Chemistry Unit 2 Section 1 Isomerism Structural isomers
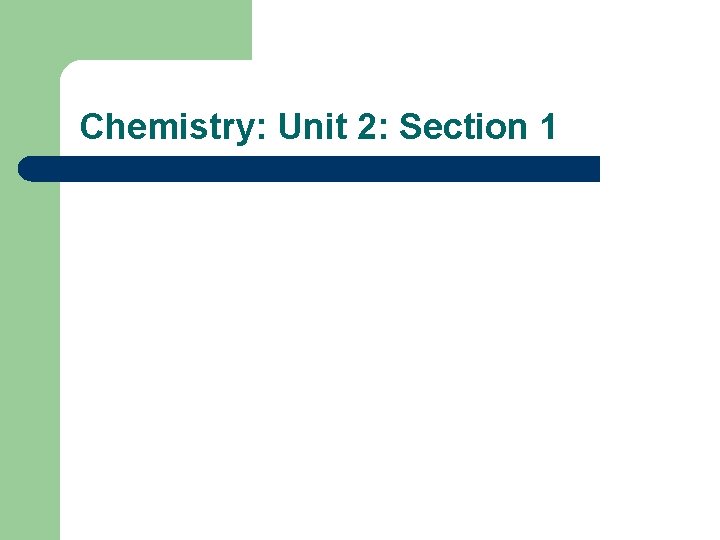
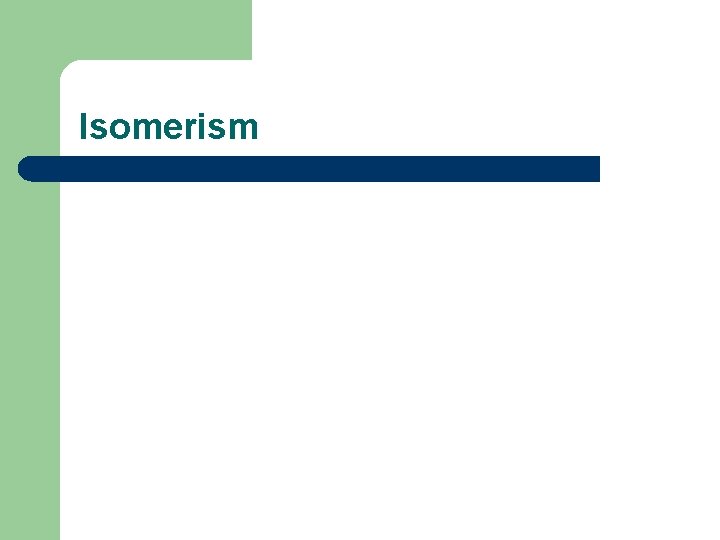
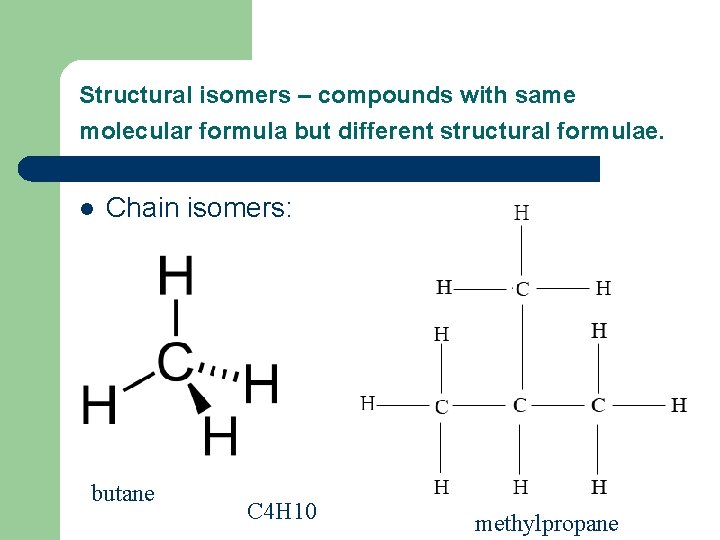
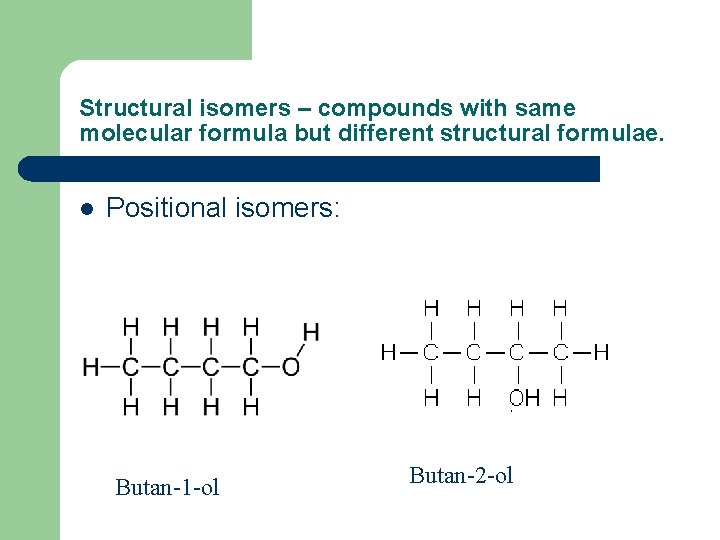
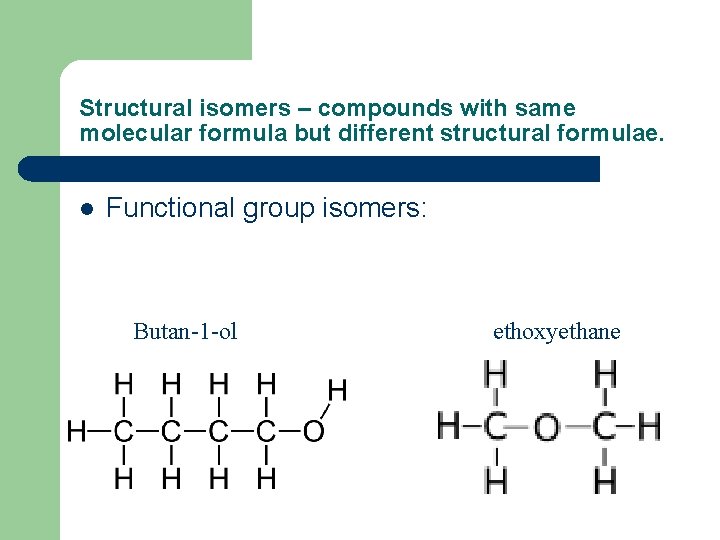
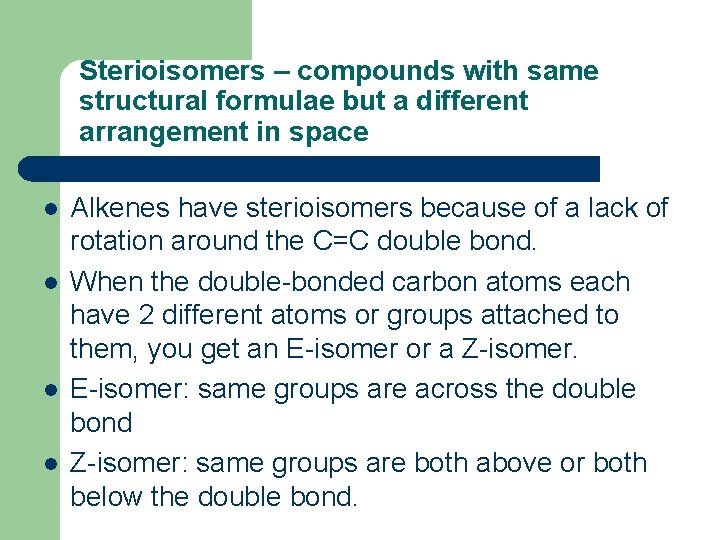
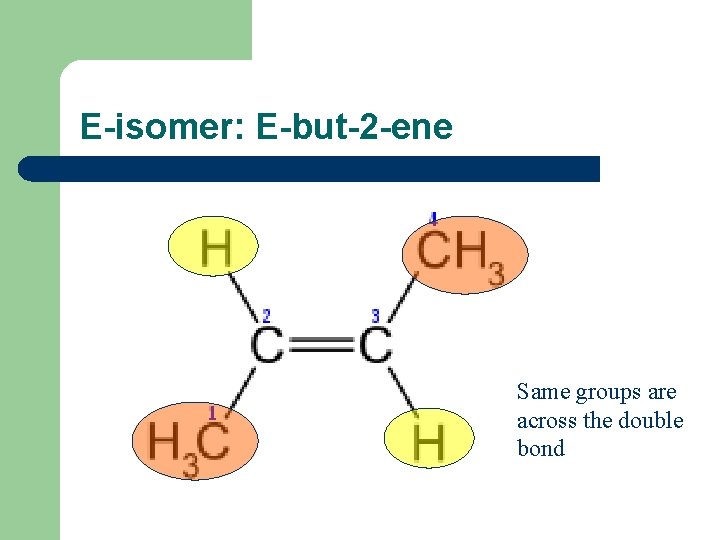
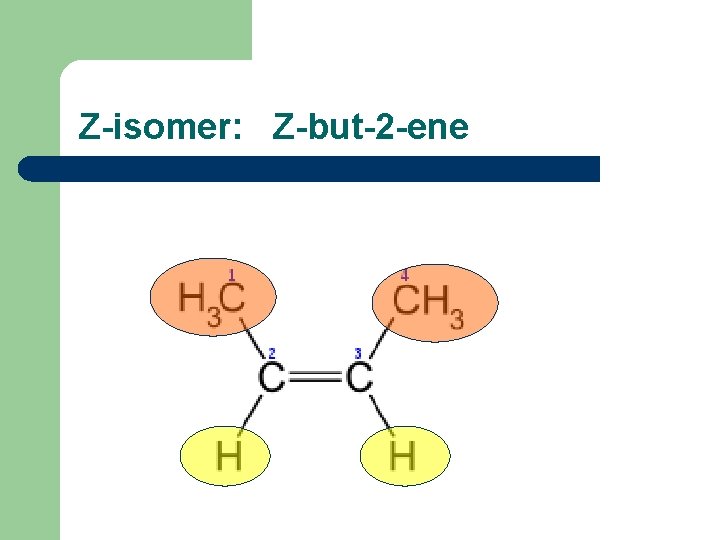
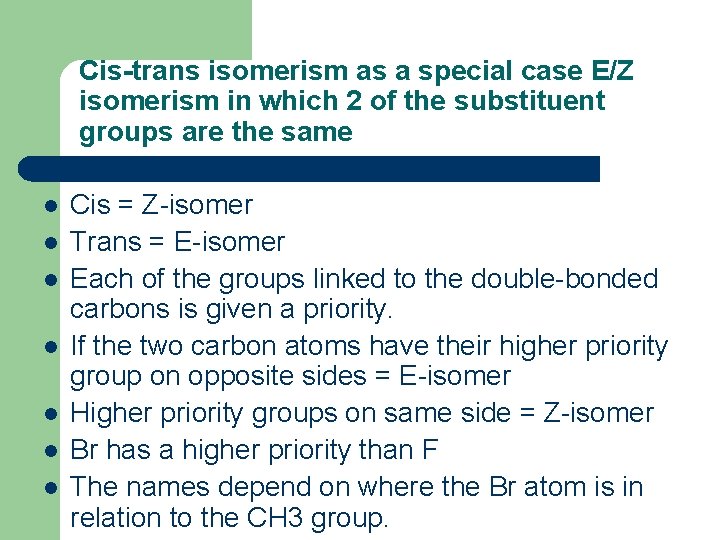
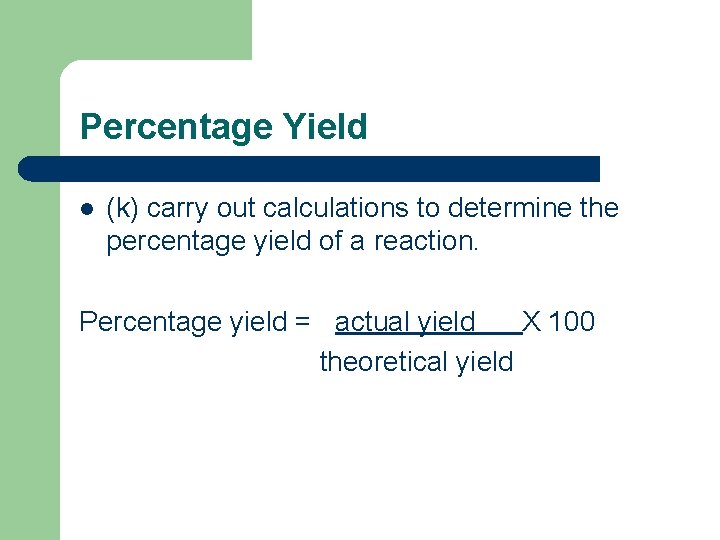
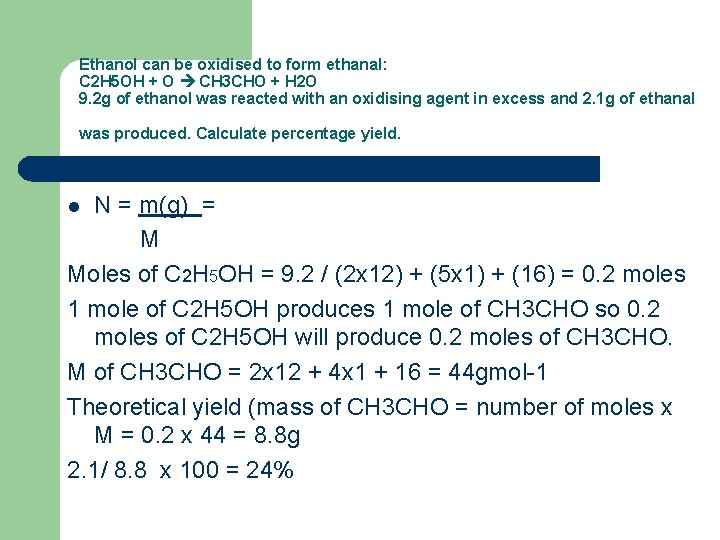
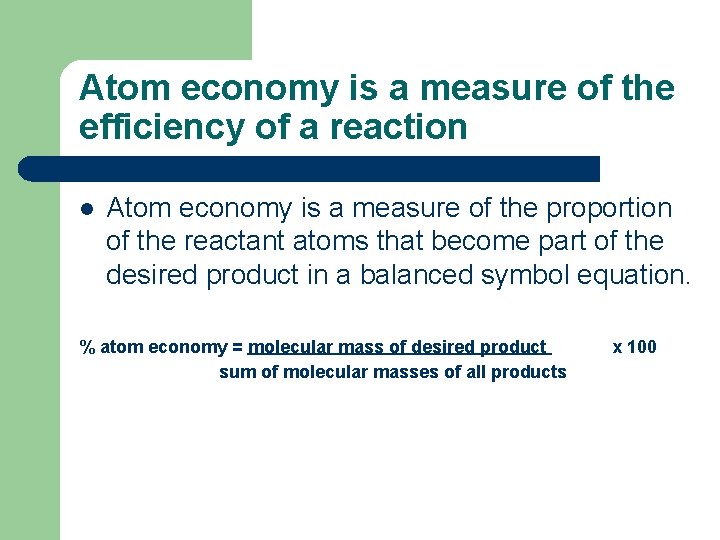
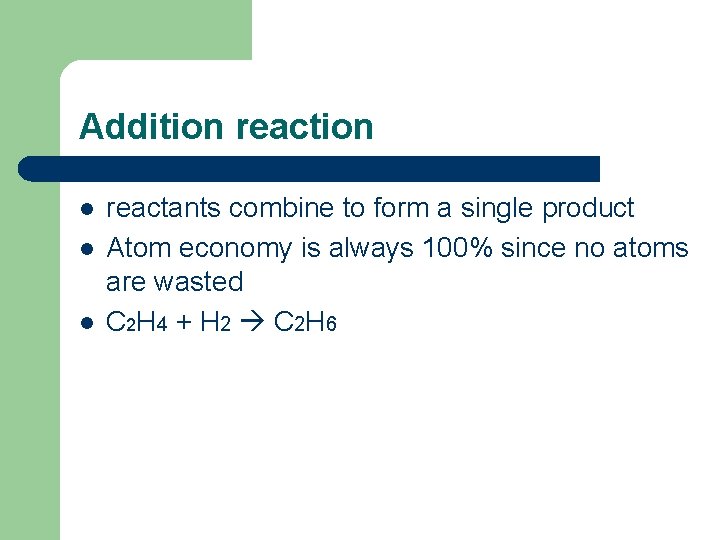
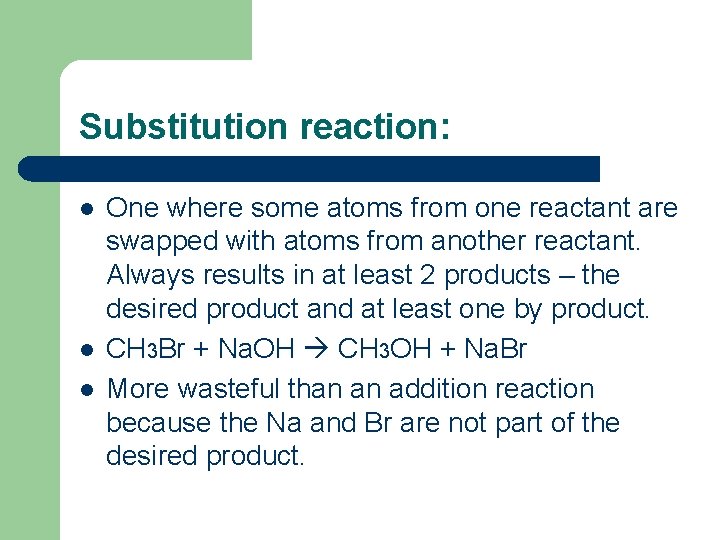
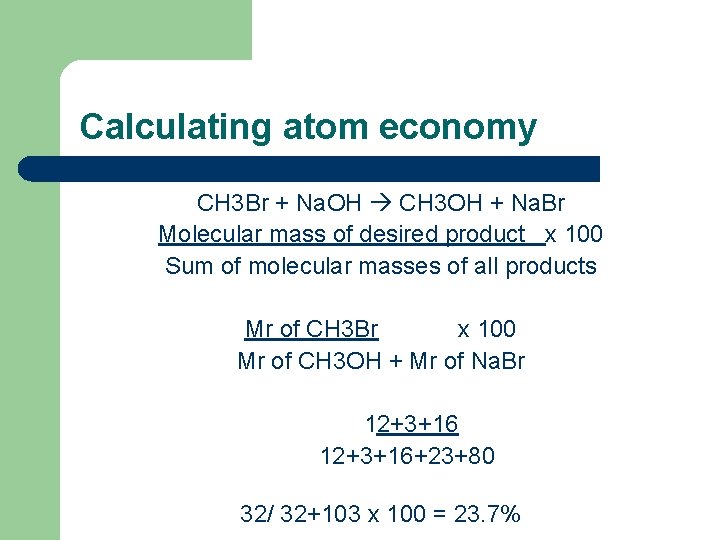
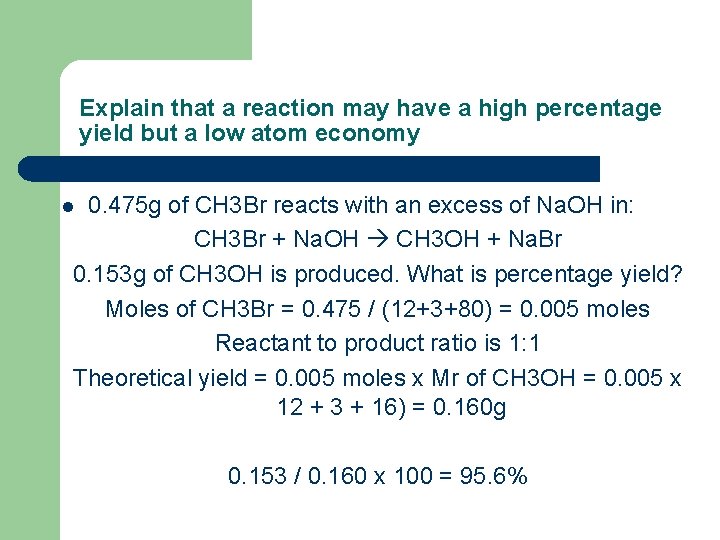
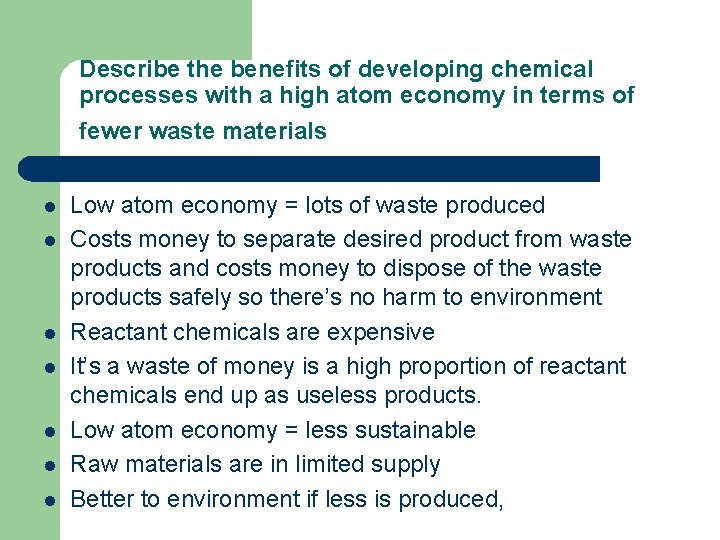
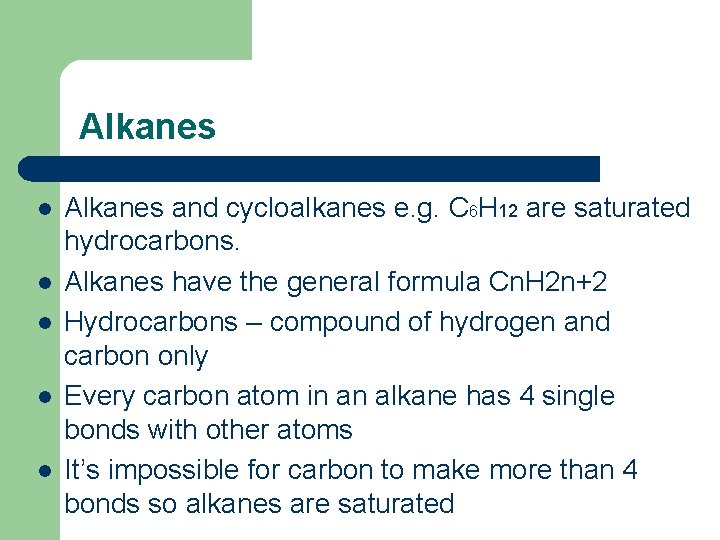
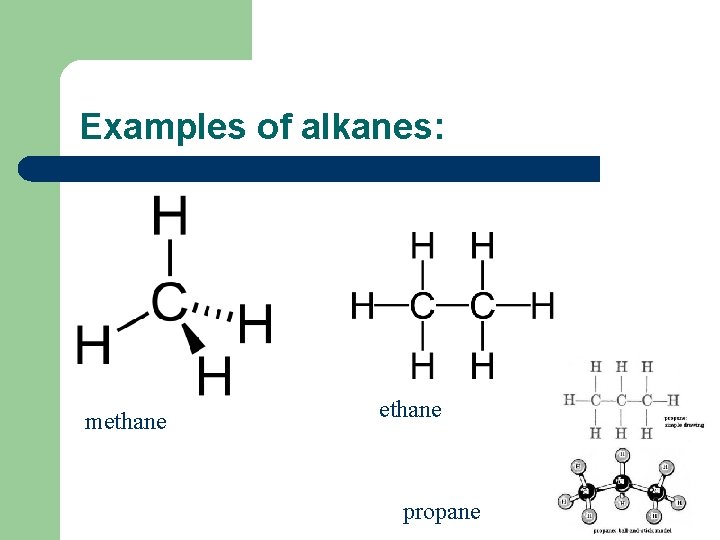
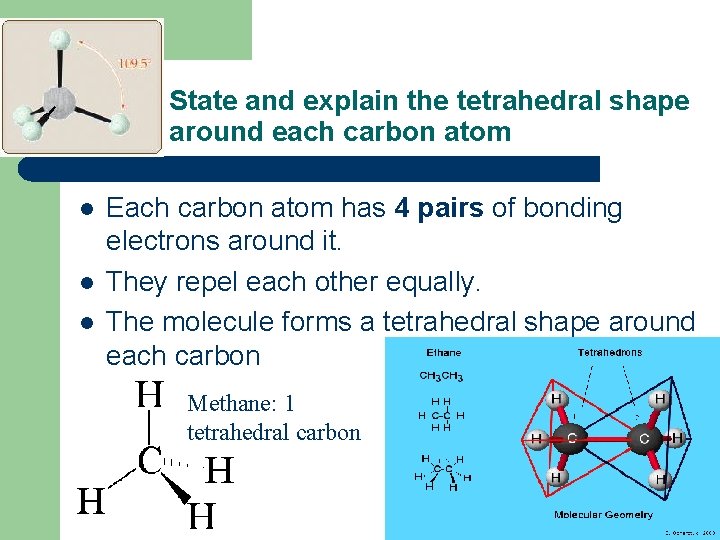
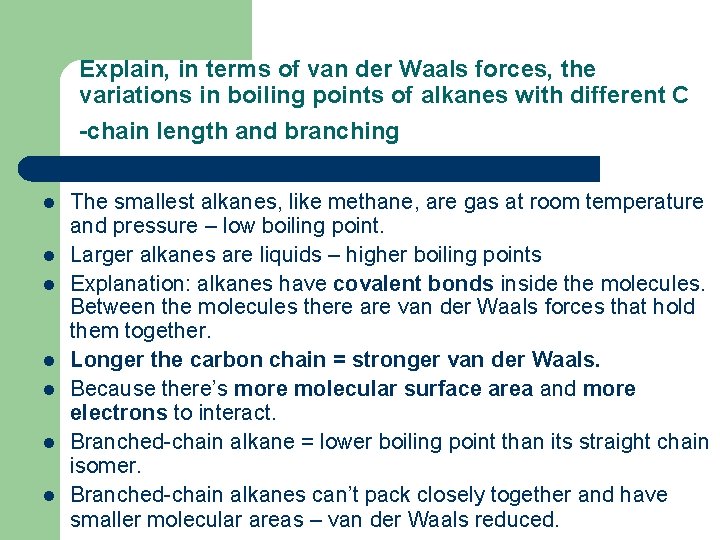
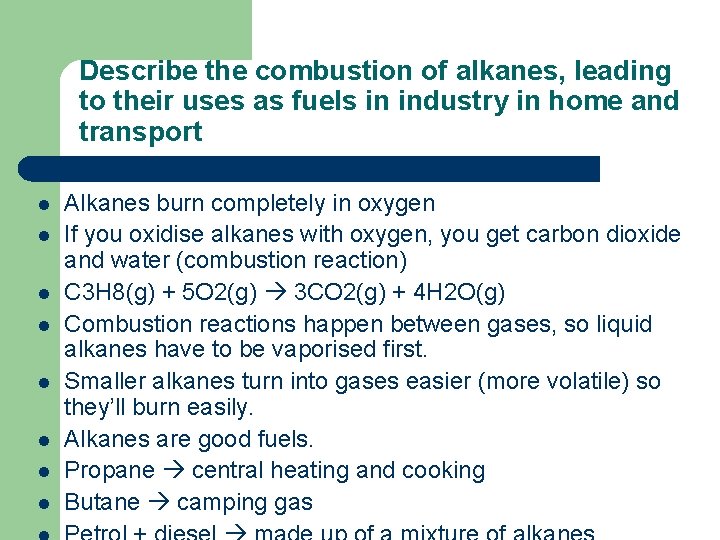
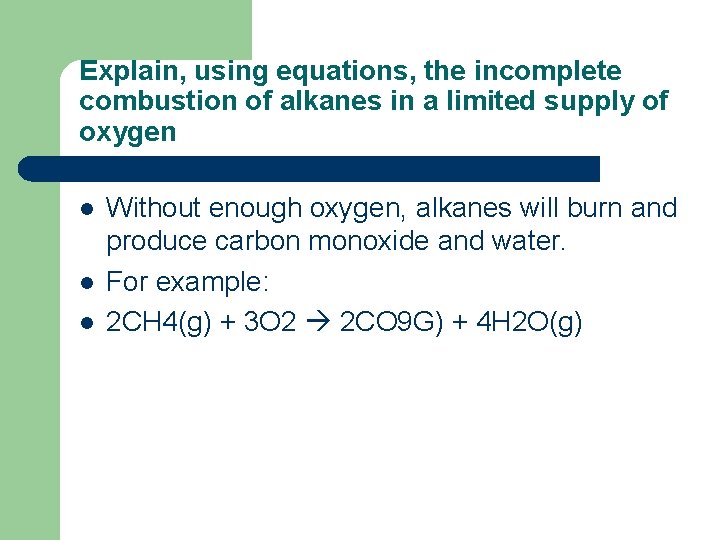
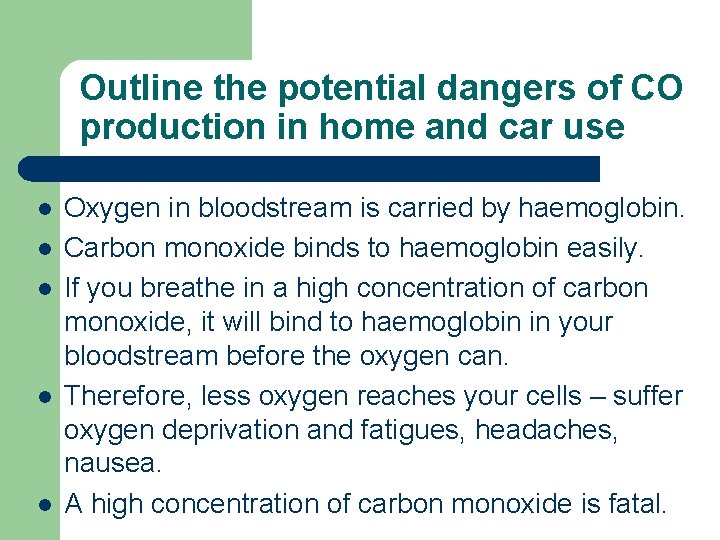
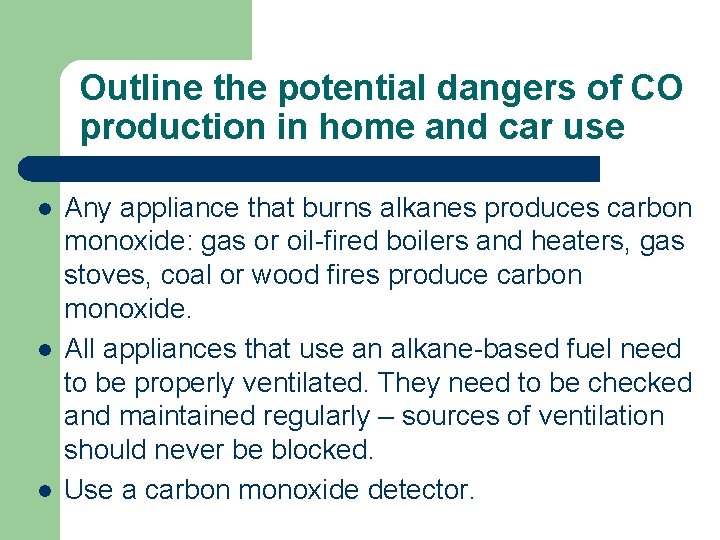
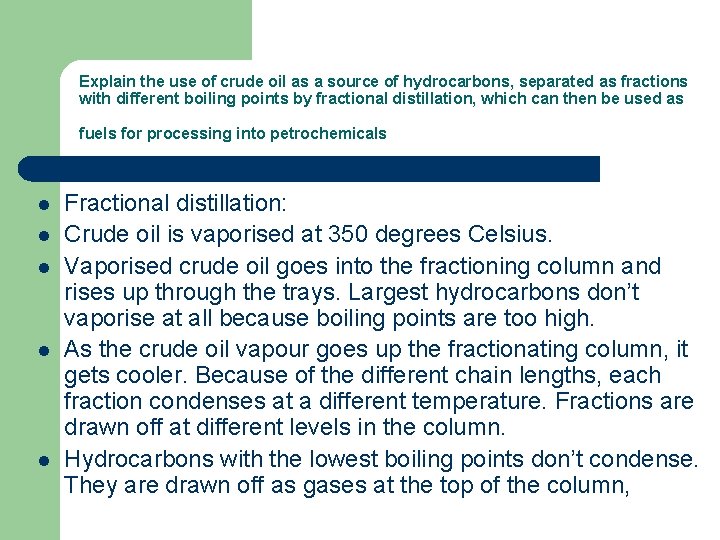
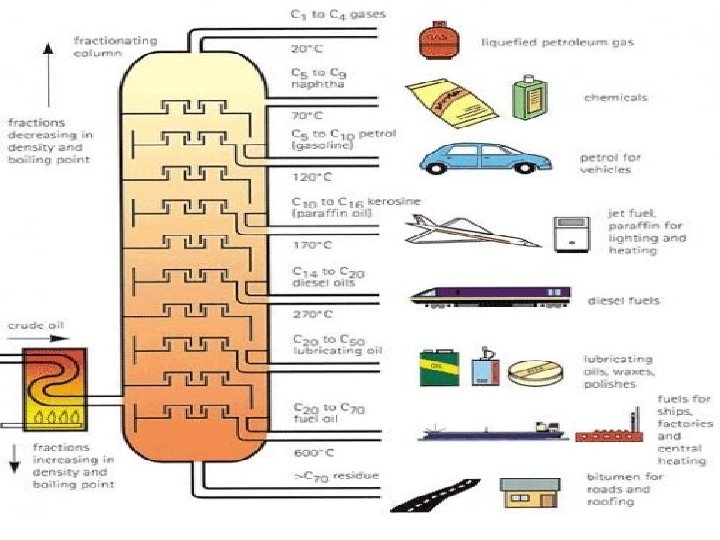
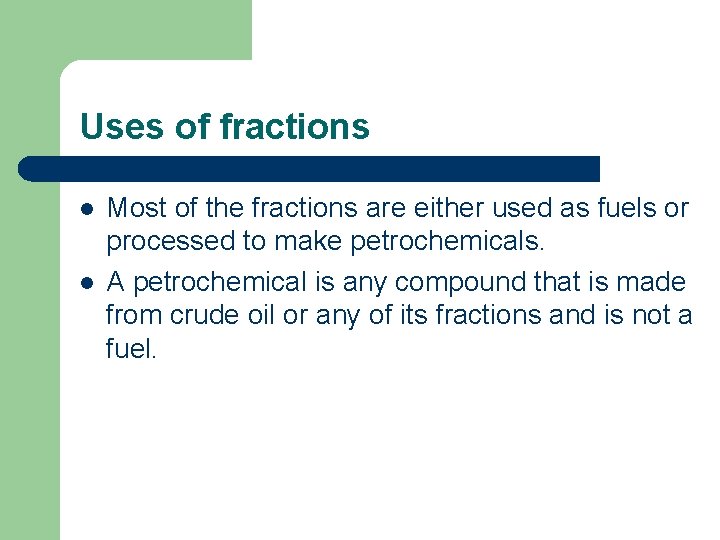
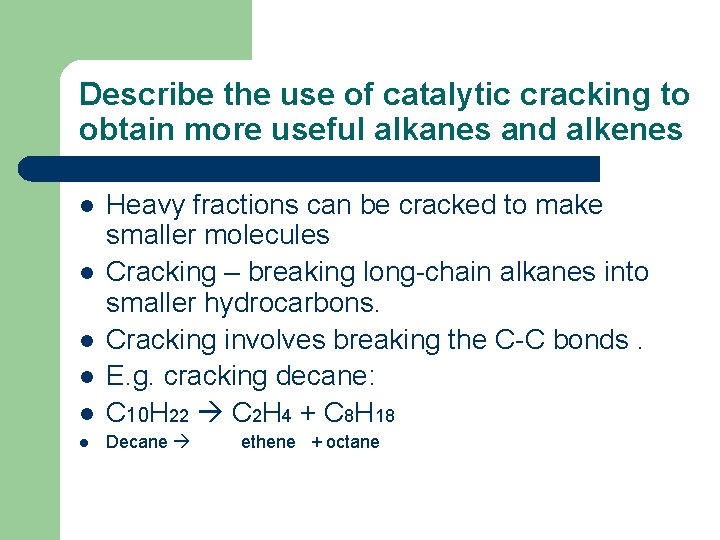
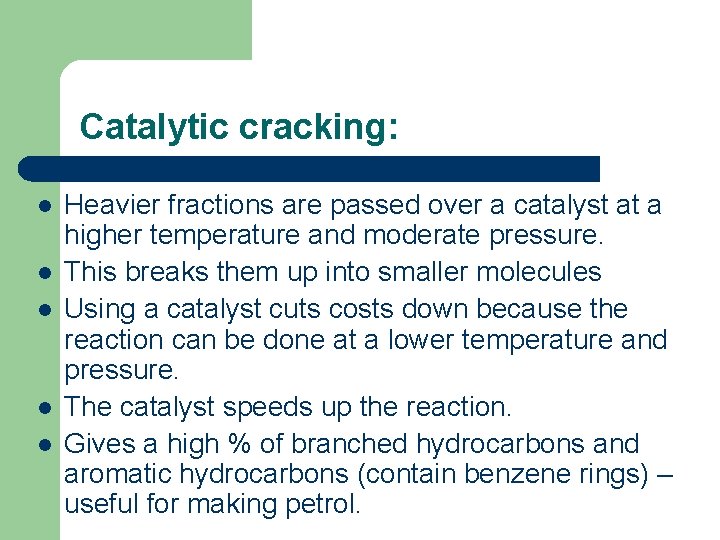
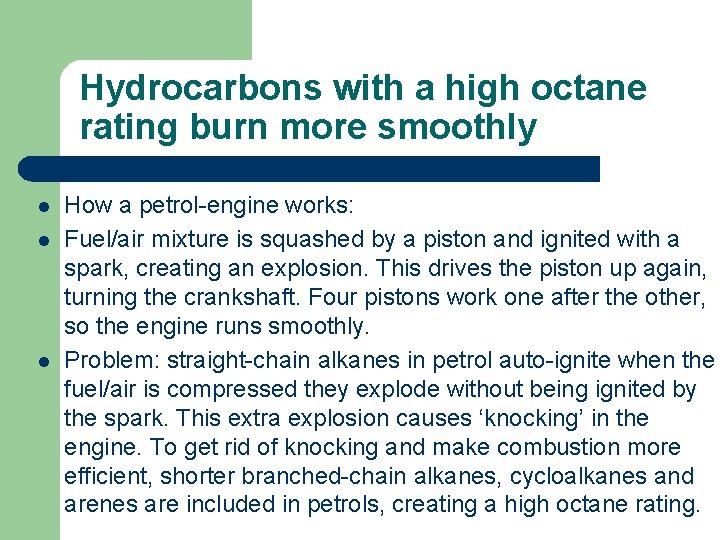
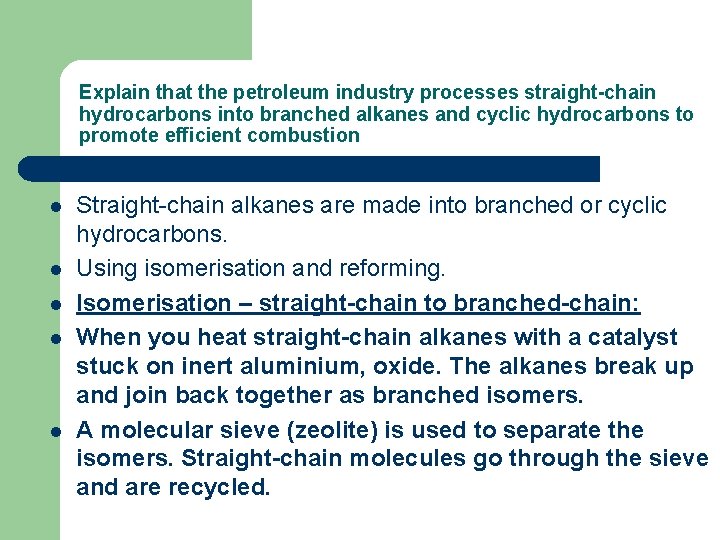
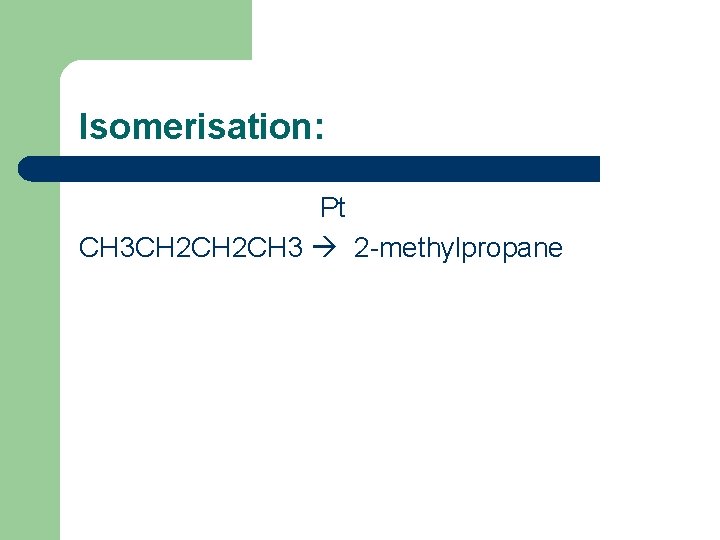
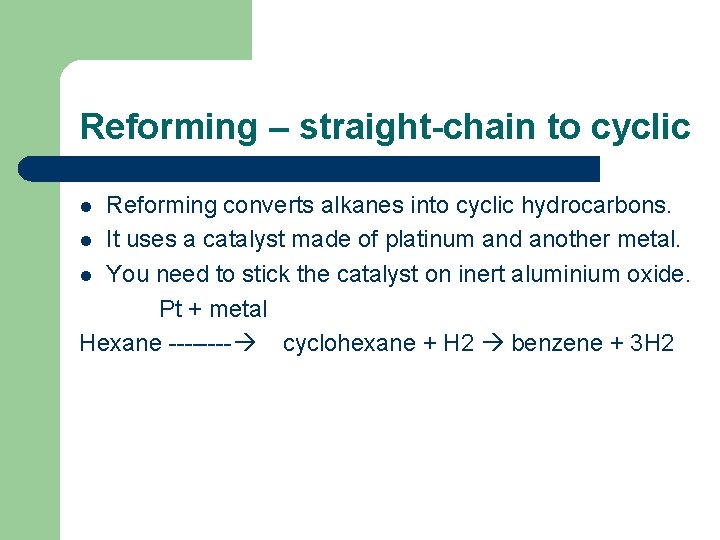
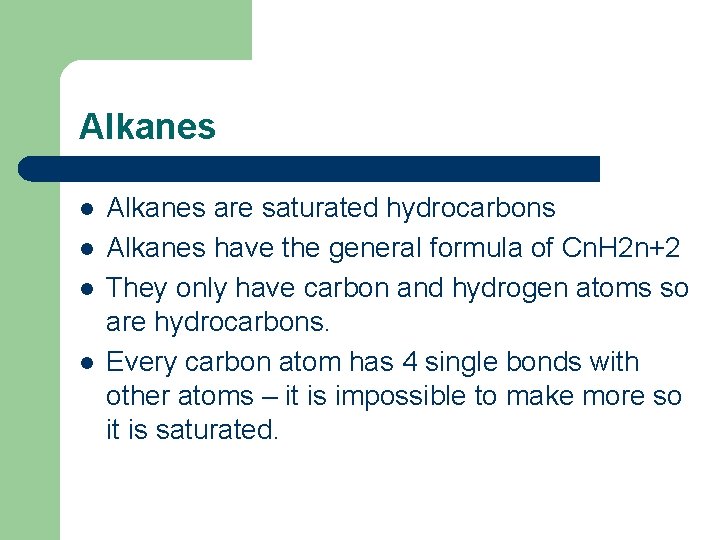
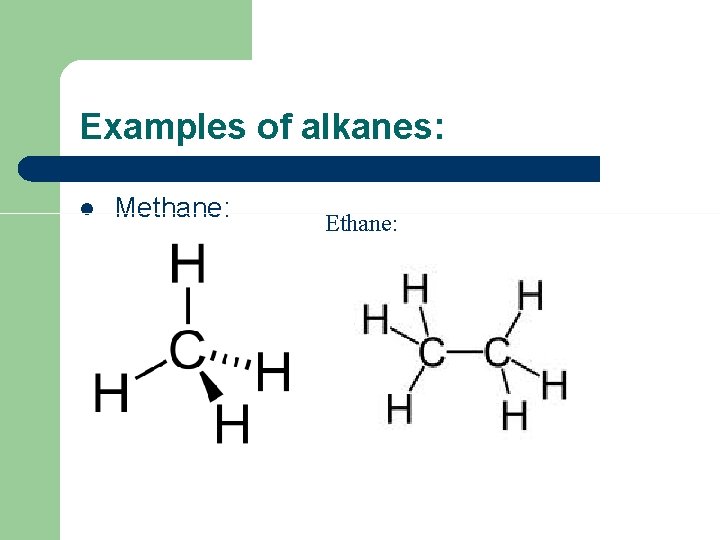
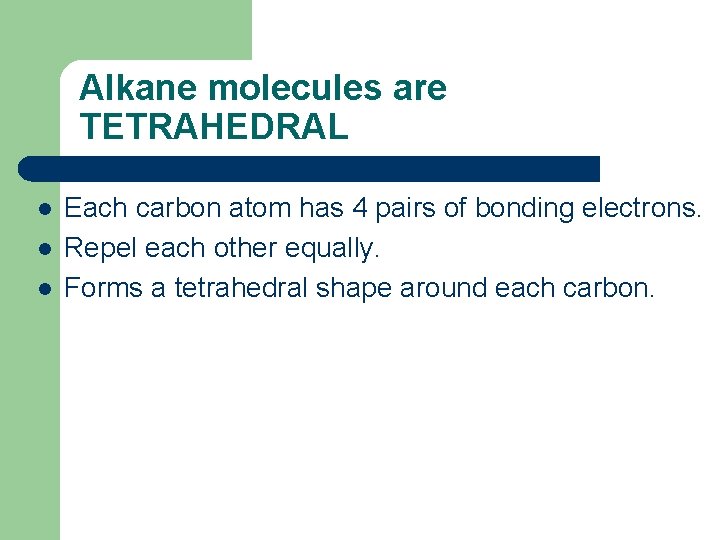
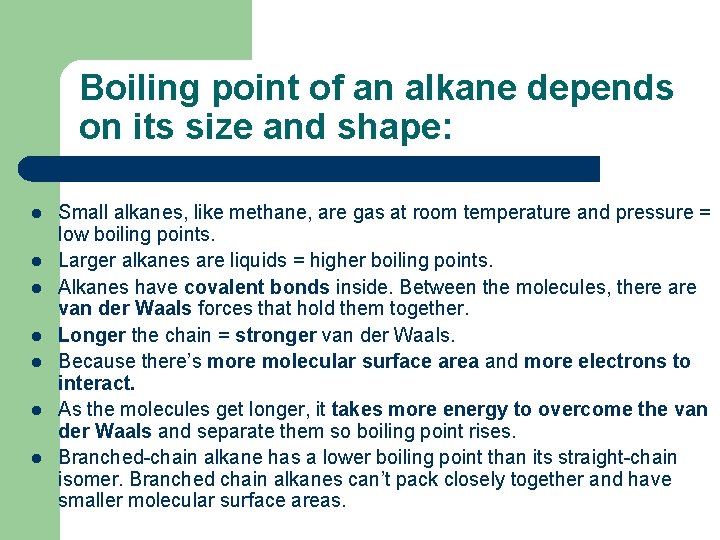
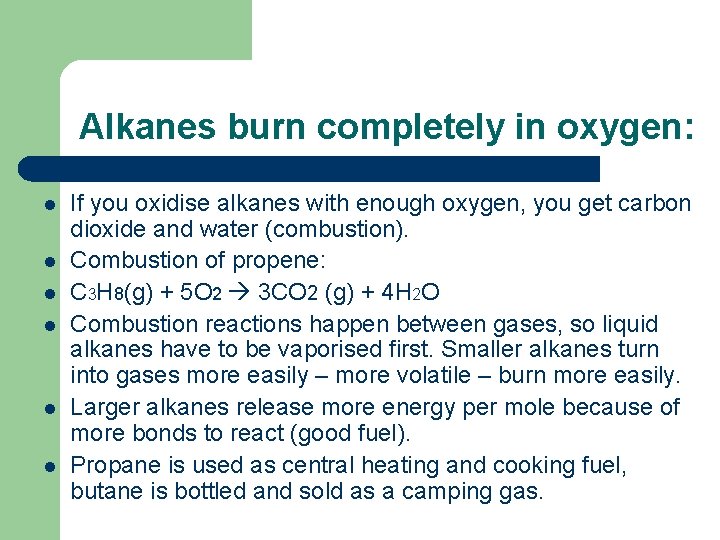
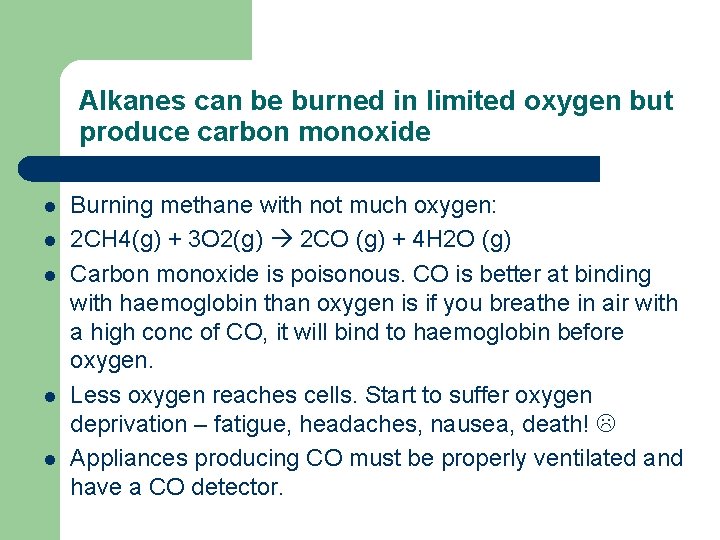
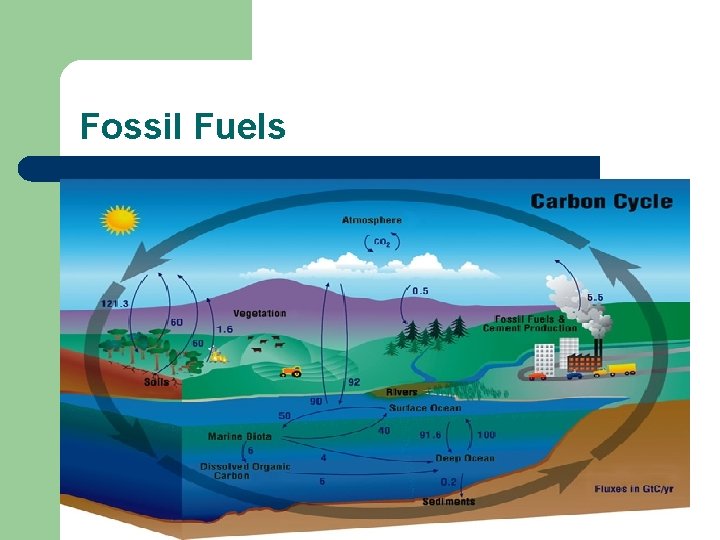
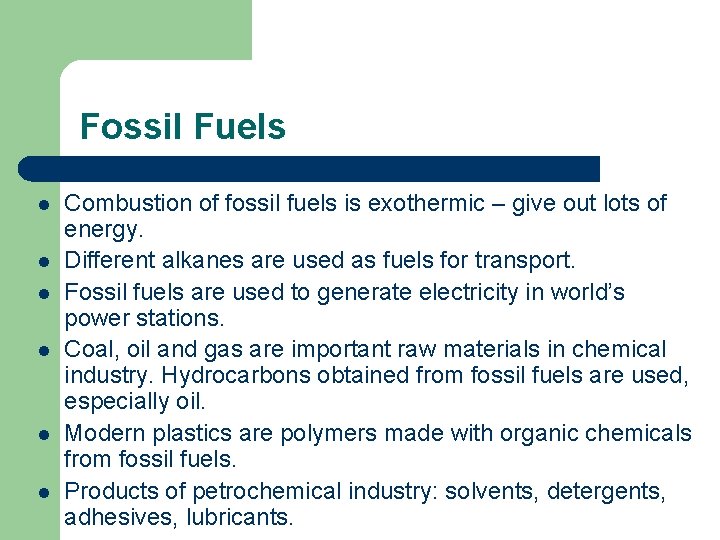
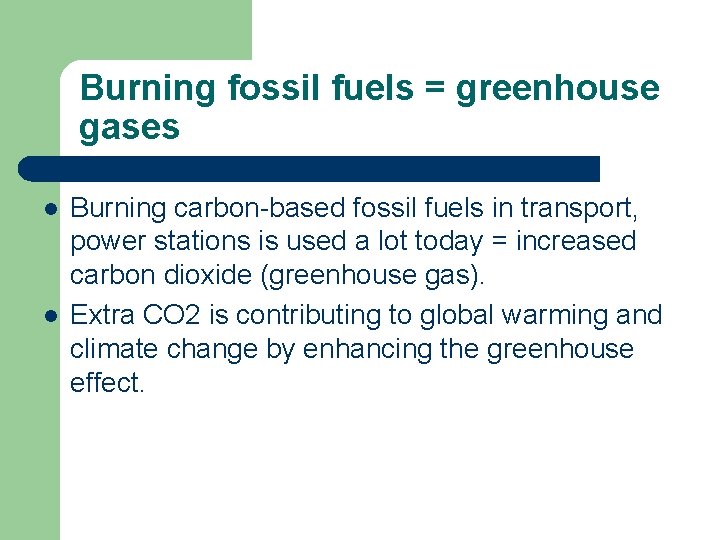
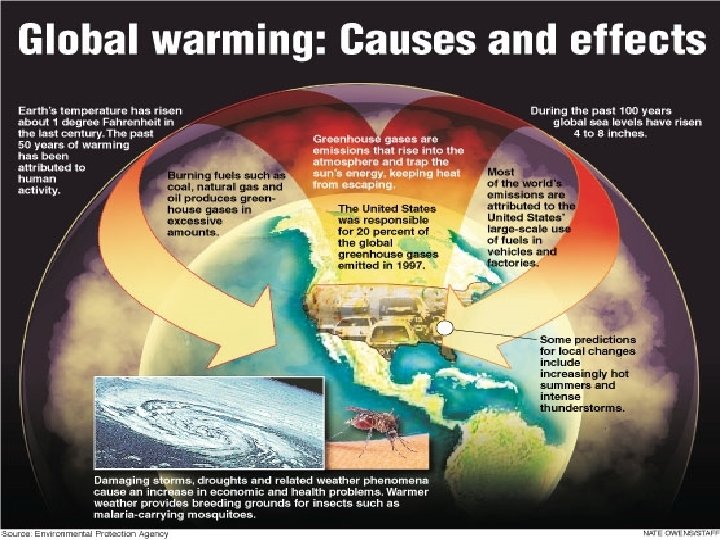
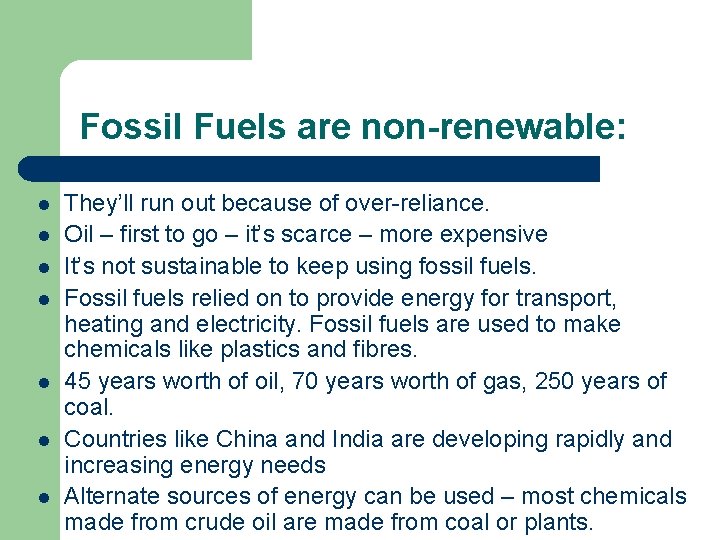
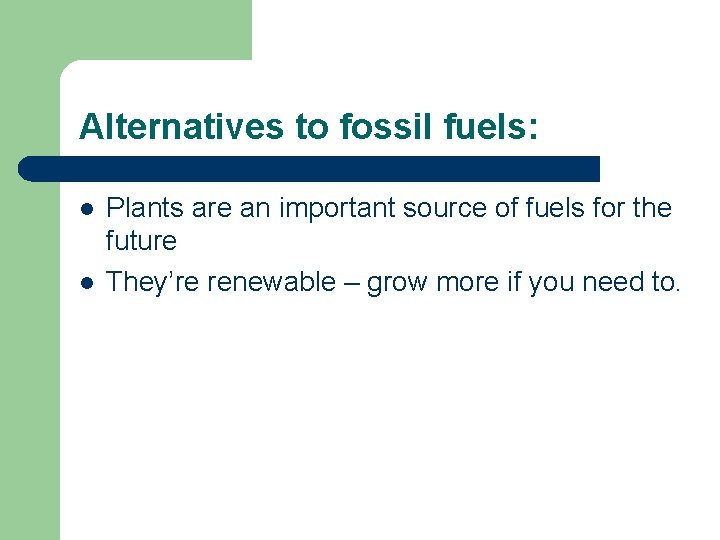
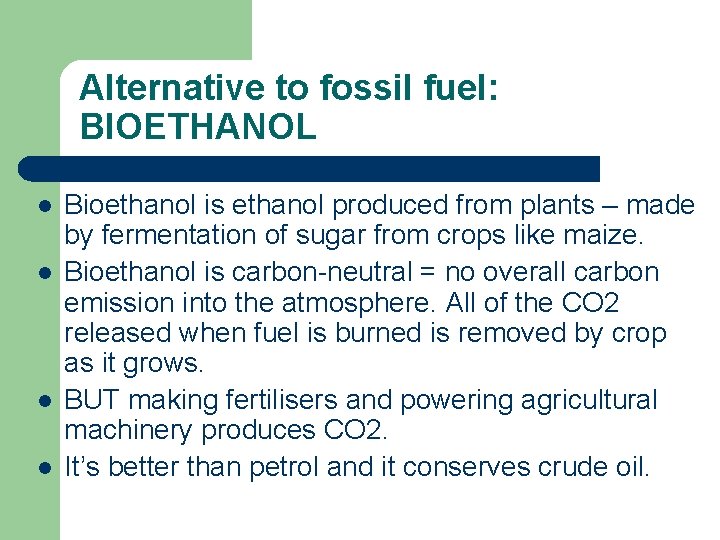
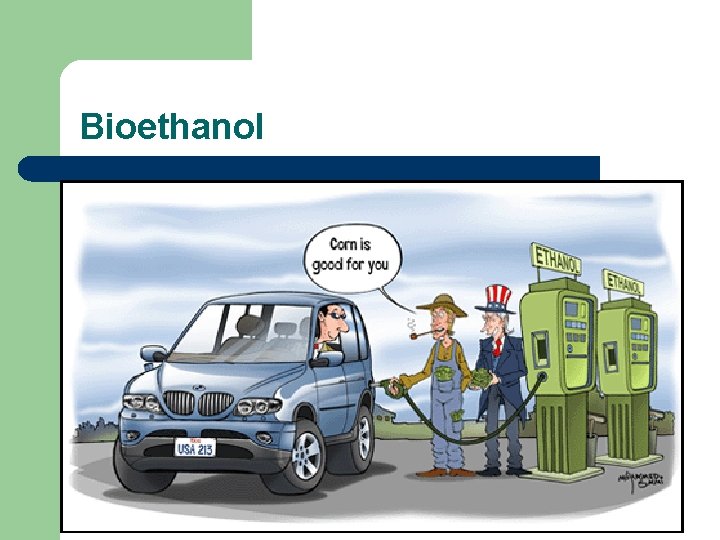
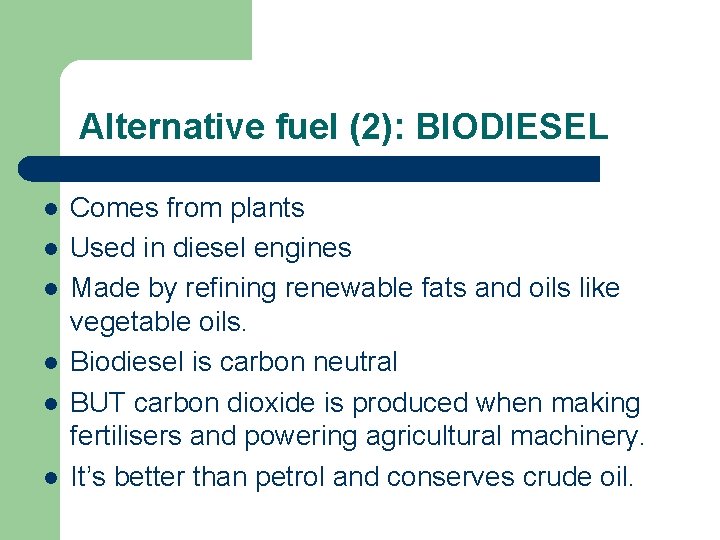
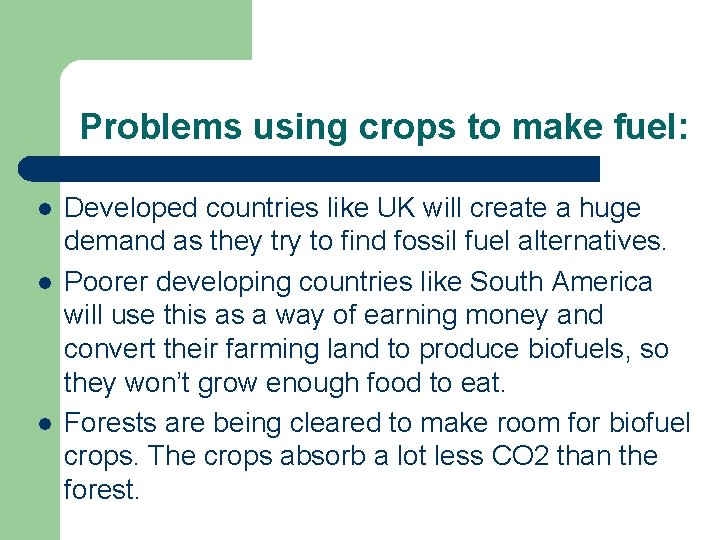
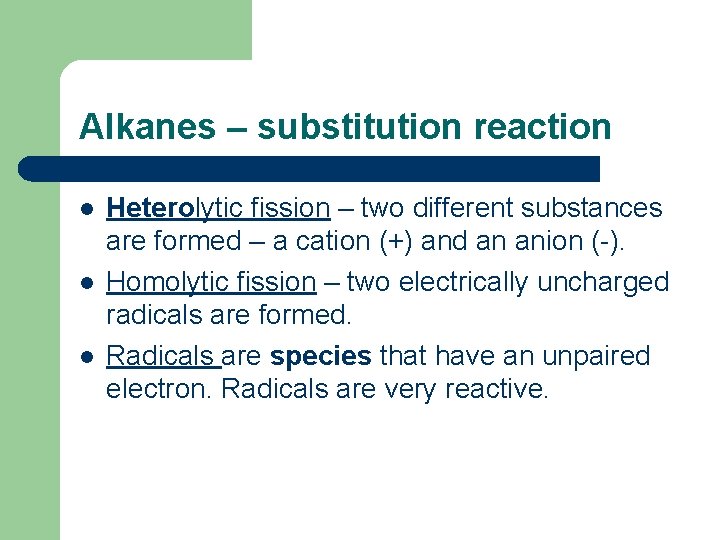
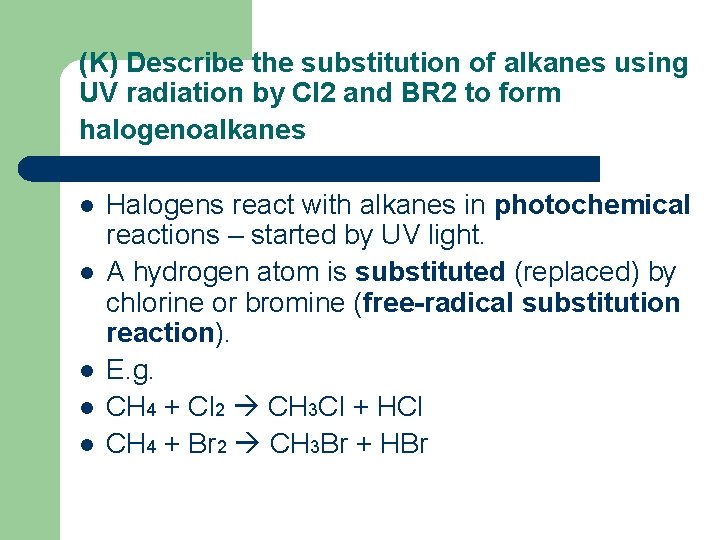
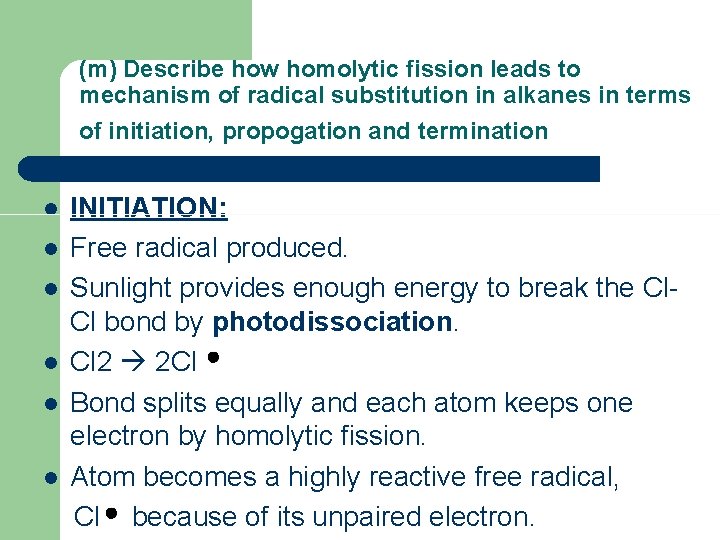
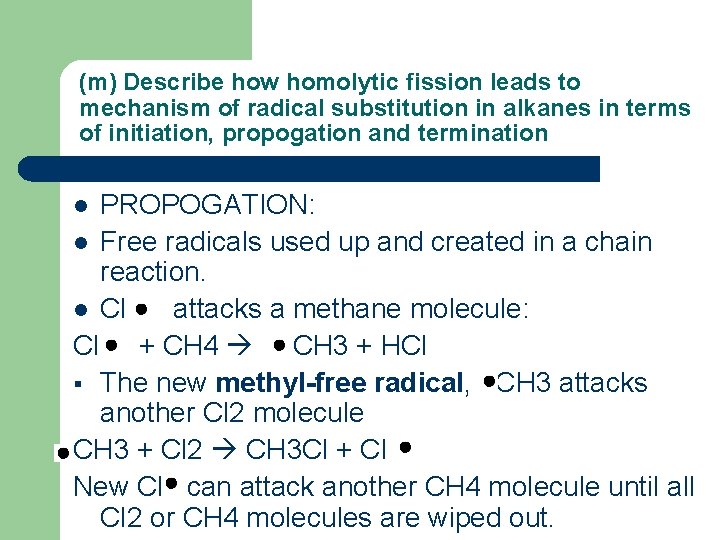
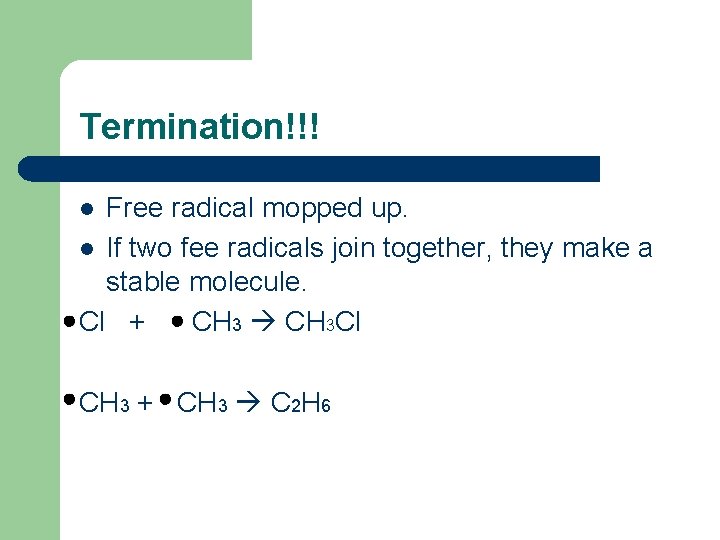
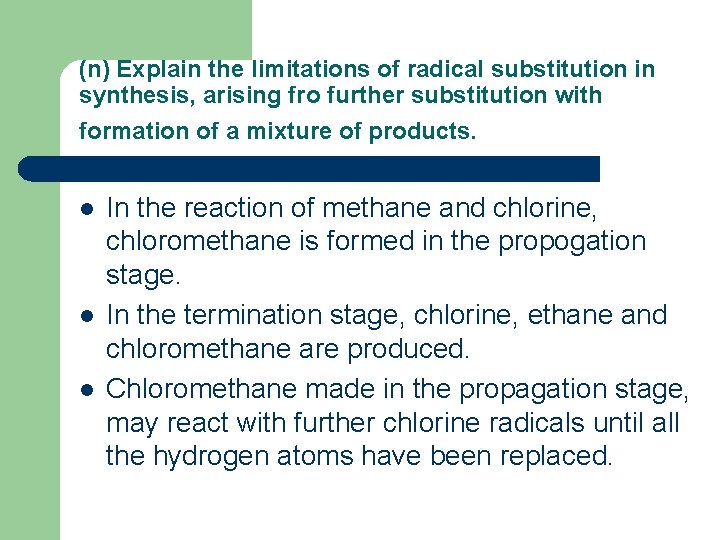
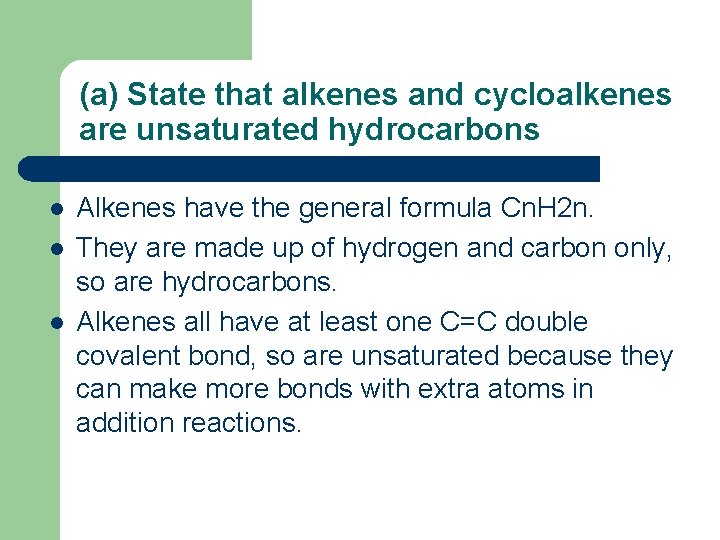
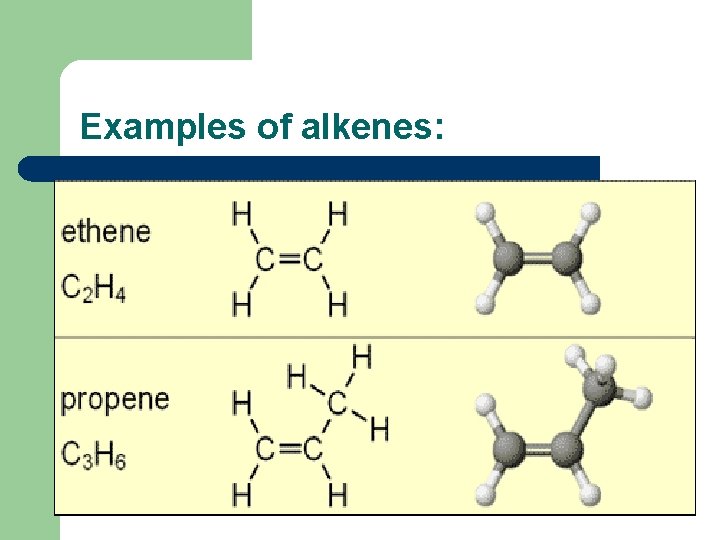
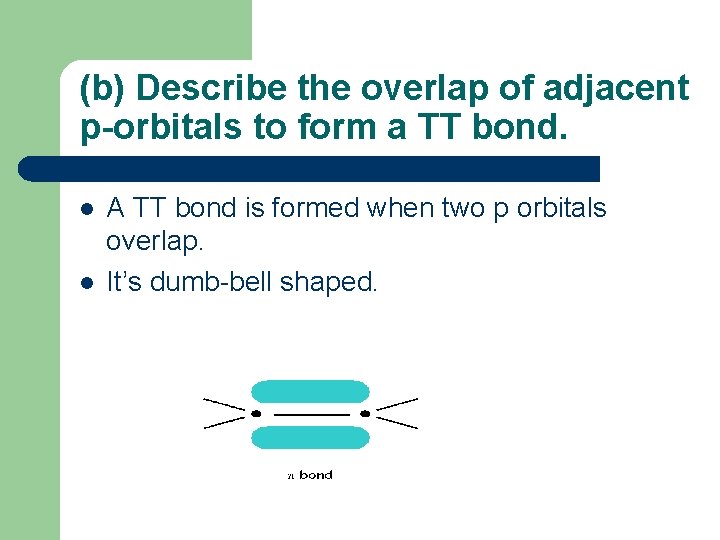
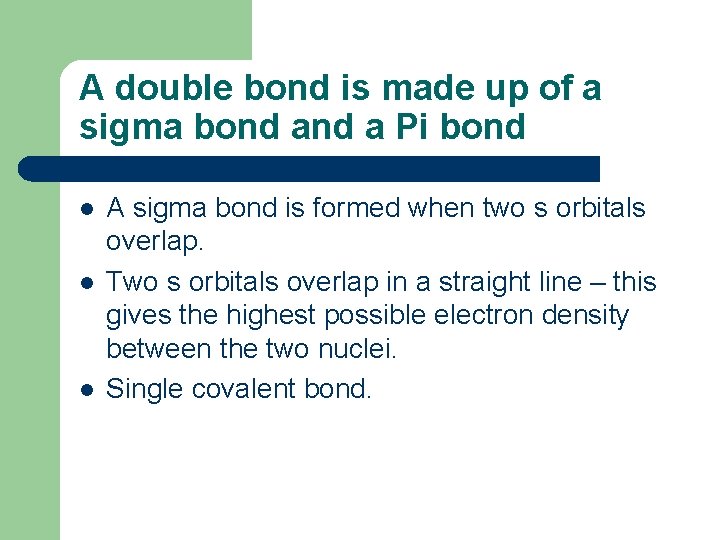
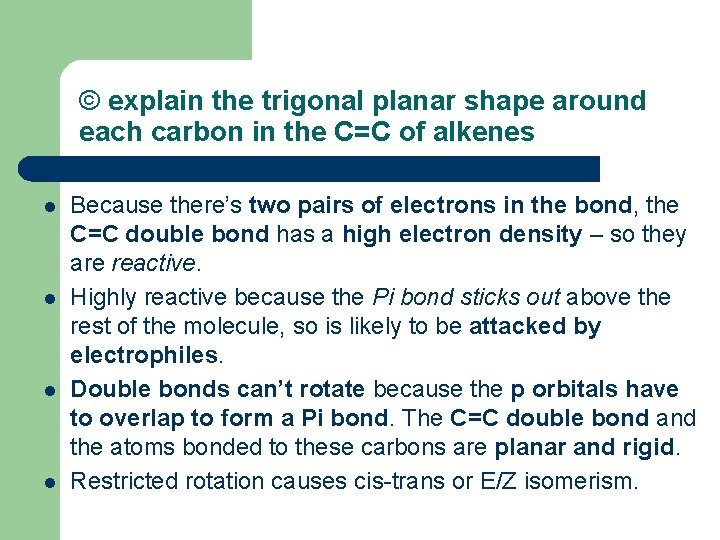
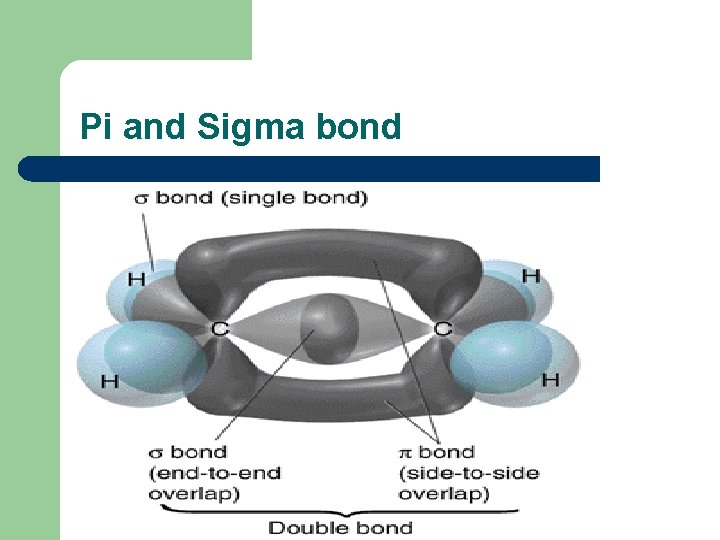
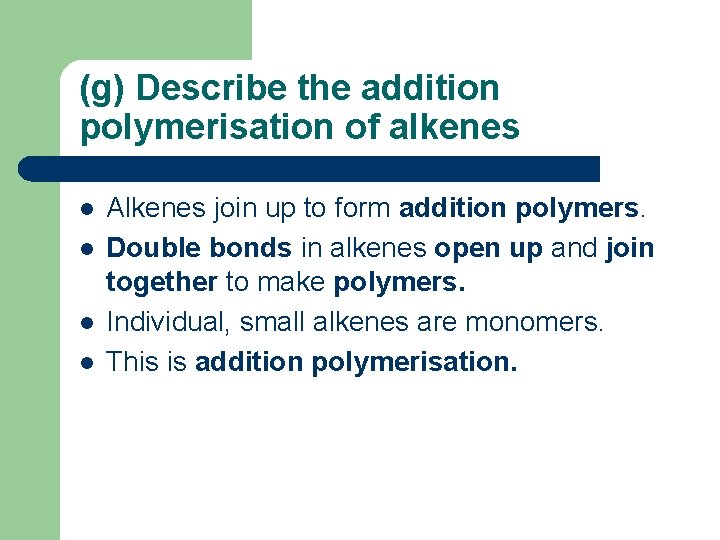
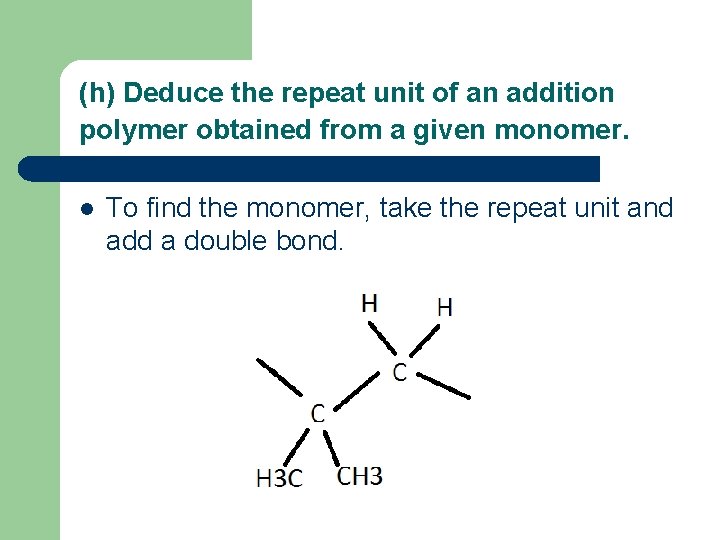
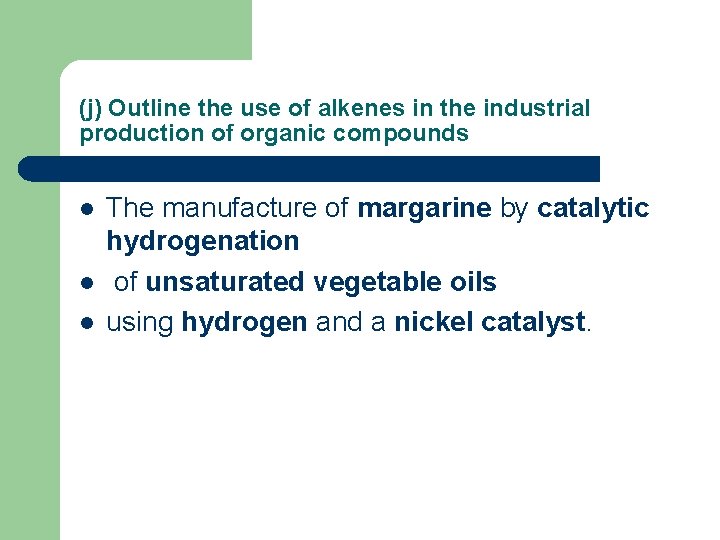
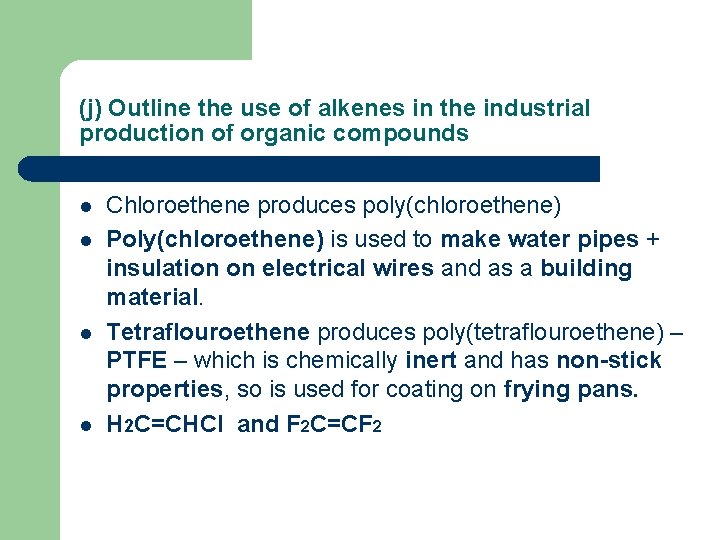
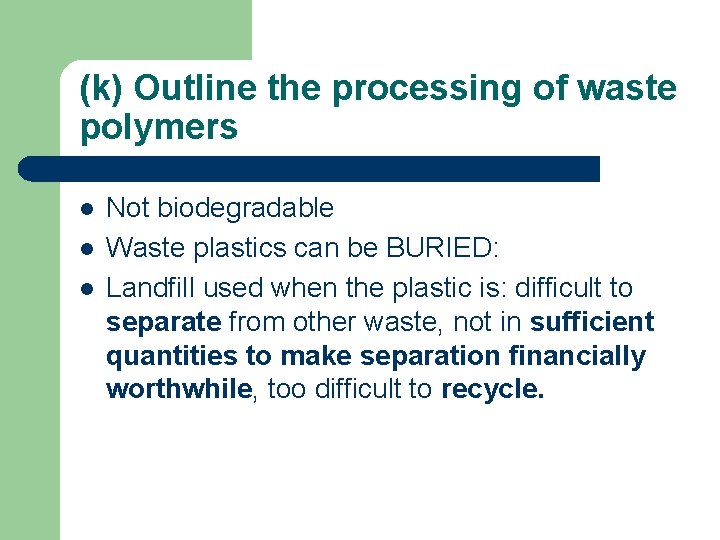
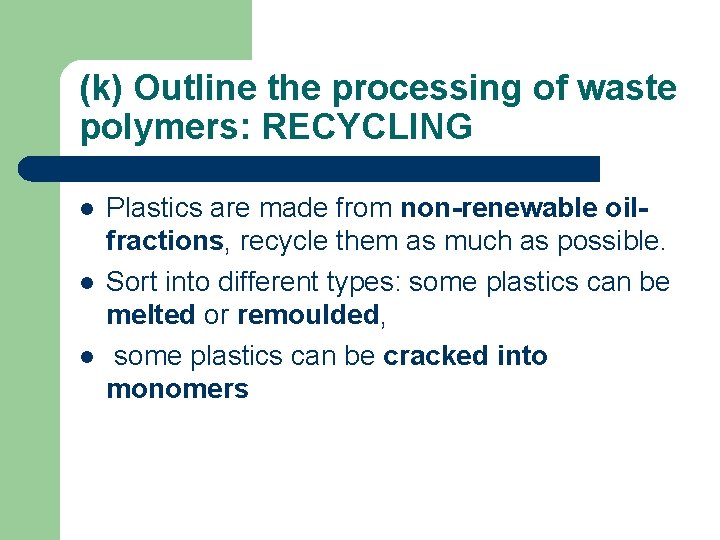
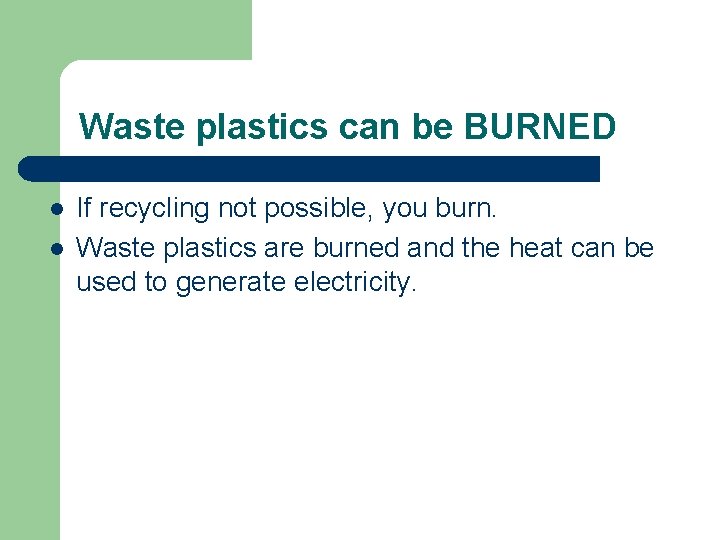
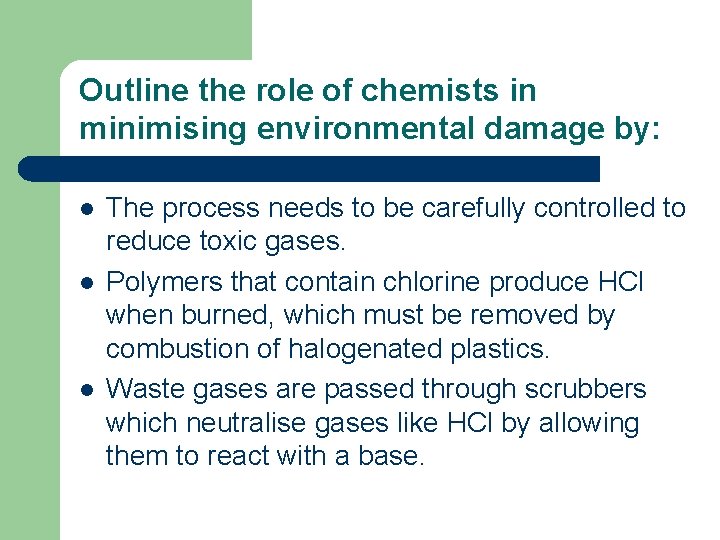
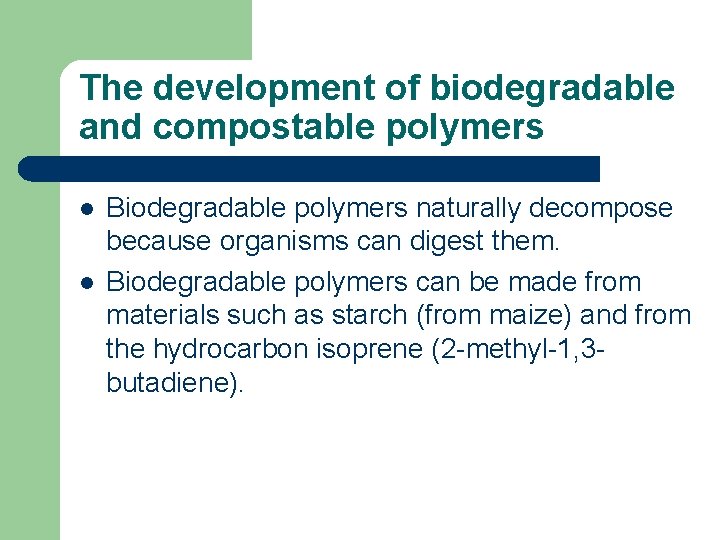
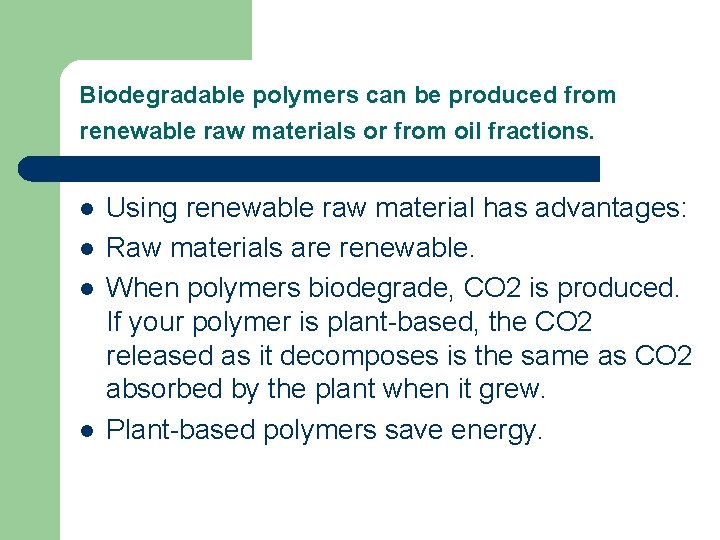
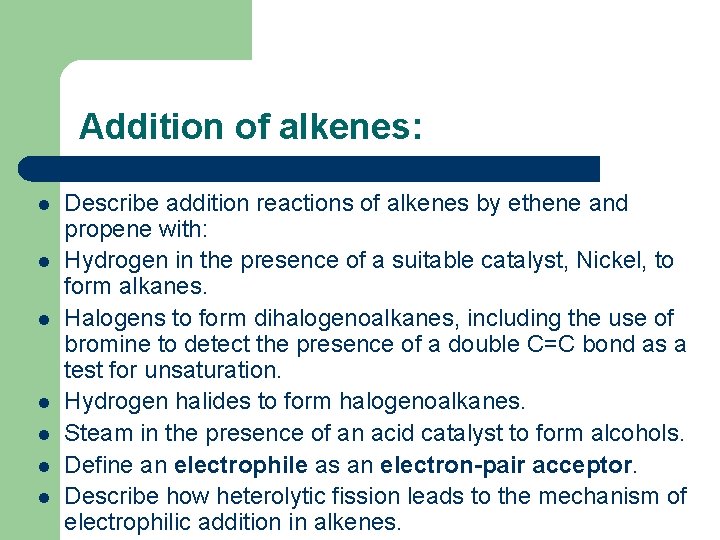
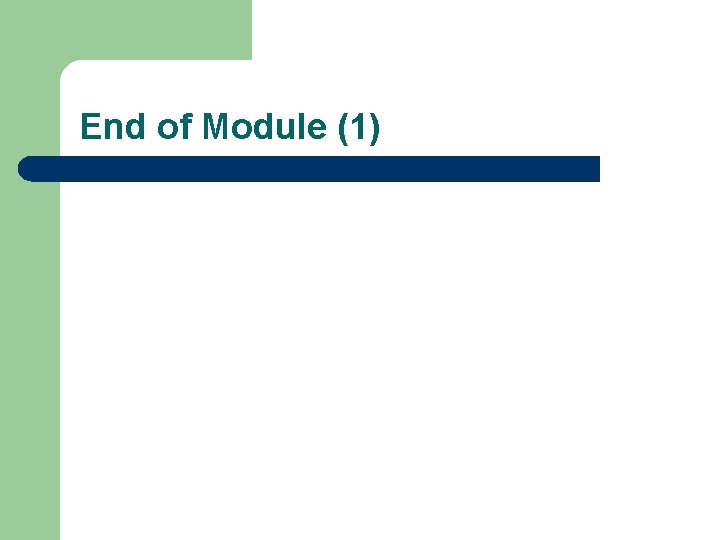
- Slides: 74
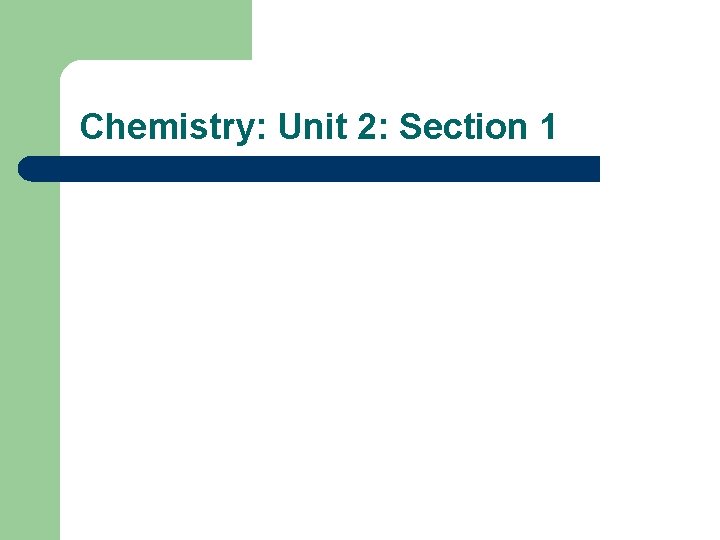
Chemistry: Unit 2: Section 1
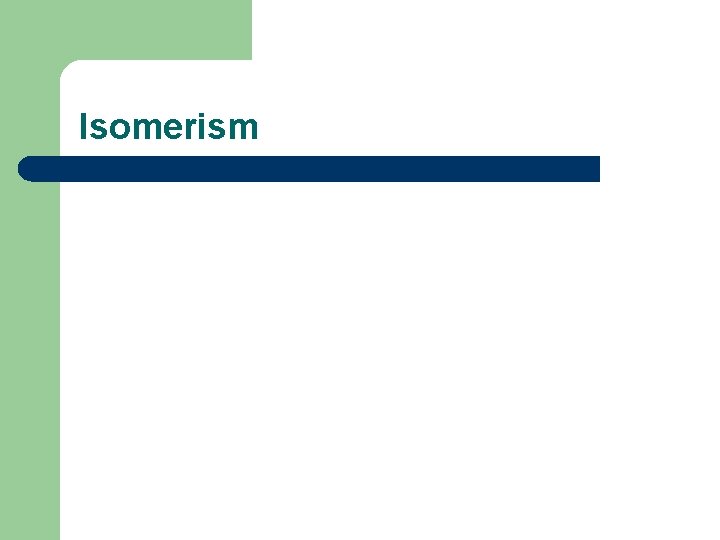
Isomerism
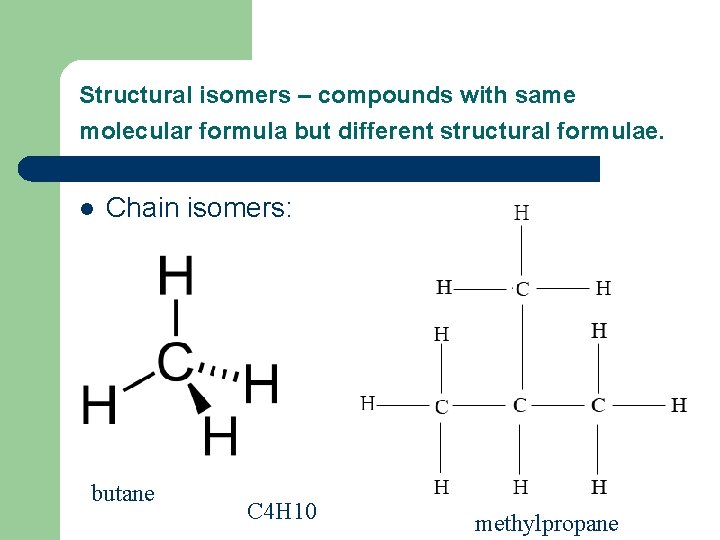
Structural isomers – compounds with same molecular formula but different structural formulae. l Chain isomers: butane C 4 H 10 methylpropane
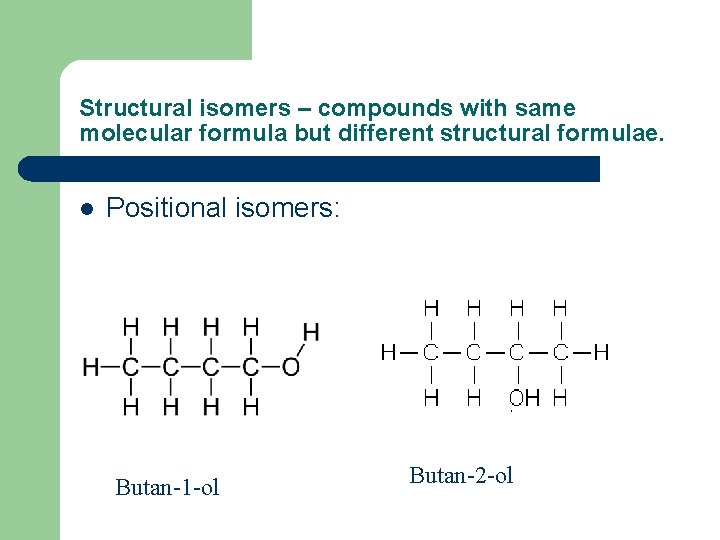
Structural isomers – compounds with same molecular formula but different structural formulae. l Positional isomers: Butan-1 -ol Butan-2 -ol
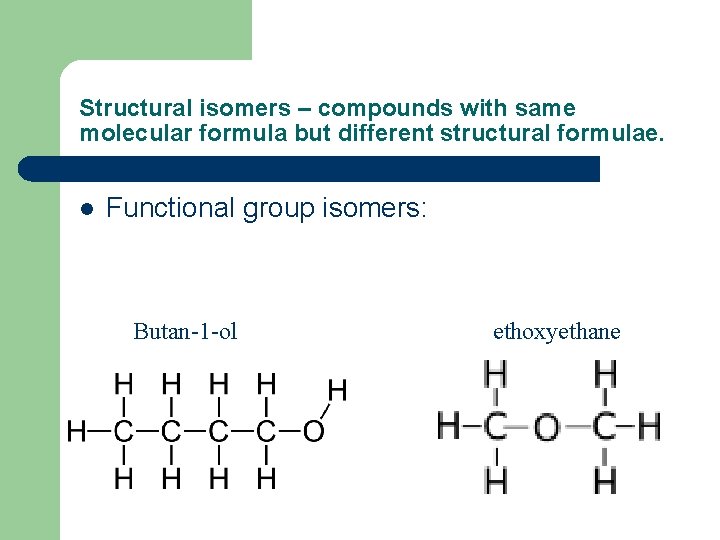
Structural isomers – compounds with same molecular formula but different structural formulae. l Functional group isomers: Butan-1 -ol ethoxyethane
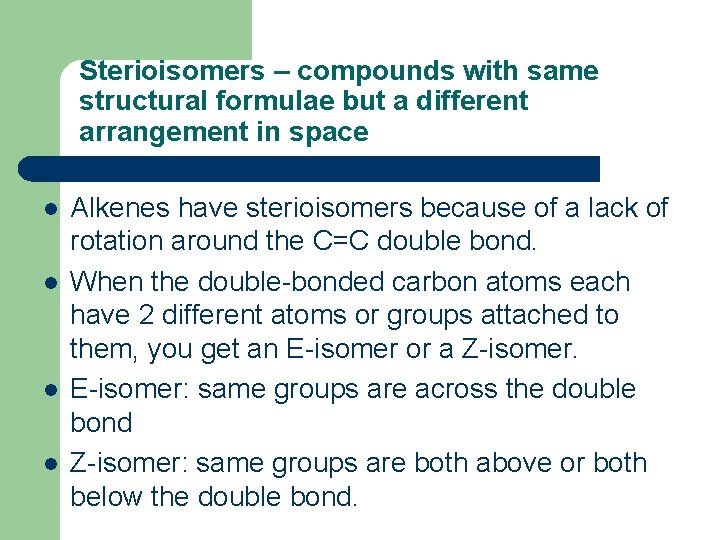
Sterioisomers – compounds with same structural formulae but a different arrangement in space l l Alkenes have sterioisomers because of a lack of rotation around the C=C double bond. When the double-bonded carbon atoms each have 2 different atoms or groups attached to them, you get an E-isomer or a Z-isomer. E-isomer: same groups are across the double bond Z-isomer: same groups are both above or both below the double bond.
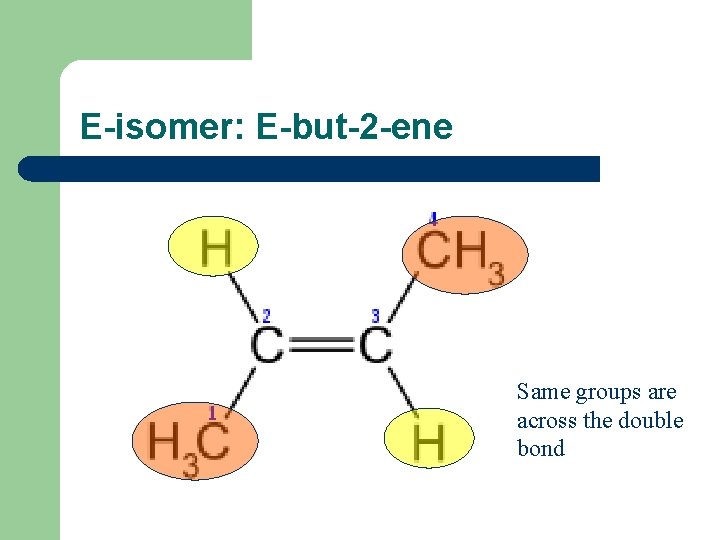
E-isomer: E-but-2 -ene Same groups are across the double bond
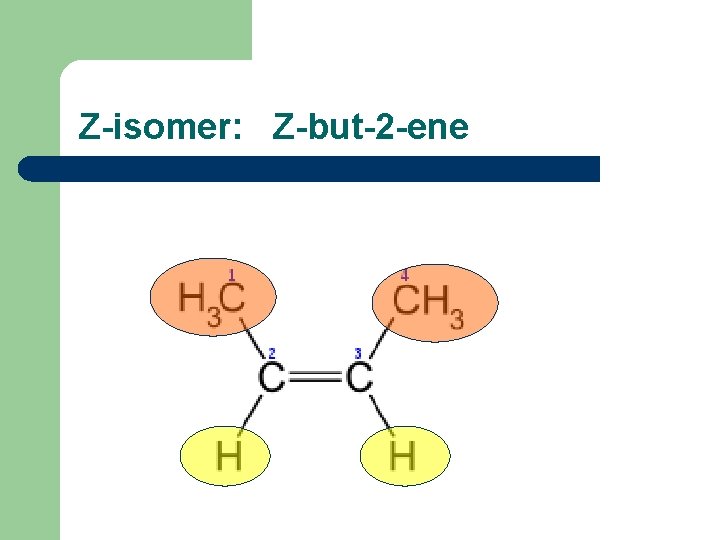
Z-isomer: Z-but-2 -ene
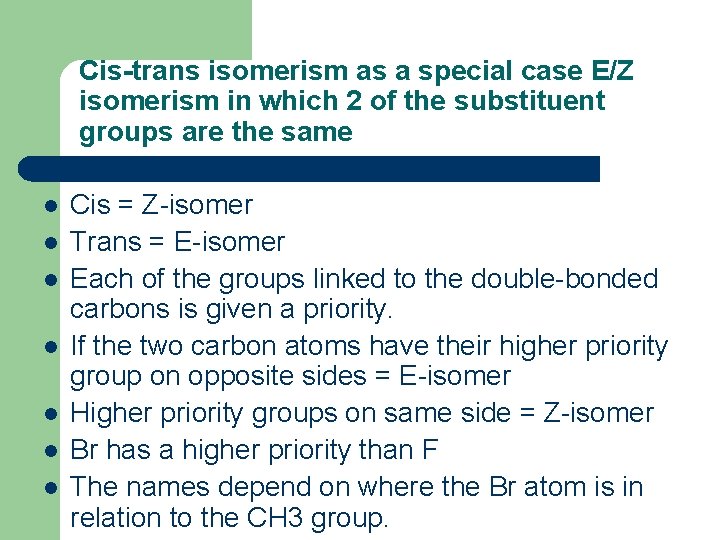
Cis-trans isomerism as a special case E/Z isomerism in which 2 of the substituent groups are the same l l l l Cis = Z-isomer Trans = E-isomer Each of the groups linked to the double-bonded carbons is given a priority. If the two carbon atoms have their higher priority group on opposite sides = E-isomer Higher priority groups on same side = Z-isomer Br has a higher priority than F The names depend on where the Br atom is in relation to the CH 3 group.
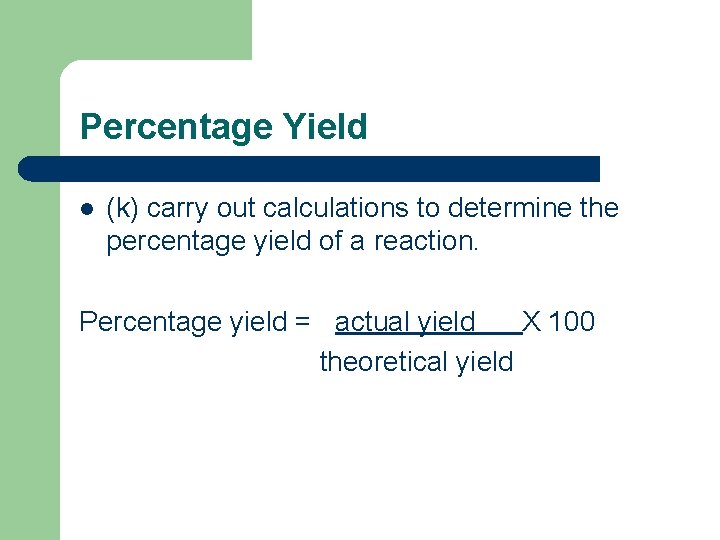
Percentage Yield l (k) carry out calculations to determine the percentage yield of a reaction. Percentage yield = actual yield X 100 theoretical yield
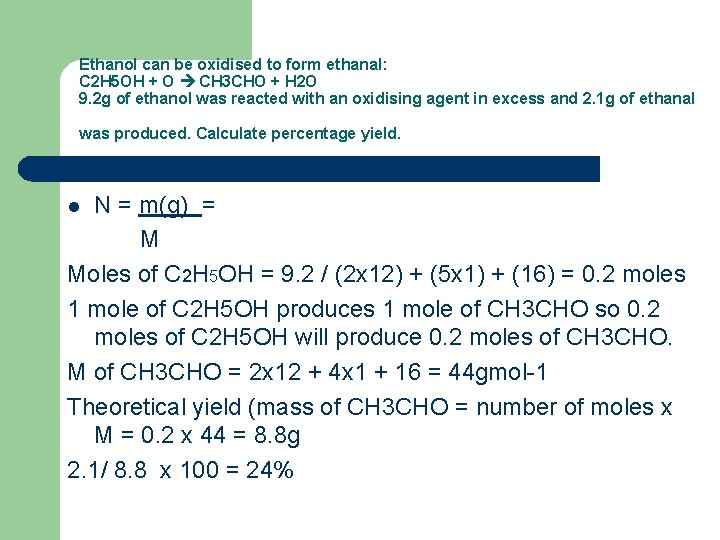
Ethanol can be oxidised to form ethanal: C 2 H 5 OH + O CH 3 CHO + H 2 O 9. 2 g of ethanol was reacted with an oxidising agent in excess and 2. 1 g of ethanal was produced. Calculate percentage yield. N = m(g) = M Moles of C 2 H 5 OH = 9. 2 / (2 x 12) + (5 x 1) + (16) = 0. 2 moles 1 mole of C 2 H 5 OH produces 1 mole of CH 3 CHO so 0. 2 moles of C 2 H 5 OH will produce 0. 2 moles of CH 3 CHO. M of CH 3 CHO = 2 x 12 + 4 x 1 + 16 = 44 gmol-1 Theoretical yield (mass of CH 3 CHO = number of moles x M = 0. 2 x 44 = 8. 8 g 2. 1/ 8. 8 x 100 = 24% l
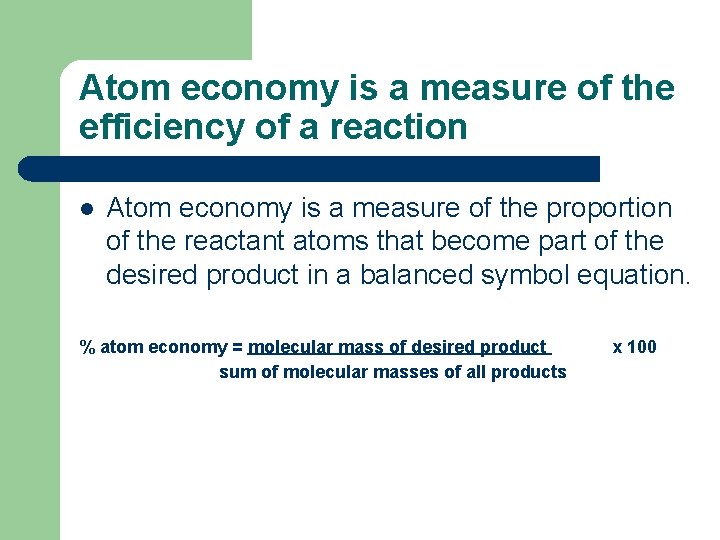
Atom economy is a measure of the efficiency of a reaction l Atom economy is a measure of the proportion of the reactant atoms that become part of the desired product in a balanced symbol equation. % atom economy = molecular mass of desired product sum of molecular masses of all products x 100
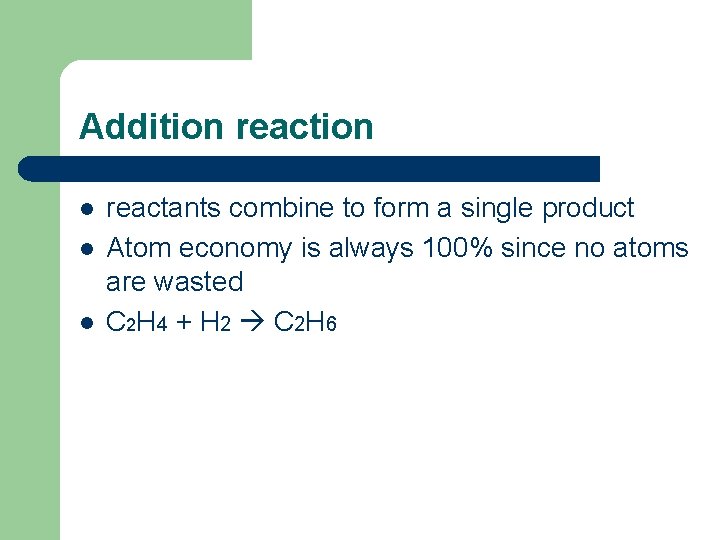
Addition reaction l l l reactants combine to form a single product Atom economy is always 100% since no atoms are wasted C 2 H 4 + H 2 C 2 H 6
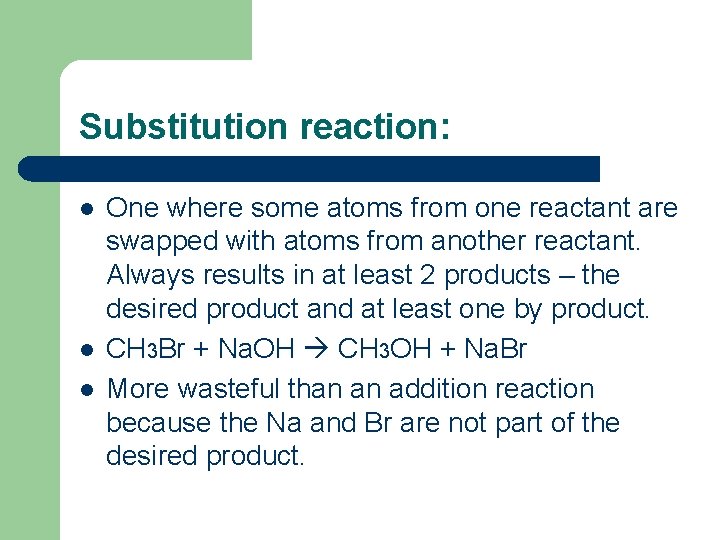
Substitution reaction: l l l One where some atoms from one reactant are swapped with atoms from another reactant. Always results in at least 2 products – the desired product and at least one by product. CH 3 Br + Na. OH CH 3 OH + Na. Br More wasteful than an addition reaction because the Na and Br are not part of the desired product.
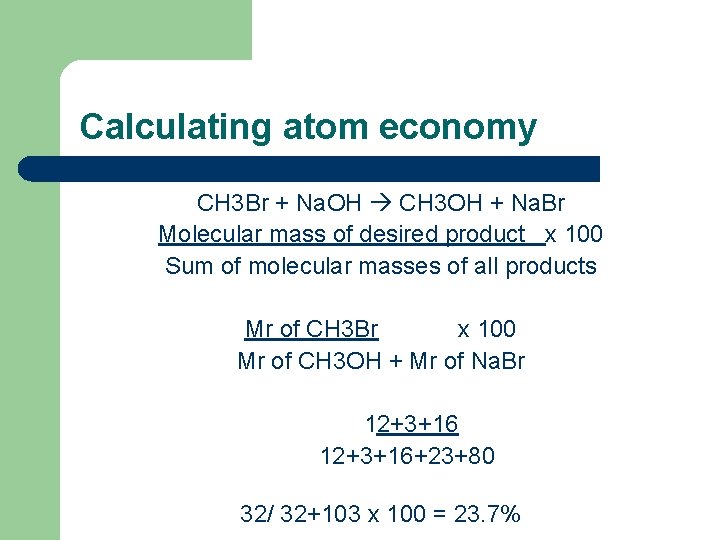
Calculating atom economy CH 3 Br + Na. OH CH 3 OH + Na. Br Molecular mass of desired product x 100 Sum of molecular masses of all products Mr of CH 3 Br x 100 Mr of CH 3 OH + Mr of Na. Br 12+3+16+23+80 32/ 32+103 x 100 = 23. 7%
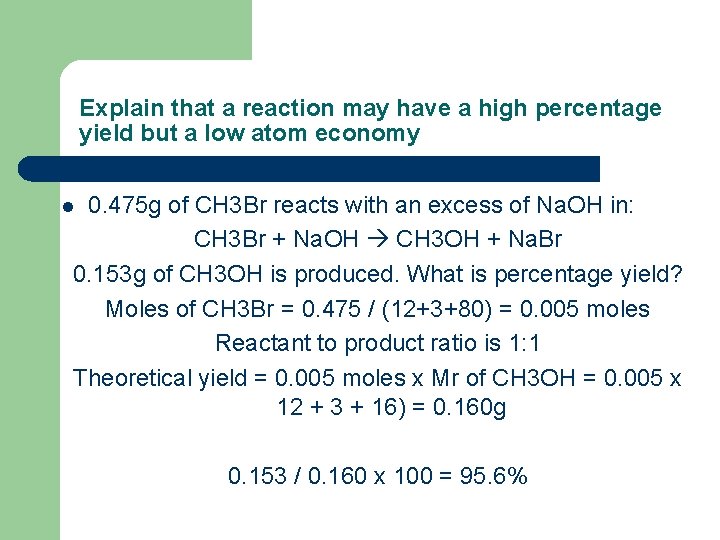
Explain that a reaction may have a high percentage yield but a low atom economy 0. 475 g of CH 3 Br reacts with an excess of Na. OH in: CH 3 Br + Na. OH CH 3 OH + Na. Br 0. 153 g of CH 3 OH is produced. What is percentage yield? Moles of CH 3 Br = 0. 475 / (12+3+80) = 0. 005 moles Reactant to product ratio is 1: 1 Theoretical yield = 0. 005 moles x Mr of CH 3 OH = 0. 005 x 12 + 3 + 16) = 0. 160 g l 0. 153 / 0. 160 x 100 = 95. 6%
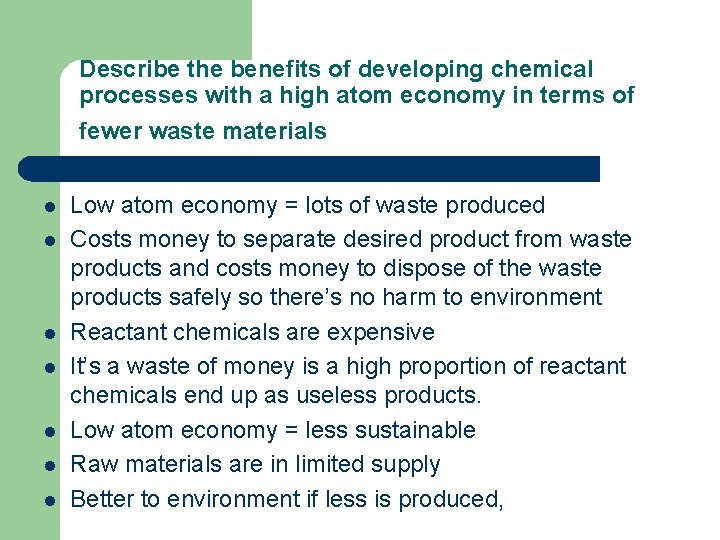
Describe the benefits of developing chemical processes with a high atom economy in terms of fewer waste materials l l l l Low atom economy = lots of waste produced Costs money to separate desired product from waste products and costs money to dispose of the waste products safely so there’s no harm to environment Reactant chemicals are expensive It’s a waste of money is a high proportion of reactant chemicals end up as useless products. Low atom economy = less sustainable Raw materials are in limited supply Better to environment if less is produced,
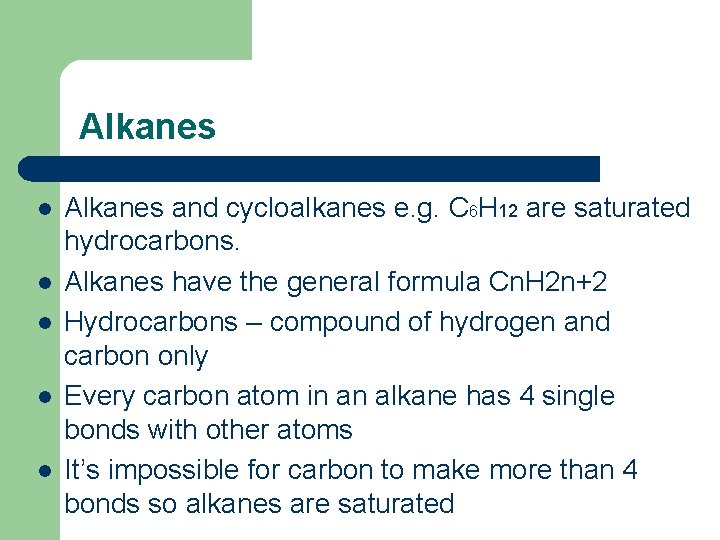
Alkanes l l l Alkanes and cycloalkanes e. g. C 6 H 12 are saturated hydrocarbons. Alkanes have the general formula Cn. H 2 n+2 Hydrocarbons – compound of hydrogen and carbon only Every carbon atom in an alkane has 4 single bonds with other atoms It’s impossible for carbon to make more than 4 bonds so alkanes are saturated
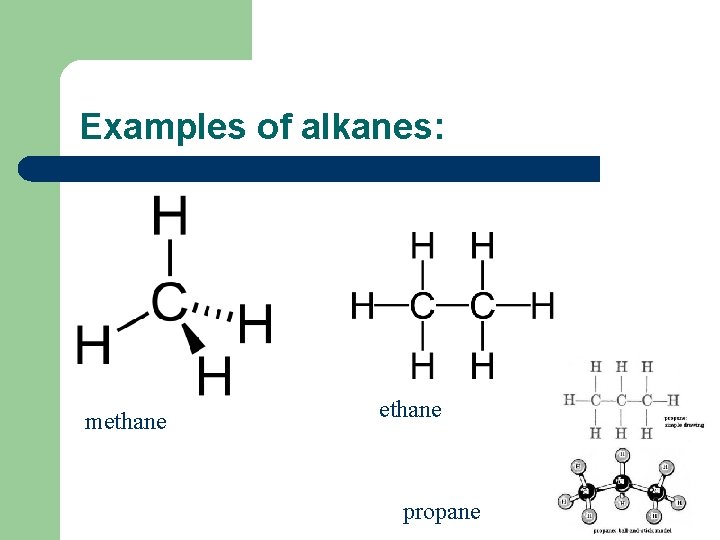
Examples of alkanes: methane propane
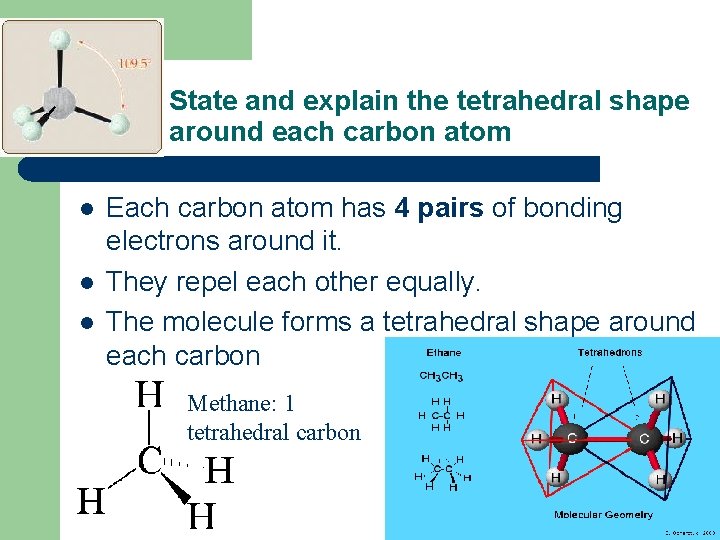
State and explain the tetrahedral shape around each carbon atom l l l Each carbon atom has 4 pairs of bonding electrons around it. They repel each other equally. The molecule forms a tetrahedral shape around each carbon Methane: 1 tetrahedral carbon
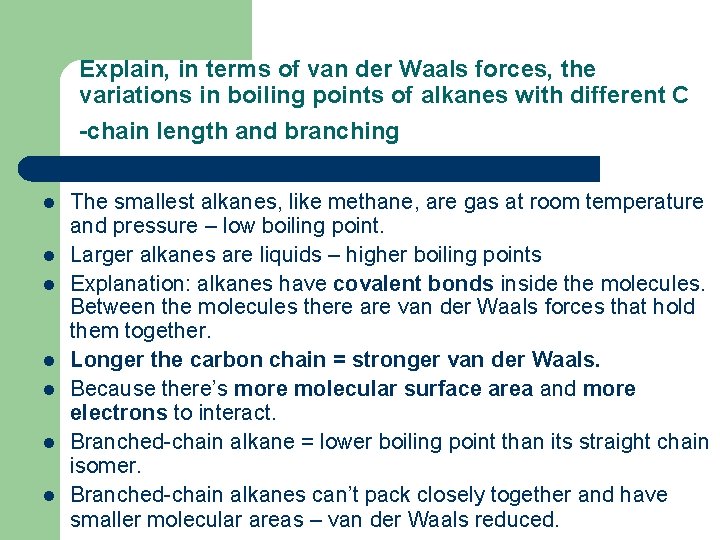
Explain, in terms of van der Waals forces, the variations in boiling points of alkanes with different C -chain length and branching l l l l The smallest alkanes, like methane, are gas at room temperature and pressure – low boiling point. Larger alkanes are liquids – higher boiling points Explanation: alkanes have covalent bonds inside the molecules. Between the molecules there are van der Waals forces that hold them together. Longer the carbon chain = stronger van der Waals. Because there’s more molecular surface area and more electrons to interact. Branched-chain alkane = lower boiling point than its straight chain isomer. Branched-chain alkanes can’t pack closely together and have smaller molecular areas – van der Waals reduced.
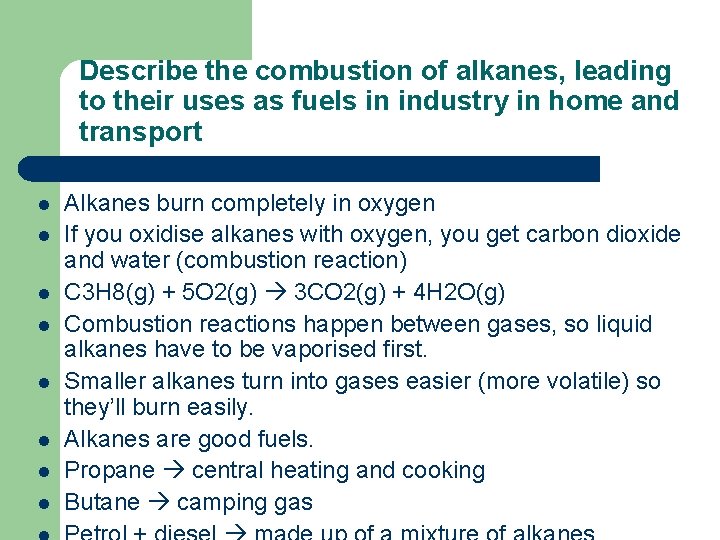
Describe the combustion of alkanes, leading to their uses as fuels in industry in home and transport l l l l Alkanes burn completely in oxygen If you oxidise alkanes with oxygen, you get carbon dioxide and water (combustion reaction) C 3 H 8(g) + 5 O 2(g) 3 CO 2(g) + 4 H 2 O(g) Combustion reactions happen between gases, so liquid alkanes have to be vaporised first. Smaller alkanes turn into gases easier (more volatile) so they’ll burn easily. Alkanes are good fuels. Propane central heating and cooking Butane camping gas
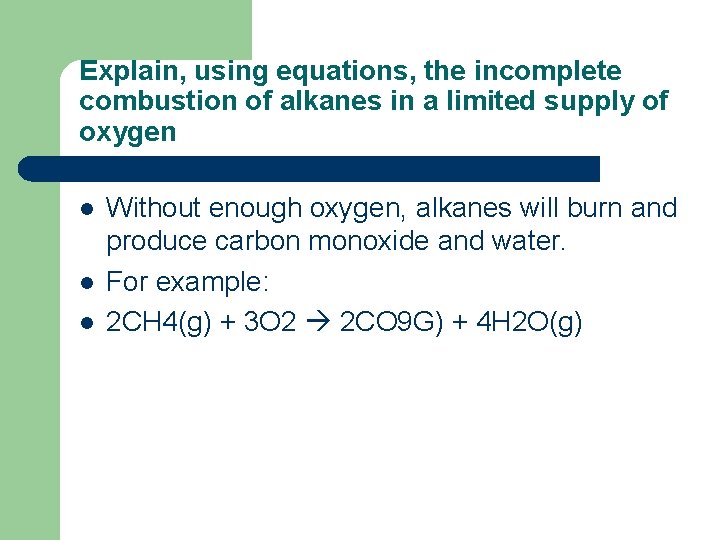
Explain, using equations, the incomplete combustion of alkanes in a limited supply of oxygen l l l Without enough oxygen, alkanes will burn and produce carbon monoxide and water. For example: 2 CH 4(g) + 3 O 2 2 CO 9 G) + 4 H 2 O(g)
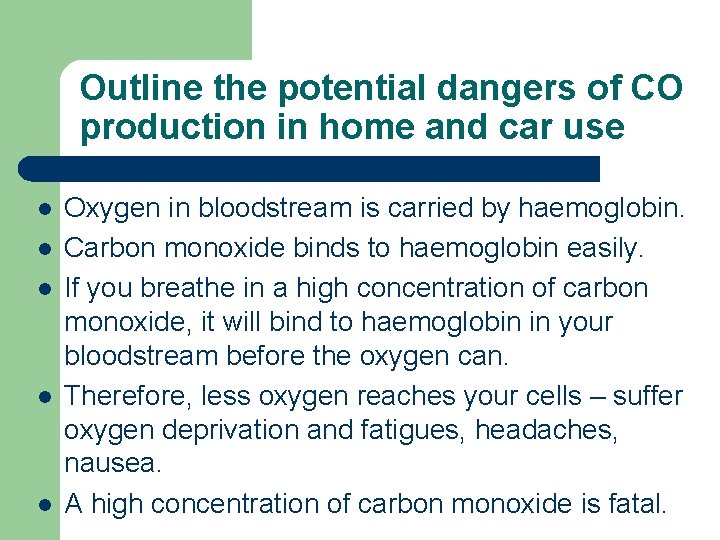
Outline the potential dangers of CO production in home and car use l l l Oxygen in bloodstream is carried by haemoglobin. Carbon monoxide binds to haemoglobin easily. If you breathe in a high concentration of carbon monoxide, it will bind to haemoglobin in your bloodstream before the oxygen can. Therefore, less oxygen reaches your cells – suffer oxygen deprivation and fatigues, headaches, nausea. A high concentration of carbon monoxide is fatal.
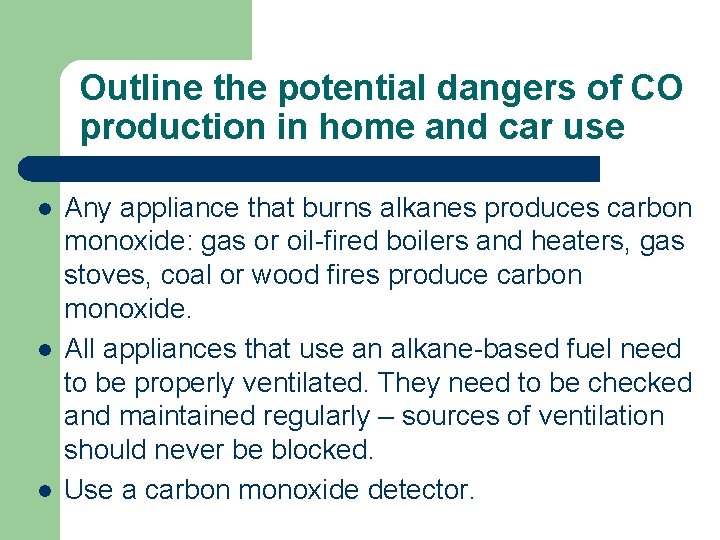
Outline the potential dangers of CO production in home and car use l l l Any appliance that burns alkanes produces carbon monoxide: gas or oil-fired boilers and heaters, gas stoves, coal or wood fires produce carbon monoxide. All appliances that use an alkane-based fuel need to be properly ventilated. They need to be checked and maintained regularly – sources of ventilation should never be blocked. Use a carbon monoxide detector.
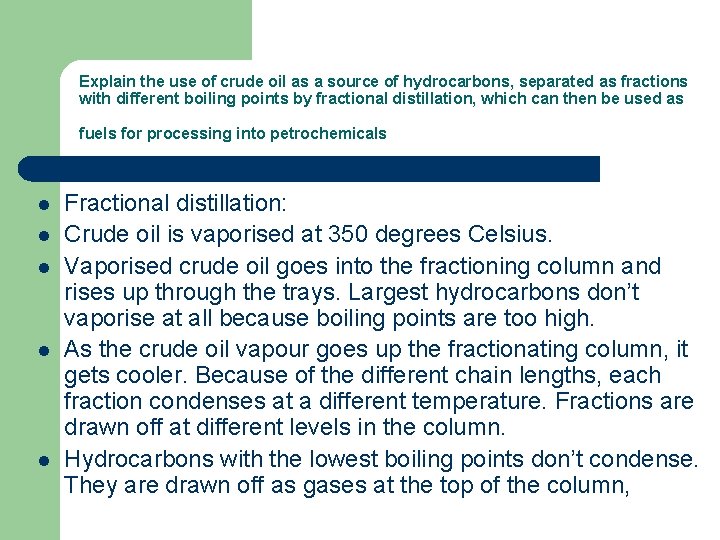
Explain the use of crude oil as a source of hydrocarbons, separated as fractions with different boiling points by fractional distillation, which can then be used as fuels for processing into petrochemicals l l l Fractional distillation: Crude oil is vaporised at 350 degrees Celsius. Vaporised crude oil goes into the fractioning column and rises up through the trays. Largest hydrocarbons don’t vaporise at all because boiling points are too high. As the crude oil vapour goes up the fractionating column, it gets cooler. Because of the different chain lengths, each fraction condenses at a different temperature. Fractions are drawn off at different levels in the column. Hydrocarbons with the lowest boiling points don’t condense. They are drawn off as gases at the top of the column,
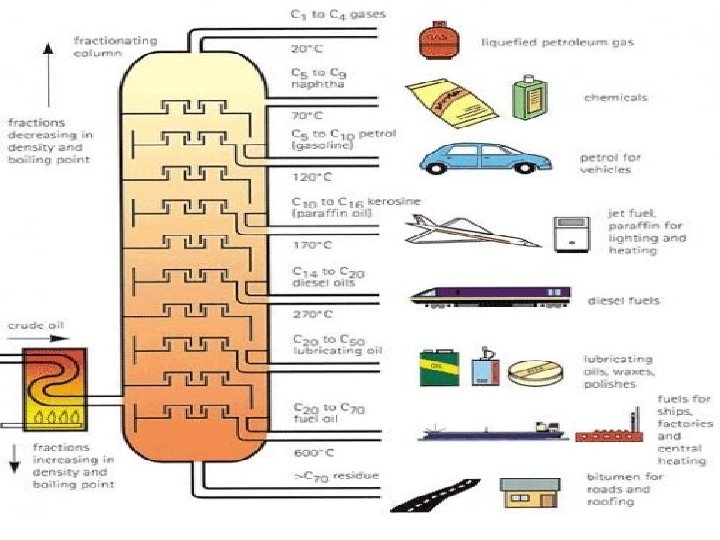
Fractional Distillation of crude oil
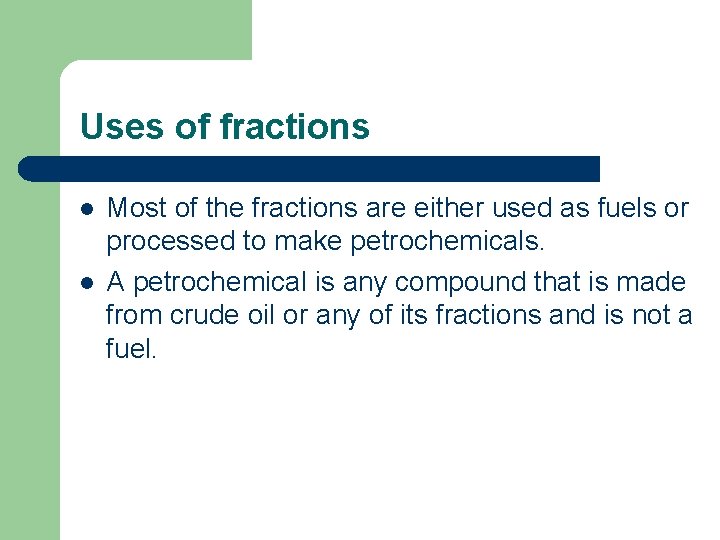
Uses of fractions l l Most of the fractions are either used as fuels or processed to make petrochemicals. A petrochemical is any compound that is made from crude oil or any of its fractions and is not a fuel.
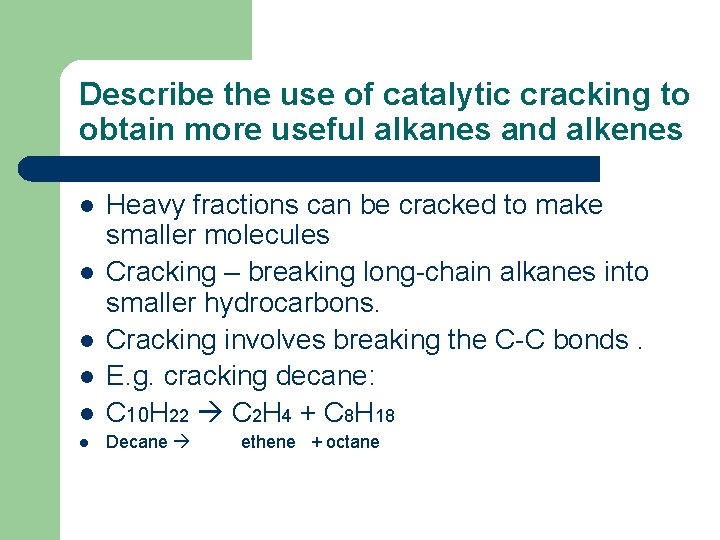
Describe the use of catalytic cracking to obtain more useful alkanes and alkenes l Heavy fractions can be cracked to make smaller molecules Cracking – breaking long-chain alkanes into smaller hydrocarbons. Cracking involves breaking the C-C bonds. E. g. cracking decane: C 10 H 22 C 2 H 4 + C 8 H 18 l Decane l l ethene + octane
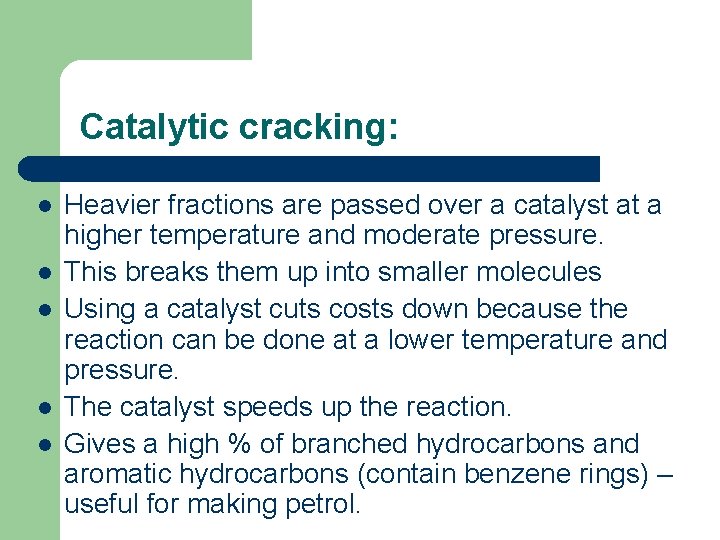
Catalytic cracking: l l l Heavier fractions are passed over a catalyst at a higher temperature and moderate pressure. This breaks them up into smaller molecules Using a catalyst cuts costs down because the reaction can be done at a lower temperature and pressure. The catalyst speeds up the reaction. Gives a high % of branched hydrocarbons and aromatic hydrocarbons (contain benzene rings) – useful for making petrol.
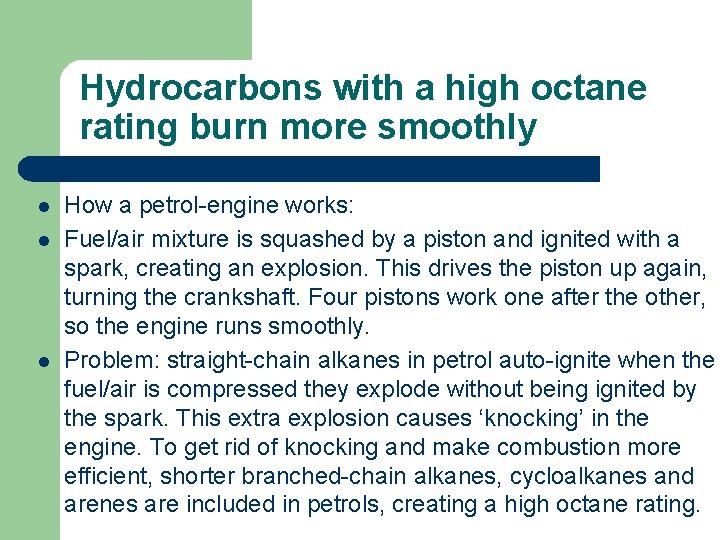
Hydrocarbons with a high octane rating burn more smoothly l l l How a petrol-engine works: Fuel/air mixture is squashed by a piston and ignited with a spark, creating an explosion. This drives the piston up again, turning the crankshaft. Four pistons work one after the other, so the engine runs smoothly. Problem: straight-chain alkanes in petrol auto-ignite when the fuel/air is compressed they explode without being ignited by the spark. This extra explosion causes ‘knocking’ in the engine. To get rid of knocking and make combustion more efficient, shorter branched-chain alkanes, cycloalkanes and arenes are included in petrols, creating a high octane rating.
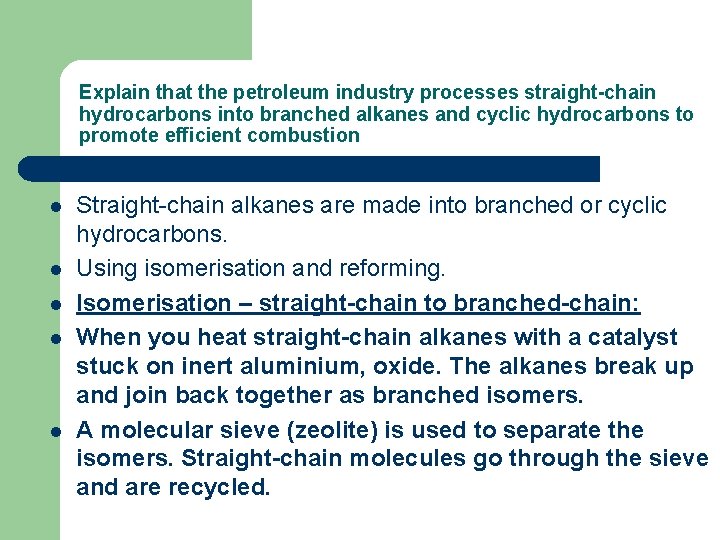
Explain that the petroleum industry processes straight-chain hydrocarbons into branched alkanes and cyclic hydrocarbons to promote efficient combustion l l l Straight-chain alkanes are made into branched or cyclic hydrocarbons. Using isomerisation and reforming. Isomerisation – straight-chain to branched-chain: When you heat straight-chain alkanes with a catalyst stuck on inert aluminium, oxide. The alkanes break up and join back together as branched isomers. A molecular sieve (zeolite) is used to separate the isomers. Straight-chain molecules go through the sieve and are recycled.
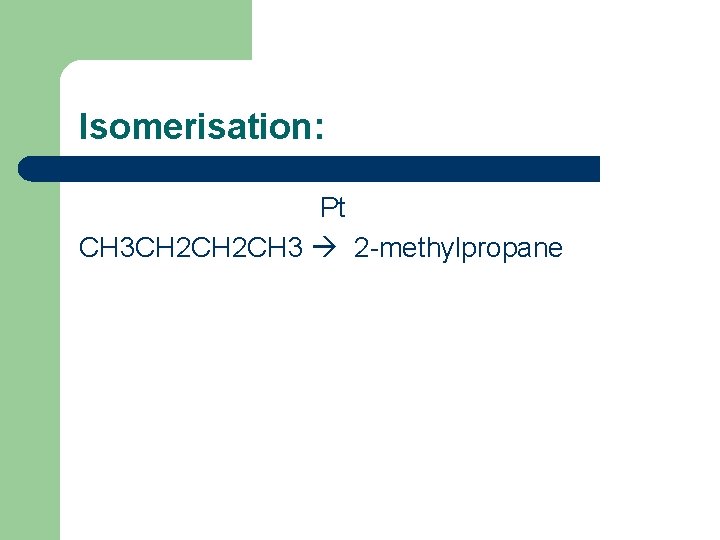
Isomerisation: Pt CH 3 CH 2 CH 3 2 -methylpropane
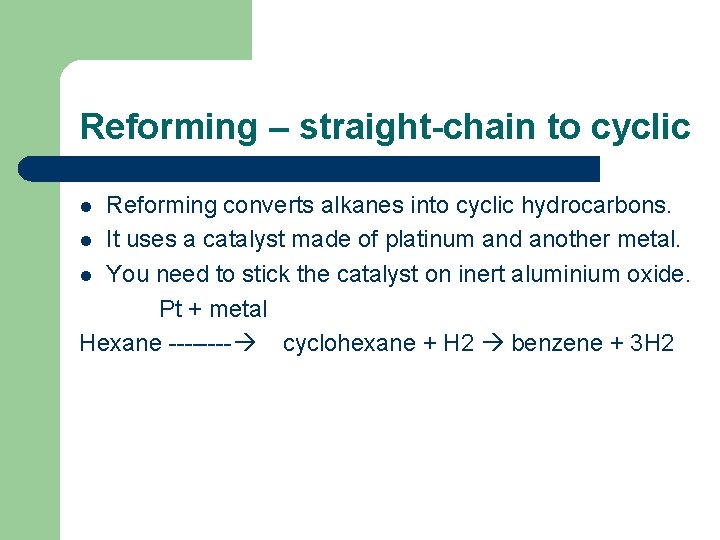
Reforming – straight-chain to cyclic Reforming converts alkanes into cyclic hydrocarbons. l It uses a catalyst made of platinum and another metal. l You need to stick the catalyst on inert aluminium oxide. Pt + metal Hexane ---- cyclohexane + H 2 benzene + 3 H 2 l
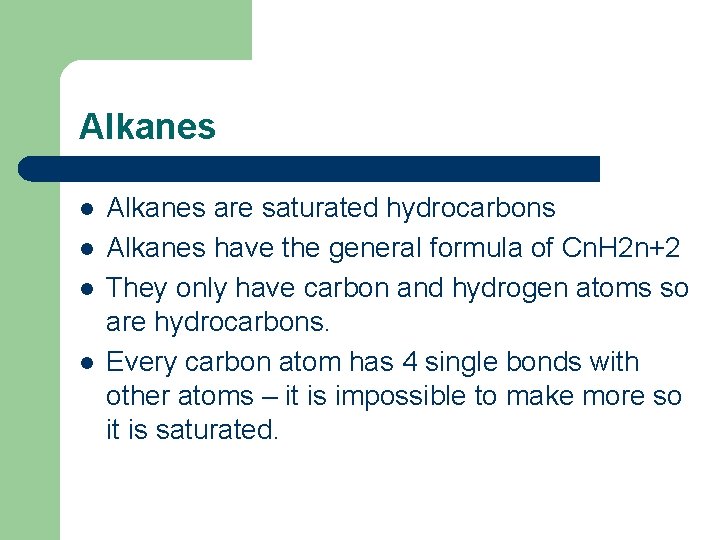
Alkanes l l Alkanes are saturated hydrocarbons Alkanes have the general formula of Cn. H 2 n+2 They only have carbon and hydrogen atoms so are hydrocarbons. Every carbon atom has 4 single bonds with other atoms – it is impossible to make more so it is saturated.
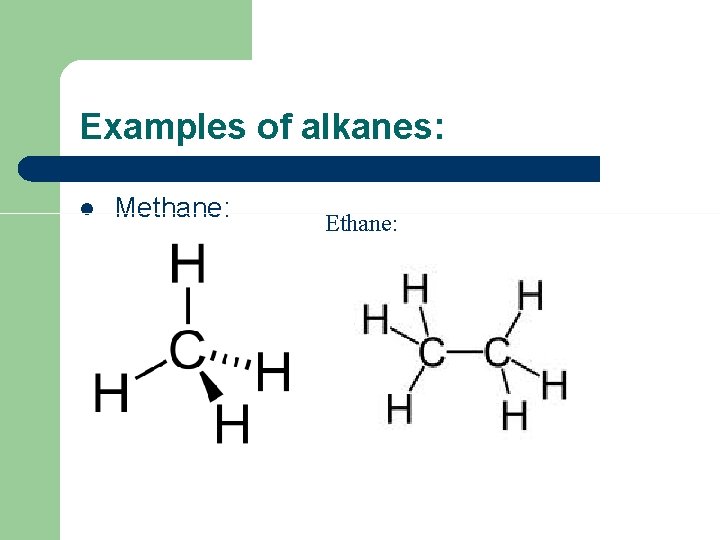
Examples of alkanes: l Methane: Ethane:
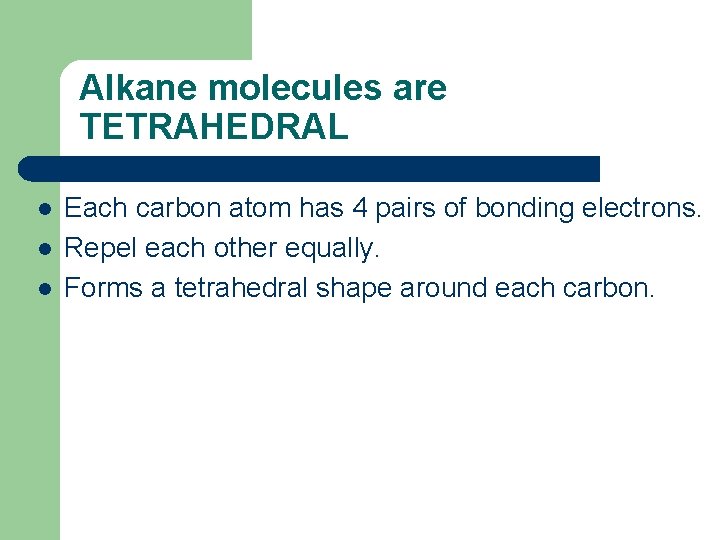
Alkane molecules are TETRAHEDRAL l l l Each carbon atom has 4 pairs of bonding electrons. Repel each other equally. Forms a tetrahedral shape around each carbon.
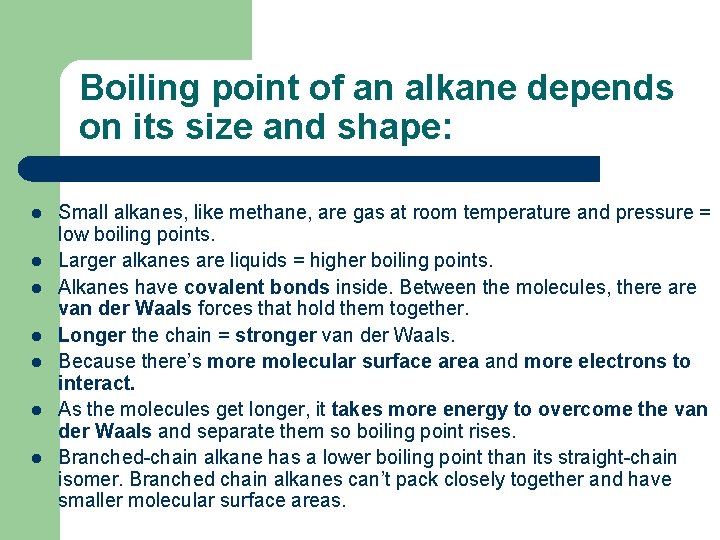
Boiling point of an alkane depends on its size and shape: l l l l Small alkanes, like methane, are gas at room temperature and pressure = low boiling points. Larger alkanes are liquids = higher boiling points. Alkanes have covalent bonds inside. Between the molecules, there are van der Waals forces that hold them together. Longer the chain = stronger van der Waals. Because there’s more molecular surface area and more electrons to interact. As the molecules get longer, it takes more energy to overcome the van der Waals and separate them so boiling point rises. Branched-chain alkane has a lower boiling point than its straight-chain isomer. Branched chain alkanes can’t pack closely together and have smaller molecular surface areas.
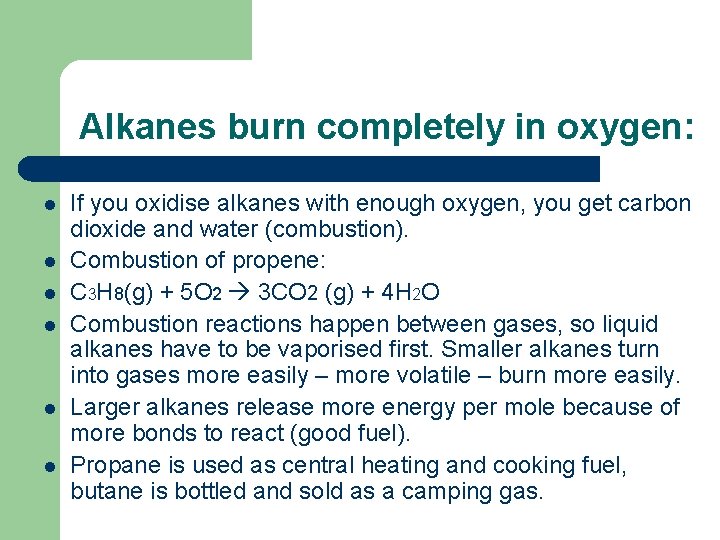
Alkanes burn completely in oxygen: l l l If you oxidise alkanes with enough oxygen, you get carbon dioxide and water (combustion). Combustion of propene: C 3 H 8(g) + 5 O 2 3 CO 2 (g) + 4 H 2 O Combustion reactions happen between gases, so liquid alkanes have to be vaporised first. Smaller alkanes turn into gases more easily – more volatile – burn more easily. Larger alkanes release more energy per mole because of more bonds to react (good fuel). Propane is used as central heating and cooking fuel, butane is bottled and sold as a camping gas.
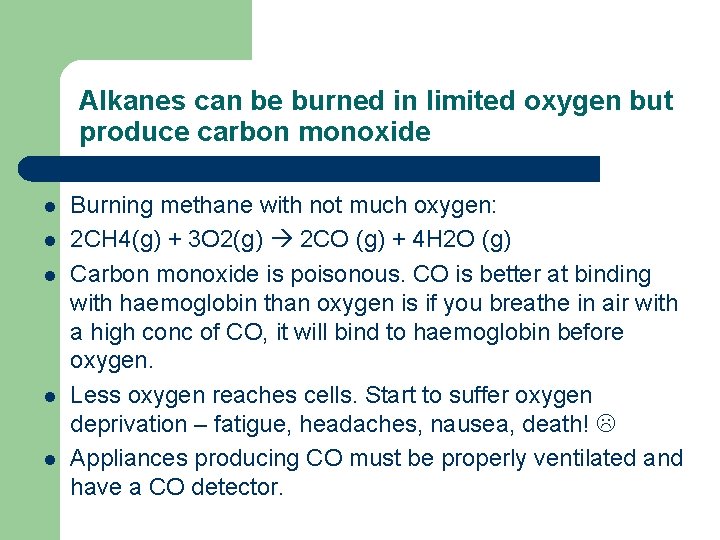
Alkanes can be burned in limited oxygen but produce carbon monoxide l l l Burning methane with not much oxygen: 2 CH 4(g) + 3 O 2(g) 2 CO (g) + 4 H 2 O (g) Carbon monoxide is poisonous. CO is better at binding with haemoglobin than oxygen is if you breathe in air with a high conc of CO, it will bind to haemoglobin before oxygen. Less oxygen reaches cells. Start to suffer oxygen deprivation – fatigue, headaches, nausea, death! Appliances producing CO must be properly ventilated and have a CO detector.
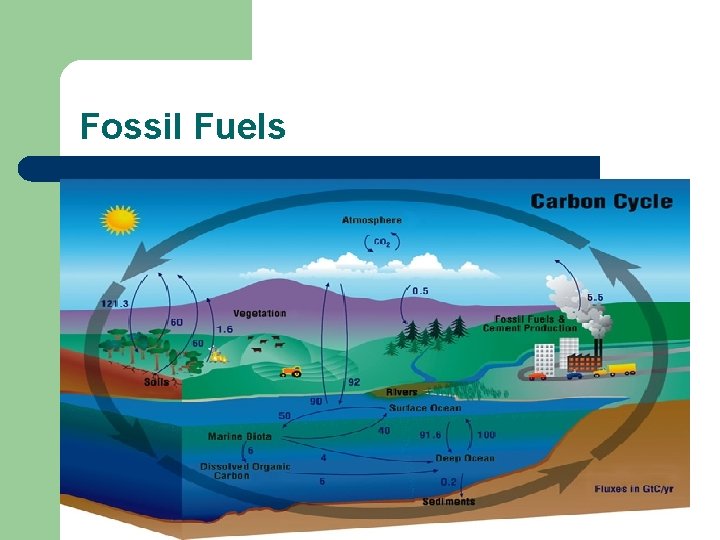
Fossil Fuels
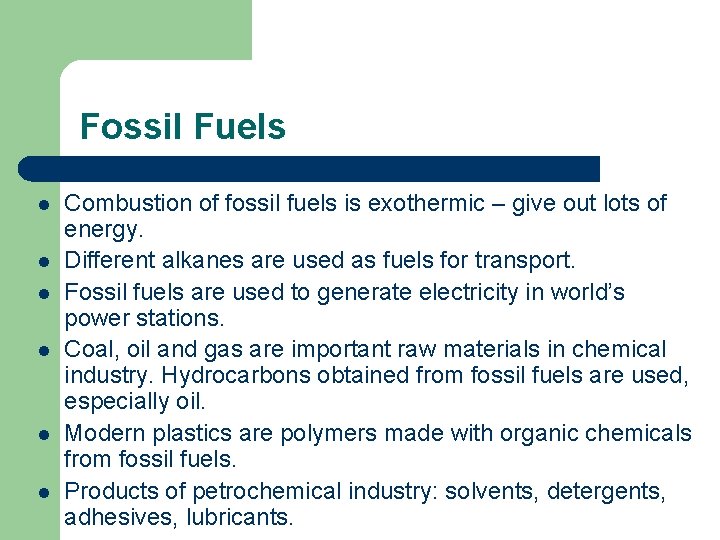
Fossil Fuels l l l Combustion of fossil fuels is exothermic – give out lots of energy. Different alkanes are used as fuels for transport. Fossil fuels are used to generate electricity in world’s power stations. Coal, oil and gas are important raw materials in chemical industry. Hydrocarbons obtained from fossil fuels are used, especially oil. Modern plastics are polymers made with organic chemicals from fossil fuels. Products of petrochemical industry: solvents, detergents, adhesives, lubricants.
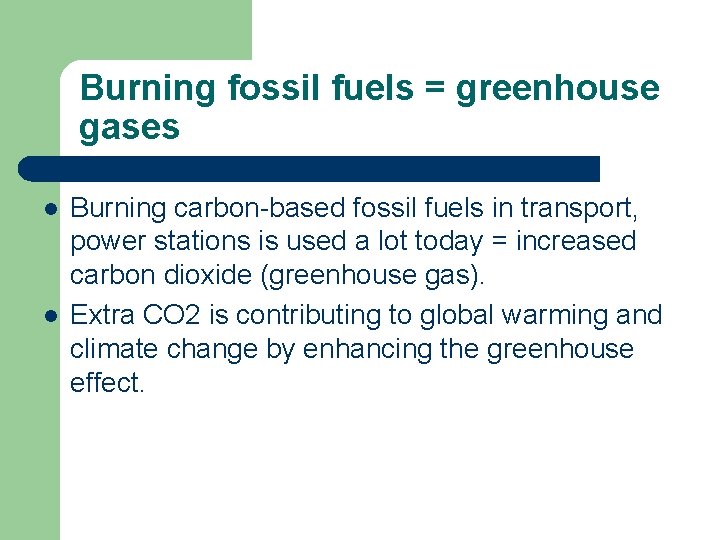
Burning fossil fuels = greenhouse gases l l Burning carbon-based fossil fuels in transport, power stations is used a lot today = increased carbon dioxide (greenhouse gas). Extra CO 2 is contributing to global warming and climate change by enhancing the greenhouse effect.
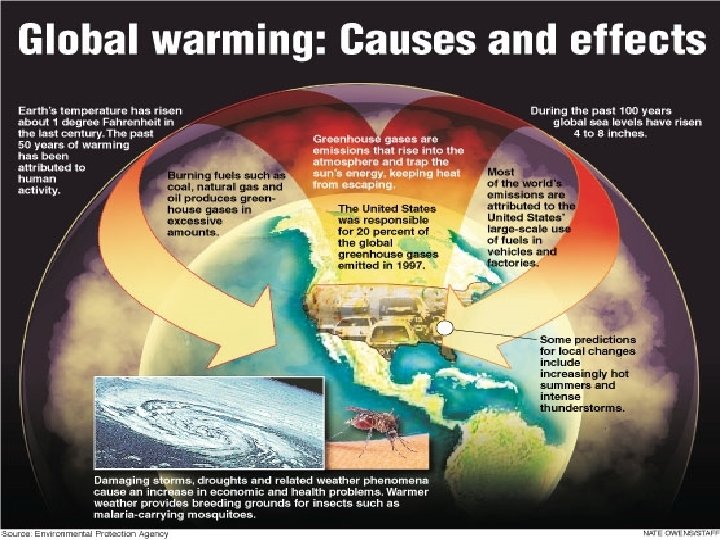
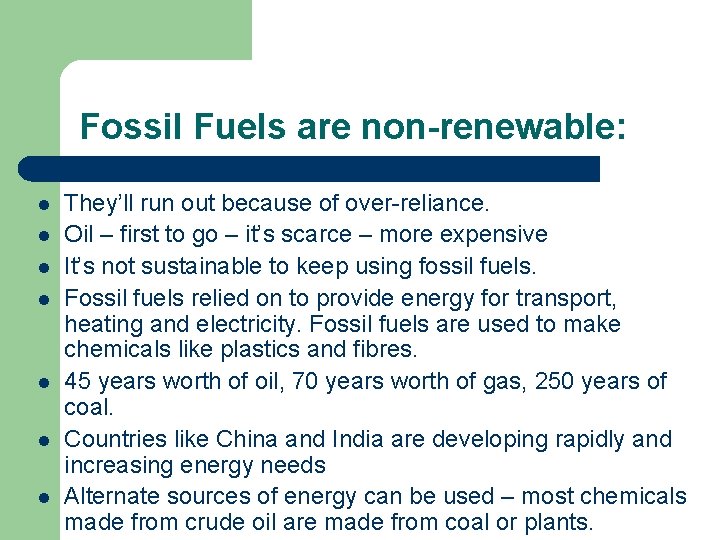
Fossil Fuels are non-renewable: l l l l They’ll run out because of over-reliance. Oil – first to go – it’s scarce – more expensive It’s not sustainable to keep using fossil fuels. Fossil fuels relied on to provide energy for transport, heating and electricity. Fossil fuels are used to make chemicals like plastics and fibres. 45 years worth of oil, 70 years worth of gas, 250 years of coal. Countries like China and India are developing rapidly and increasing energy needs Alternate sources of energy can be used – most chemicals made from crude oil are made from coal or plants.
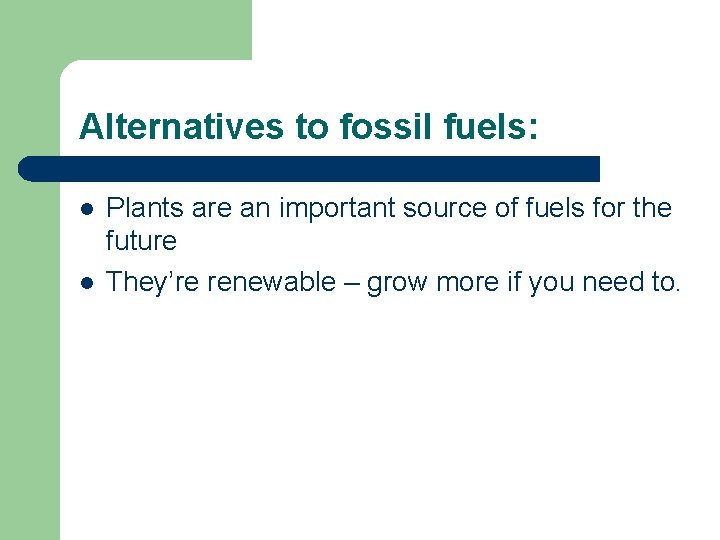
Alternatives to fossil fuels: l l Plants are an important source of fuels for the future They’re renewable – grow more if you need to.
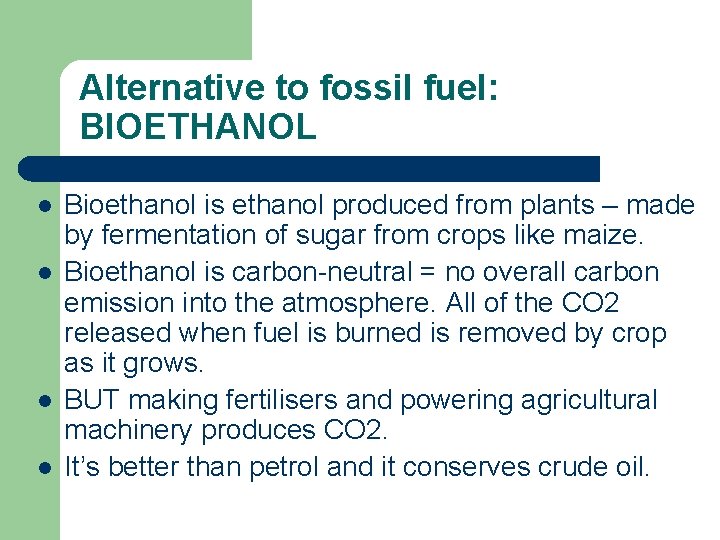
Alternative to fossil fuel: BIOETHANOL l l Bioethanol is ethanol produced from plants – made by fermentation of sugar from crops like maize. Bioethanol is carbon-neutral = no overall carbon emission into the atmosphere. All of the CO 2 released when fuel is burned is removed by crop as it grows. BUT making fertilisers and powering agricultural machinery produces CO 2. It’s better than petrol and it conserves crude oil.
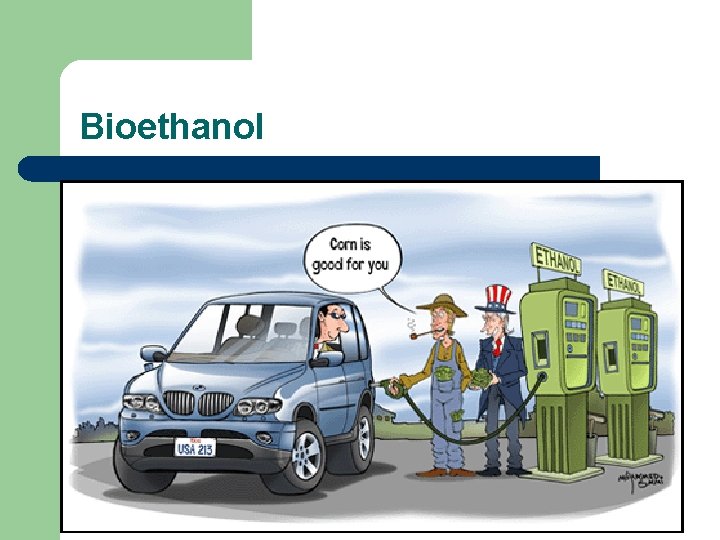
Bioethanol
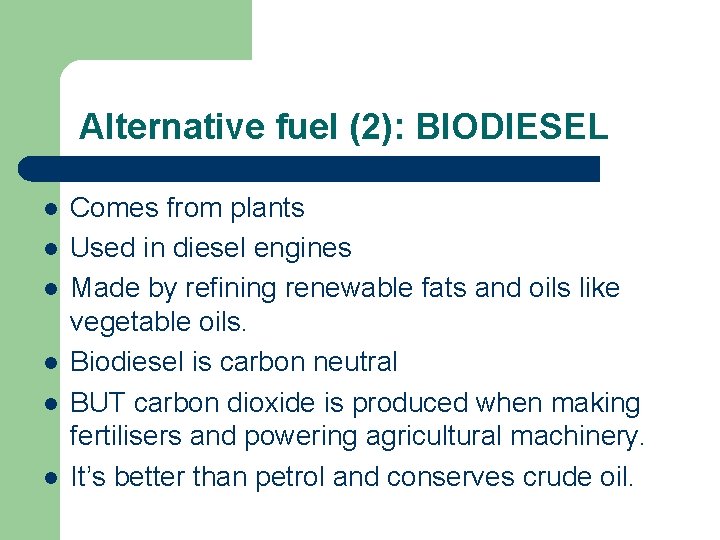
Alternative fuel (2): BIODIESEL l l l Comes from plants Used in diesel engines Made by refining renewable fats and oils like vegetable oils. Biodiesel is carbon neutral BUT carbon dioxide is produced when making fertilisers and powering agricultural machinery. It’s better than petrol and conserves crude oil.
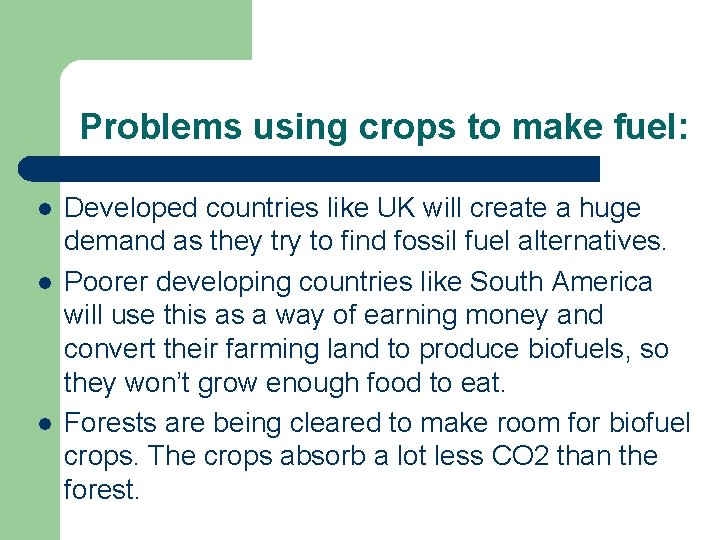
Problems using crops to make fuel: l l l Developed countries like UK will create a huge demand as they try to find fossil fuel alternatives. Poorer developing countries like South America will use this as a way of earning money and convert their farming land to produce biofuels, so they won’t grow enough food to eat. Forests are being cleared to make room for biofuel crops. The crops absorb a lot less CO 2 than the forest.
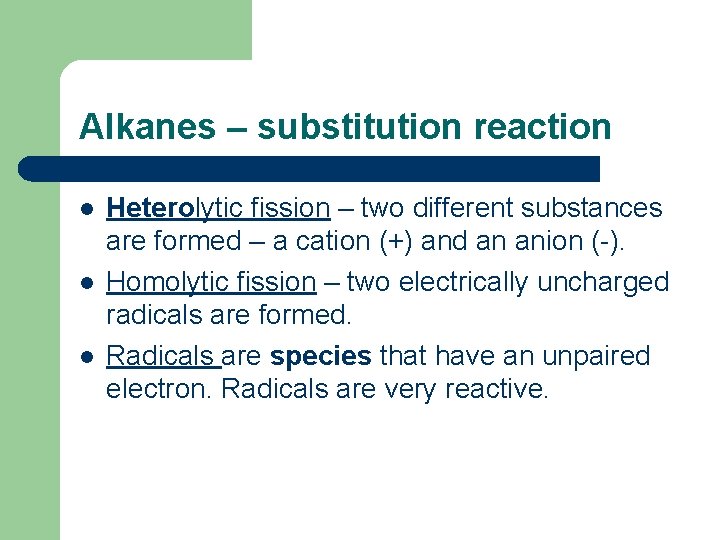
Alkanes – substitution reaction l l l Heterolytic fission – two different substances are formed – a cation (+) and an anion (-). Homolytic fission – two electrically uncharged radicals are formed. Radicals are species that have an unpaired electron. Radicals are very reactive.
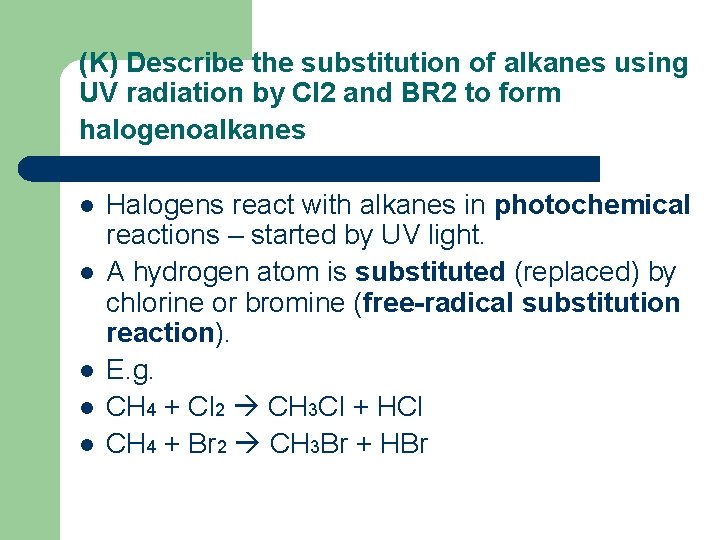
(K) Describe the substitution of alkanes using UV radiation by Cl 2 and BR 2 to form halogenoalkanes l l l Halogens react with alkanes in photochemical reactions – started by UV light. A hydrogen atom is substituted (replaced) by chlorine or bromine (free-radical substitution reaction). E. g. CH 4 + Cl 2 CH 3 Cl + HCl CH 4 + Br 2 CH 3 Br + HBr
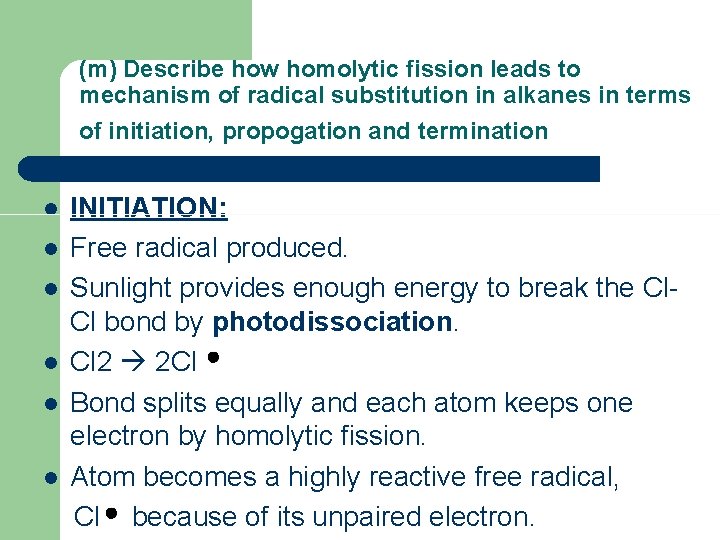
(m) Describe how homolytic fission leads to mechanism of radical substitution in alkanes in terms of initiation, propogation and termination l l l INITIATION: Free radical produced. Sunlight provides enough energy to break the Cl. Cl bond by photodissociation. Cl 2 2 Cl Bond splits equally and each atom keeps one electron by homolytic fission. Atom becomes a highly reactive free radical, Cl because of its unpaired electron.
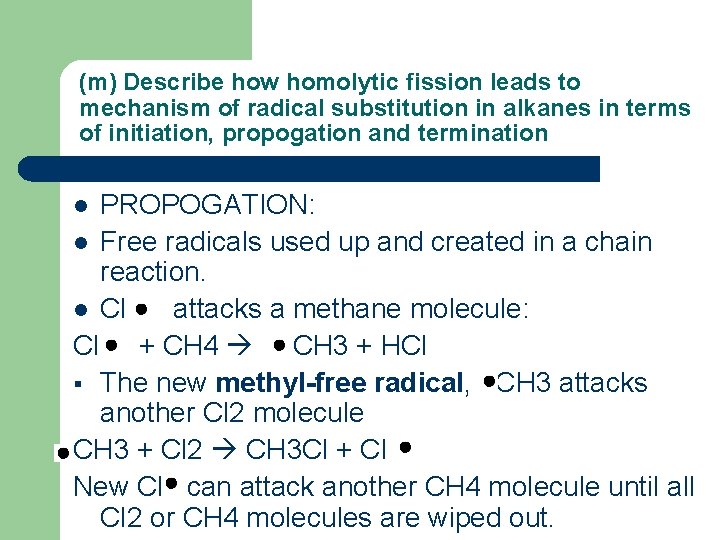
(m) Describe how homolytic fission leads to mechanism of radical substitution in alkanes in terms of initiation, propogation and termination PROPOGATION: l Free radicals used up and created in a chain reaction. l Cl attacks a methane molecule: Cl + CH 4 CH 3 + HCl § The new methyl-free radical, CH 3 attacks another Cl 2 molecule CH 3 + Cl 2 CH 3 Cl + Cl New Cl can attack another CH 4 molecule until all Cl 2 or CH 4 molecules are wiped out. l
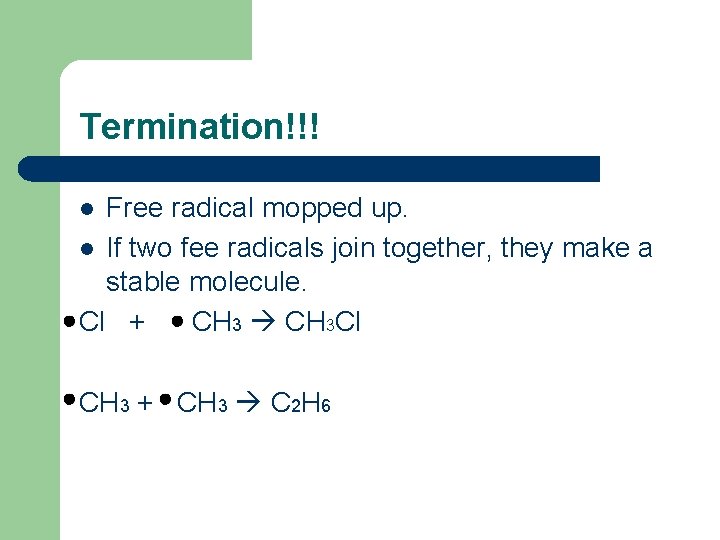
Termination!!! Free radical mopped up. l If two fee radicals join together, they make a stable molecule. Cl + CH 3 Cl l CH 3 + CH 3 C 2 H 6
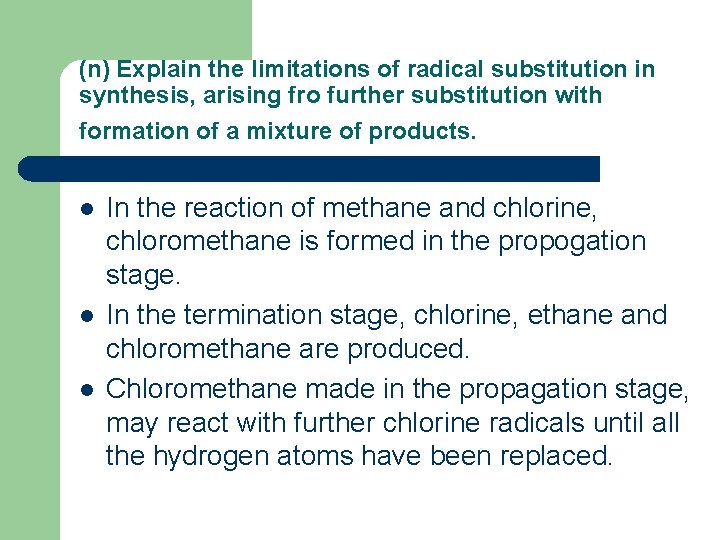
(n) Explain the limitations of radical substitution in synthesis, arising fro further substitution with formation of a mixture of products. l l l In the reaction of methane and chlorine, chloromethane is formed in the propogation stage. In the termination stage, chlorine, ethane and chloromethane are produced. Chloromethane made in the propagation stage, may react with further chlorine radicals until all the hydrogen atoms have been replaced.
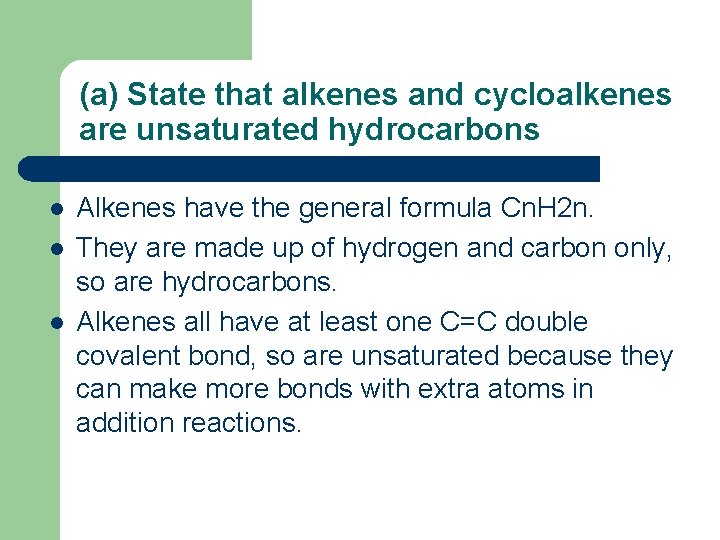
(a) State that alkenes and cycloalkenes are unsaturated hydrocarbons l l l Alkenes have the general formula Cn. H 2 n. They are made up of hydrogen and carbon only, so are hydrocarbons. Alkenes all have at least one C=C double covalent bond, so are unsaturated because they can make more bonds with extra atoms in addition reactions.
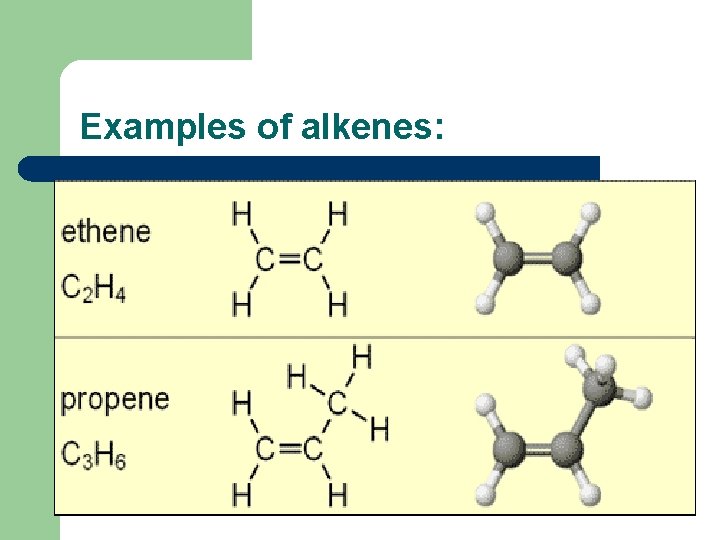
Examples of alkenes:
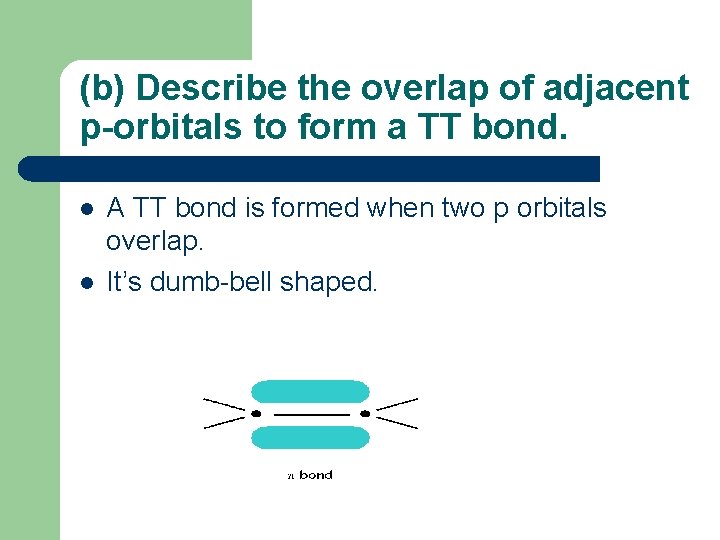
(b) Describe the overlap of adjacent p-orbitals to form a TT bond. l l A TT bond is formed when two p orbitals overlap. It’s dumb-bell shaped.
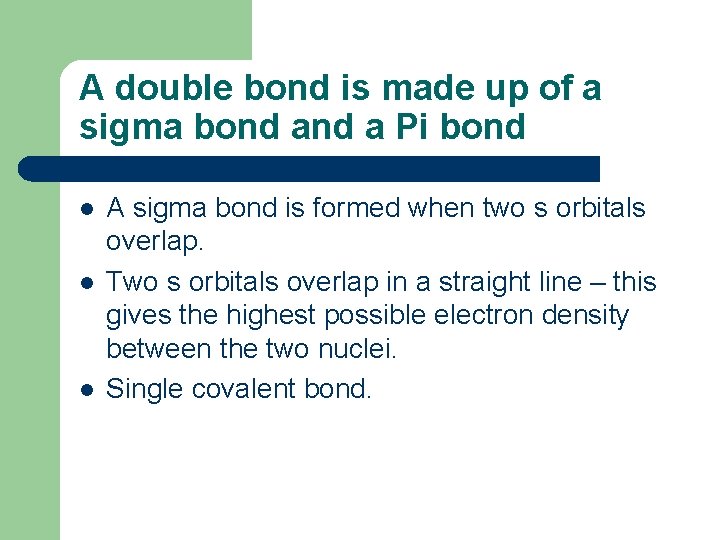
A double bond is made up of a sigma bond a Pi bond l l l A sigma bond is formed when two s orbitals overlap. Two s orbitals overlap in a straight line – this gives the highest possible electron density between the two nuclei. Single covalent bond.
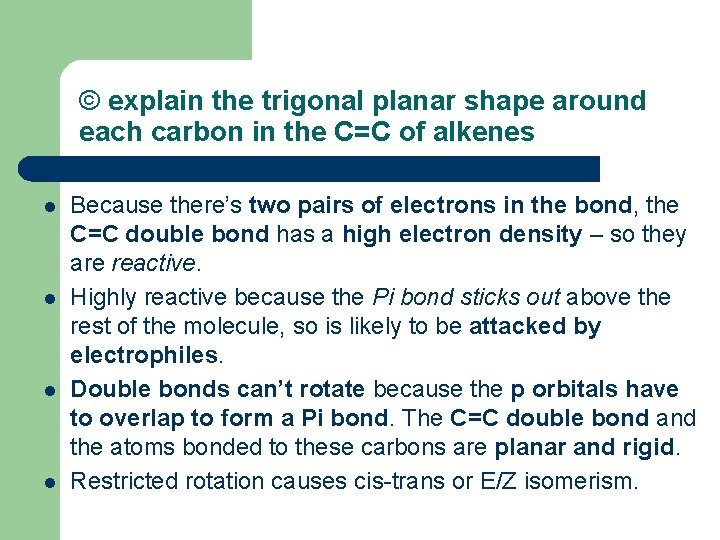
© explain the trigonal planar shape around each carbon in the C=C of alkenes l l Because there’s two pairs of electrons in the bond, the C=C double bond has a high electron density – so they are reactive. Highly reactive because the Pi bond sticks out above the rest of the molecule, so is likely to be attacked by electrophiles. Double bonds can’t rotate because the p orbitals have to overlap to form a Pi bond. The C=C double bond and the atoms bonded to these carbons are planar and rigid. Restricted rotation causes cis-trans or E/Z isomerism.
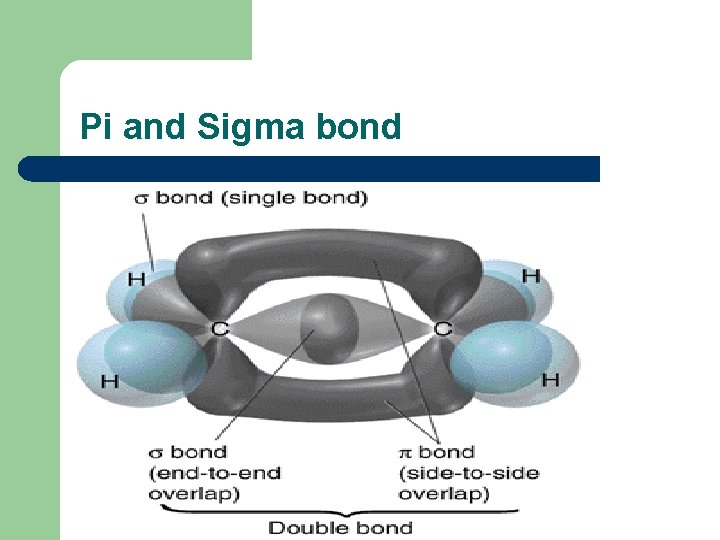
Pi and Sigma bond
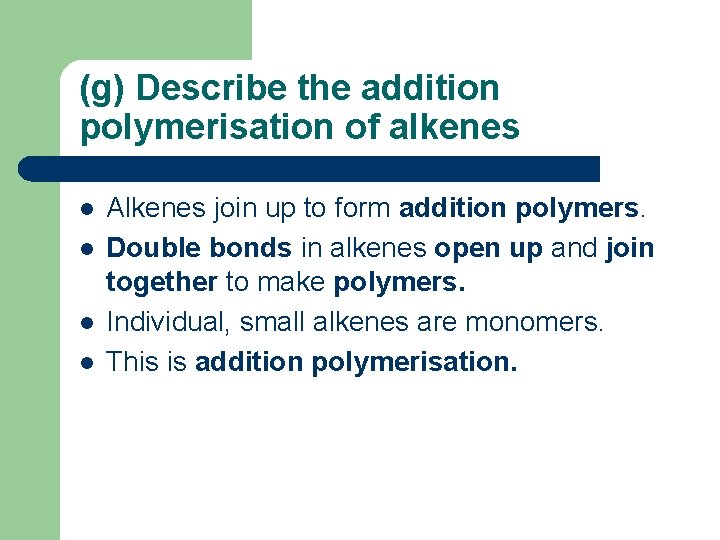
(g) Describe the addition polymerisation of alkenes l l Alkenes join up to form addition polymers. Double bonds in alkenes open up and join together to make polymers. Individual, small alkenes are monomers. This is addition polymerisation.
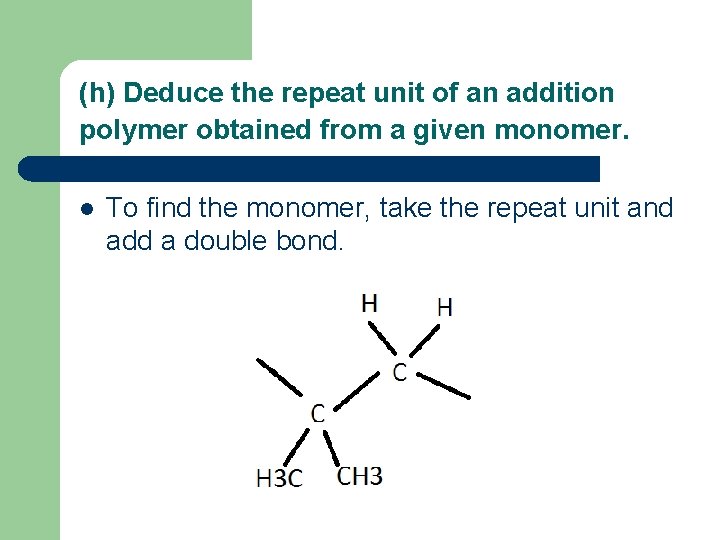
(h) Deduce the repeat unit of an addition polymer obtained from a given monomer. l To find the monomer, take the repeat unit and add a double bond.
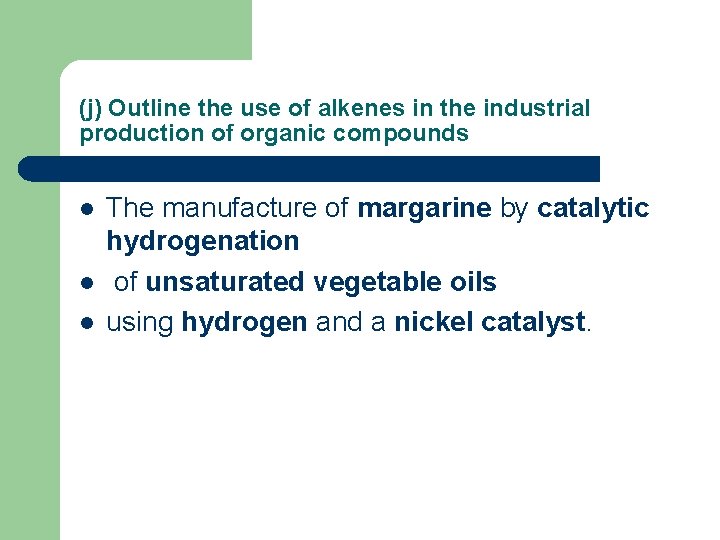
(j) Outline the use of alkenes in the industrial production of organic compounds l l l The manufacture of margarine by catalytic hydrogenation of unsaturated vegetable oils using hydrogen and a nickel catalyst.
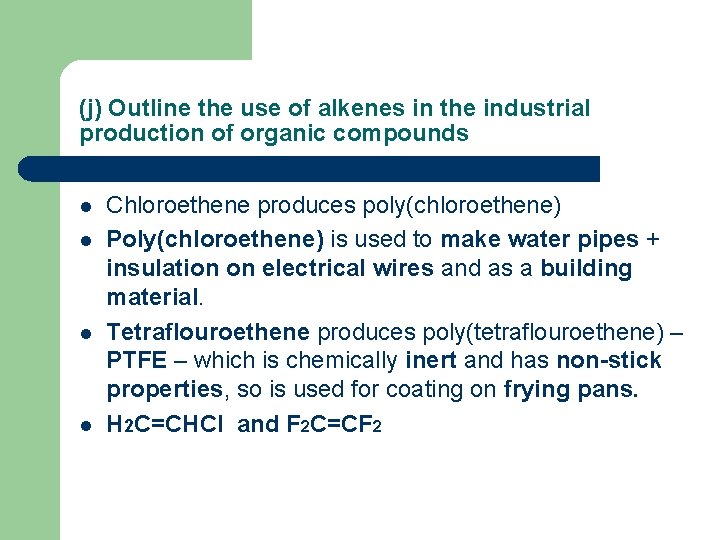
(j) Outline the use of alkenes in the industrial production of organic compounds l l Chloroethene produces poly(chloroethene) Poly(chloroethene) is used to make water pipes + insulation on electrical wires and as a building material. Tetraflouroethene produces poly(tetraflouroethene) – PTFE – which is chemically inert and has non-stick properties, so is used for coating on frying pans. H 2 C=CHCl and F 2 C=CF 2
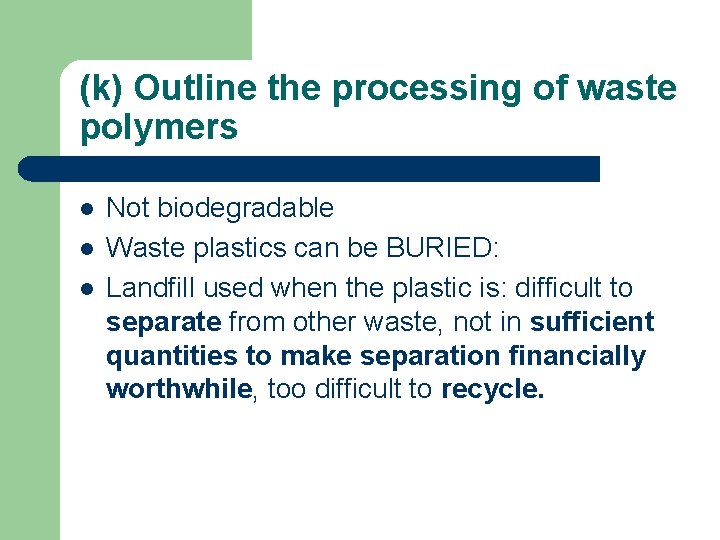
(k) Outline the processing of waste polymers l l l Not biodegradable Waste plastics can be BURIED: Landfill used when the plastic is: difficult to separate from other waste, not in sufficient quantities to make separation financially worthwhile, too difficult to recycle.
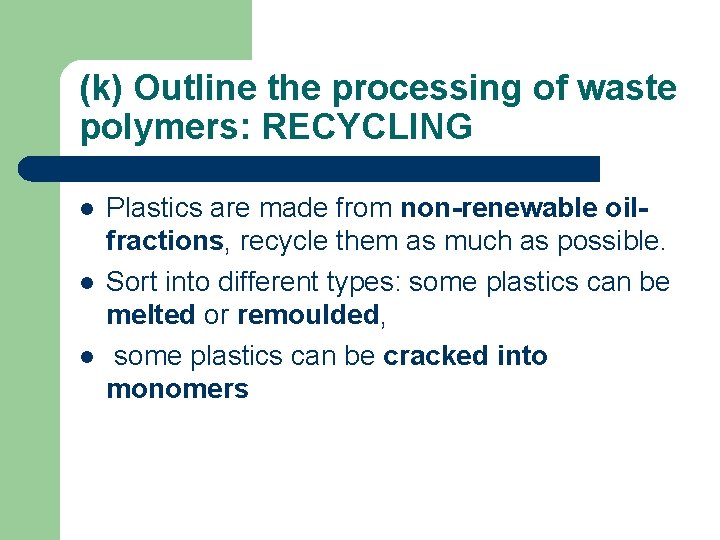
(k) Outline the processing of waste polymers: RECYCLING l l l Plastics are made from non-renewable oilfractions, recycle them as much as possible. Sort into different types: some plastics can be melted or remoulded, some plastics can be cracked into monomers
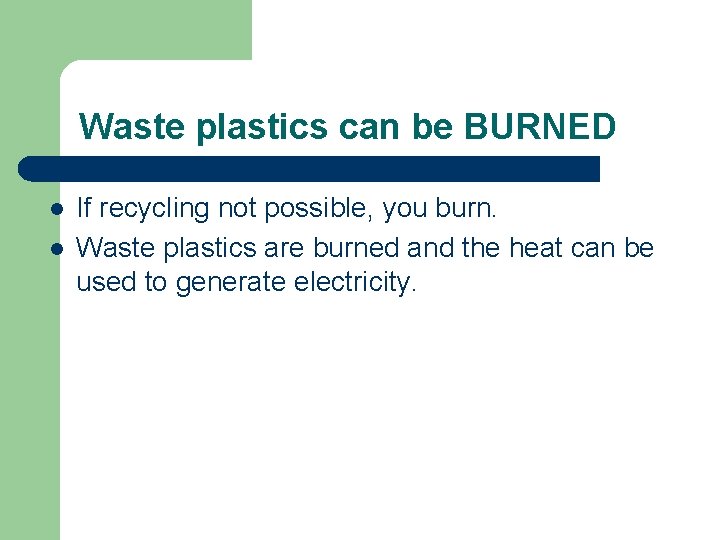
Waste plastics can be BURNED l l If recycling not possible, you burn. Waste plastics are burned and the heat can be used to generate electricity.
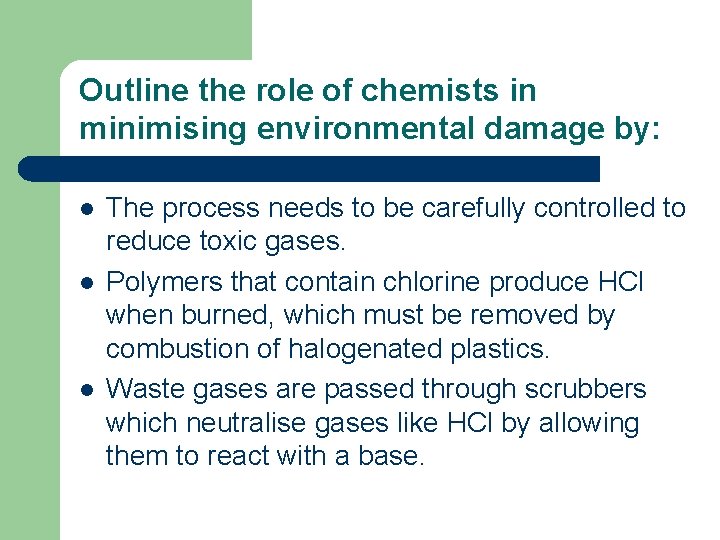
Outline the role of chemists in minimising environmental damage by: l l l The process needs to be carefully controlled to reduce toxic gases. Polymers that contain chlorine produce HCl when burned, which must be removed by combustion of halogenated plastics. Waste gases are passed through scrubbers which neutralise gases like HCl by allowing them to react with a base.
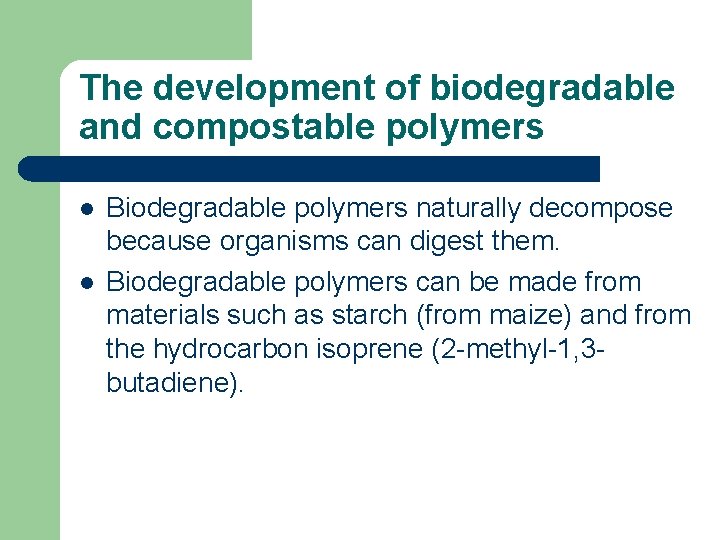
The development of biodegradable and compostable polymers l l Biodegradable polymers naturally decompose because organisms can digest them. Biodegradable polymers can be made from materials such as starch (from maize) and from the hydrocarbon isoprene (2 -methyl-1, 3 butadiene).
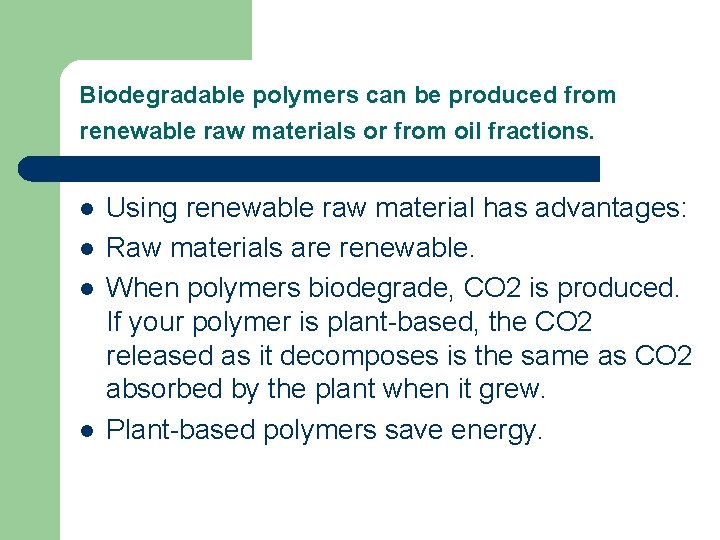
Biodegradable polymers can be produced from renewable raw materials or from oil fractions. l l Using renewable raw material has advantages: Raw materials are renewable. When polymers biodegrade, CO 2 is produced. If your polymer is plant-based, the CO 2 released as it decomposes is the same as CO 2 absorbed by the plant when it grew. Plant-based polymers save energy.
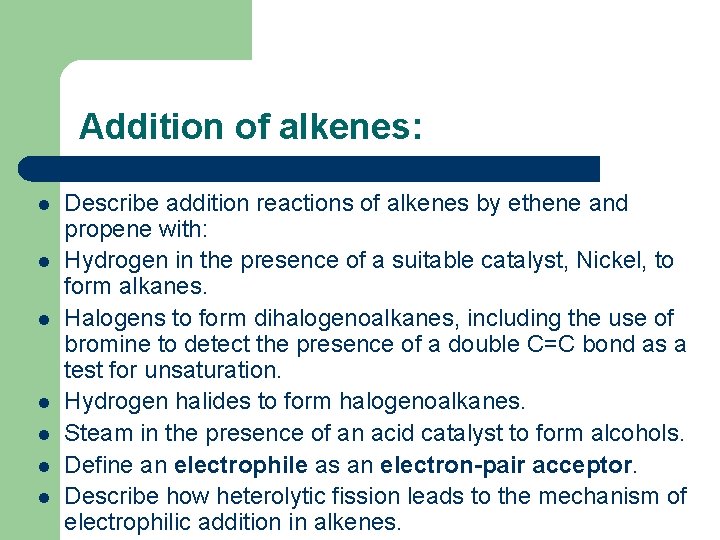
Addition of alkenes: l l l l Describe addition reactions of alkenes by ethene and propene with: Hydrogen in the presence of a suitable catalyst, Nickel, to form alkanes. Halogens to form dihalogenoalkanes, including the use of bromine to detect the presence of a double C=C bond as a test for unsaturation. Hydrogen halides to form halogenoalkanes. Steam in the presence of an acid catalyst to form alcohols. Define an electrophile as an electron-pair acceptor. Describe how heterolytic fission leads to the mechanism of electrophilic addition in alkenes.
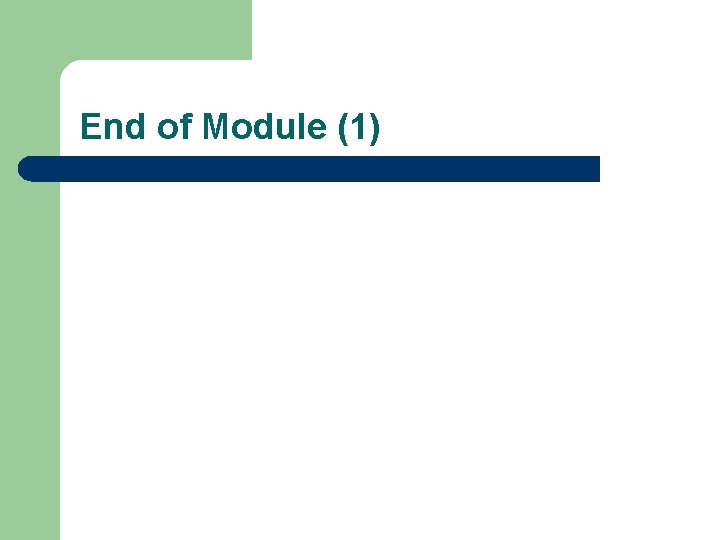
End of Module (1)