily impacted cities, such as the ones cited by Thakali et. al in Ellicott City. If the results of this study were to be further investigated, one could control certain variables that may confound the results and look for the effects of only one variable, specifically the effects of climate change and land use. The results could be reported to legislators to attempt to reduce the impression of that variable to further assess risk. One could also study rivers with more gauges to ensure accuracy of the data, or rivers that have a larger span of data collection to ensure the accuracy of the trend result. References Figure 2 (above): Shows the varying inundation boundary changes over the years at the Deer Creek gauge site. A zoomed in image is displayed where the largest portrayal of area difference can be seen. As per the calculated Bull. 17 B 100 -year discharge estimates, a delineation of the change in floodplain size is shown in Figure 2. The Mann-Kendall trend test was utilized to determine if there was a consistent positive, neutral, or negative trend in a data set. Thakali, R. , Bhandari, R. , Kandissounon, G. A. , Kalra, A. , & Ahmad, S. (2017). Flood risk assessment using the updated FEMA floodplain standard in the Ellicott City, Maryland, United States. World Environmental and Water Resources Congress. https: //www. researchgate. net/publication/317073449_Flood_Risk_Assessment_ Using_the_Updated_FEMA_Floodplain_Standard_in_the_Ellicott_City_Maryla nd_United_States U. S. Geological Survey. (2020). Peak Streamflow -- 381 sites found (0. 76) [Data set]. U. S Department of the Interior. https: //nwis. waterdata. usgs. gov/md/nwis/peak?