Chapter 2 6 General Types of Analog Filters
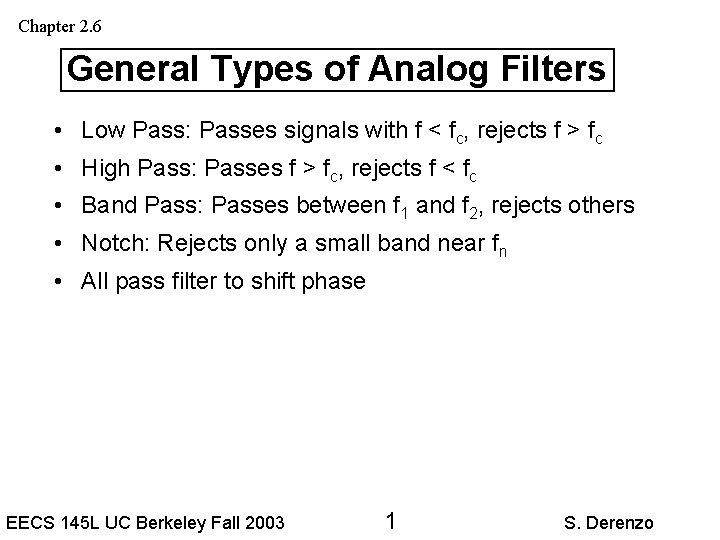
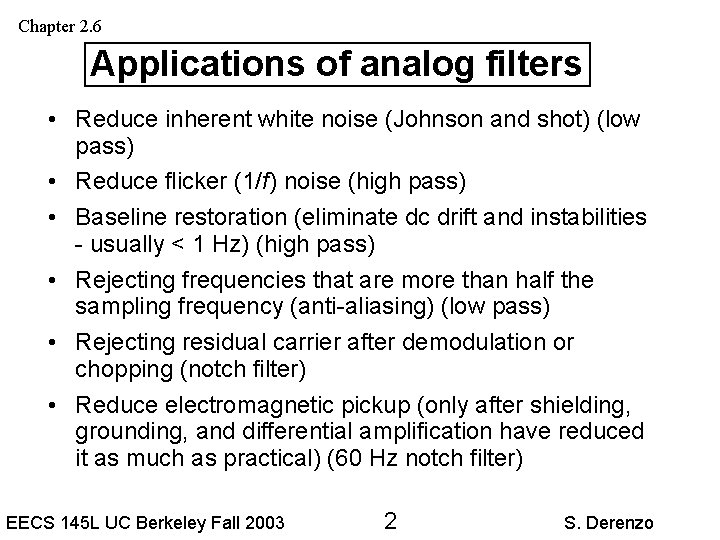
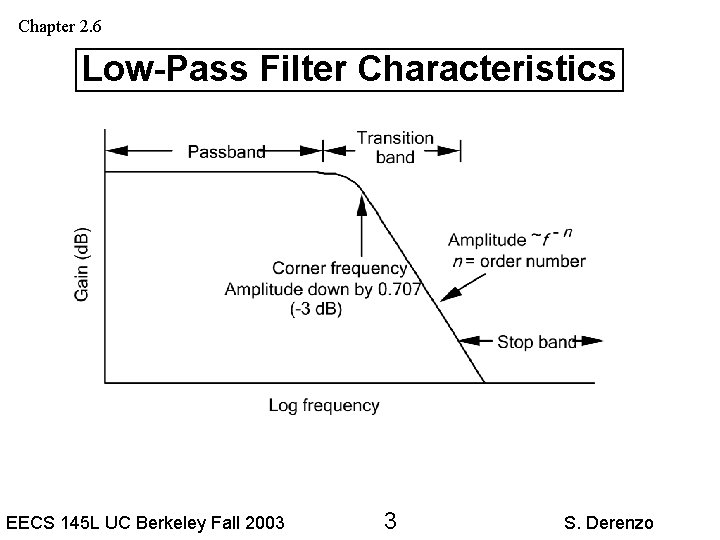
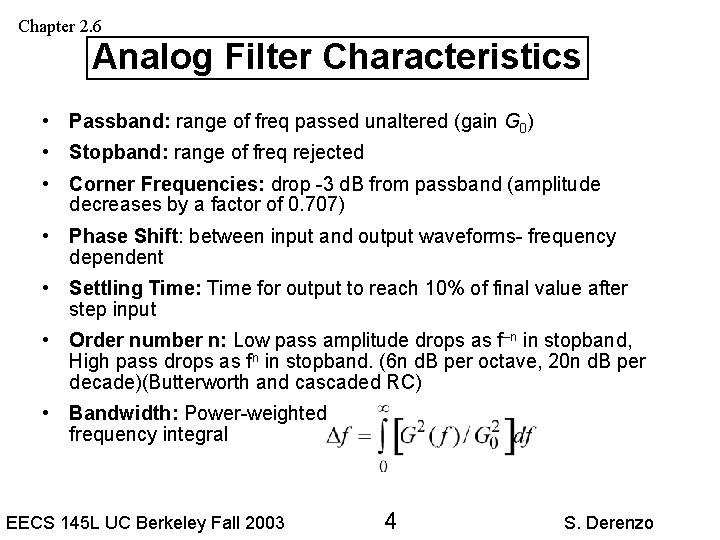
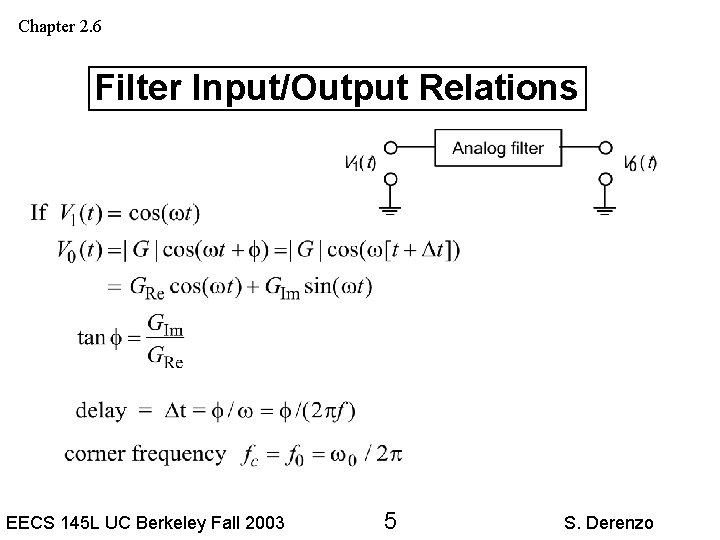
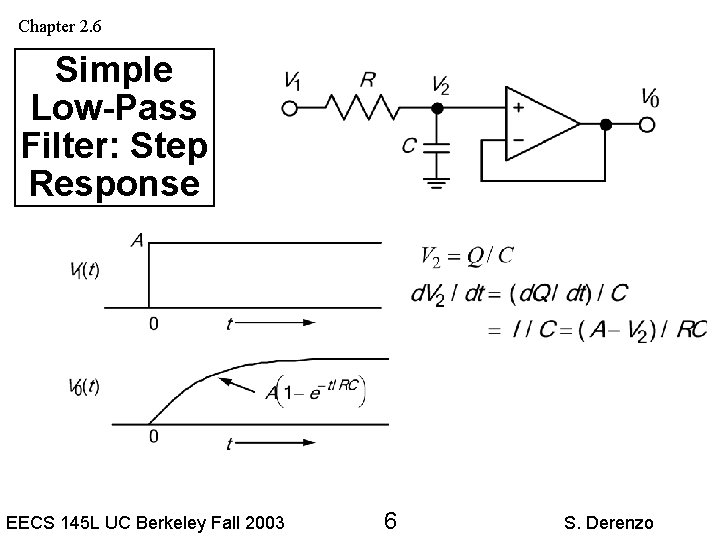
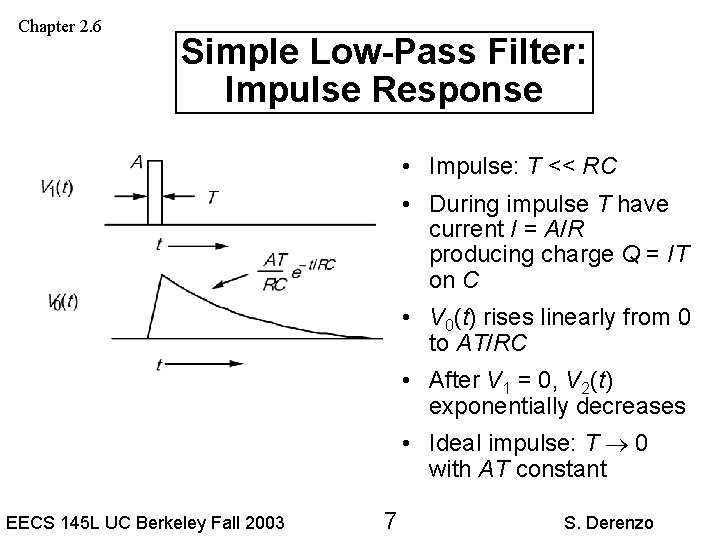
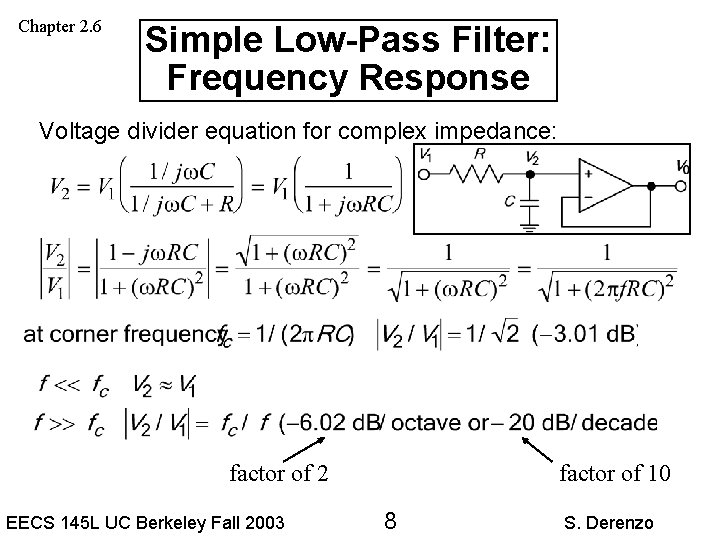
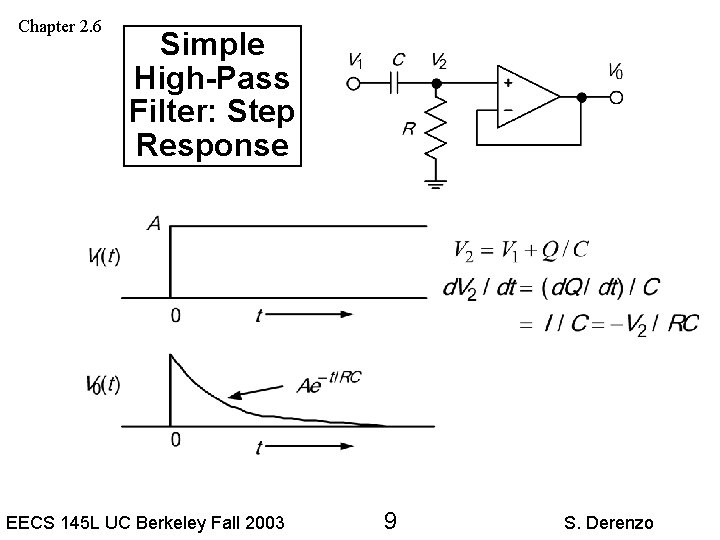
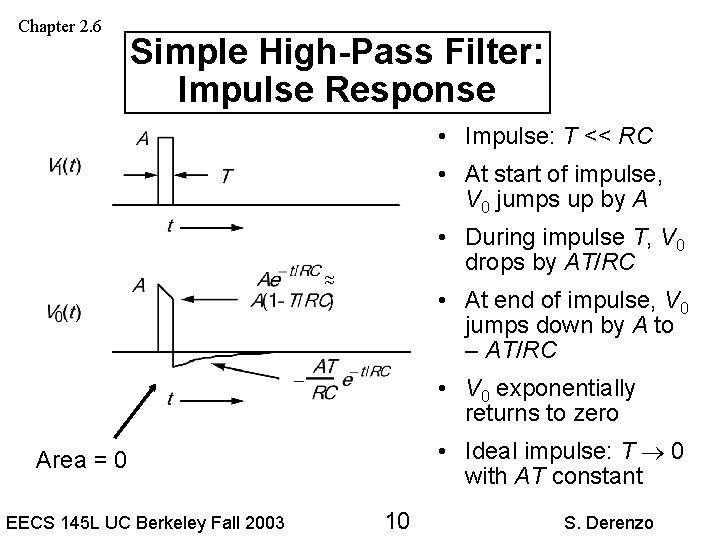
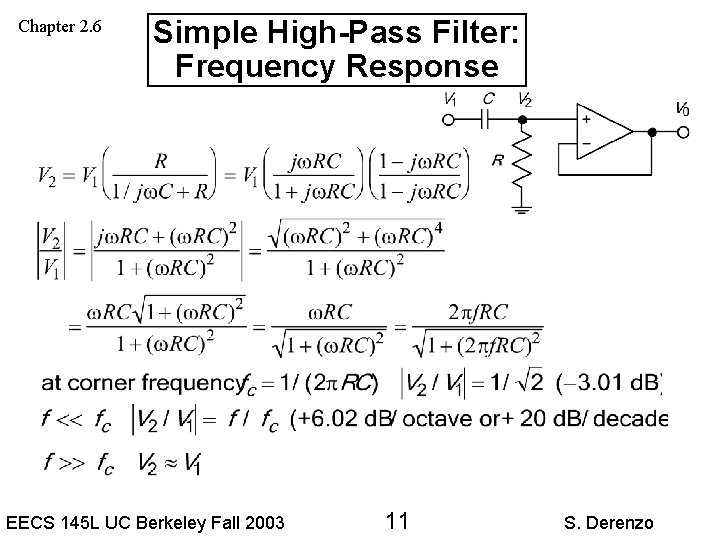
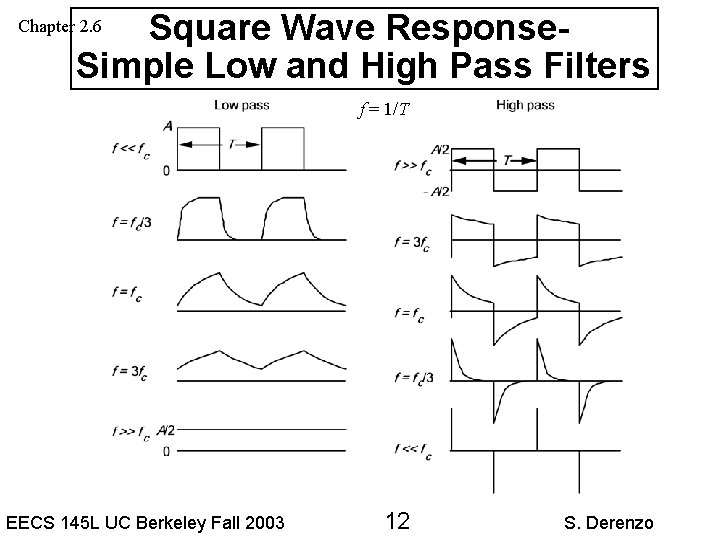
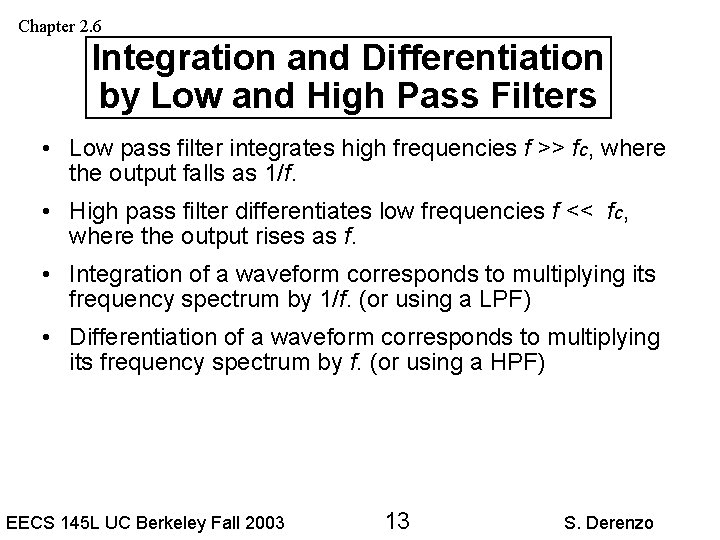
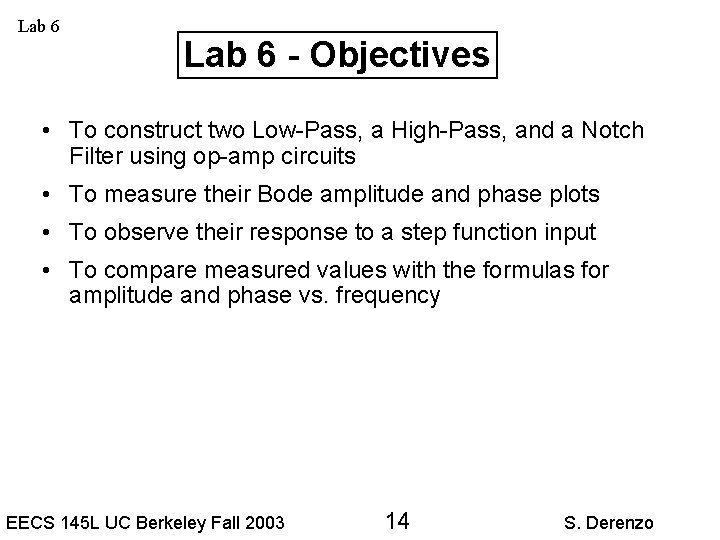
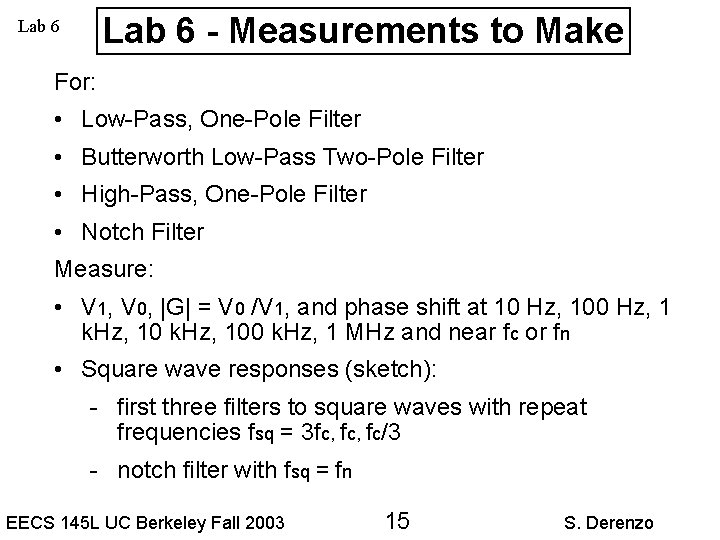
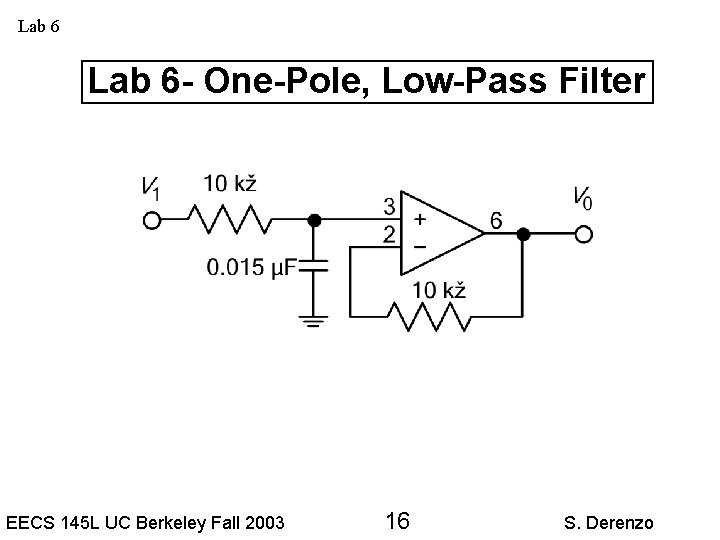
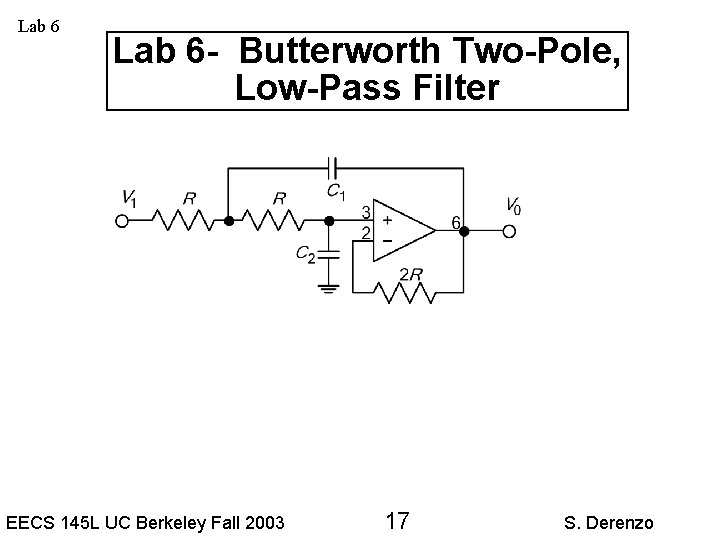
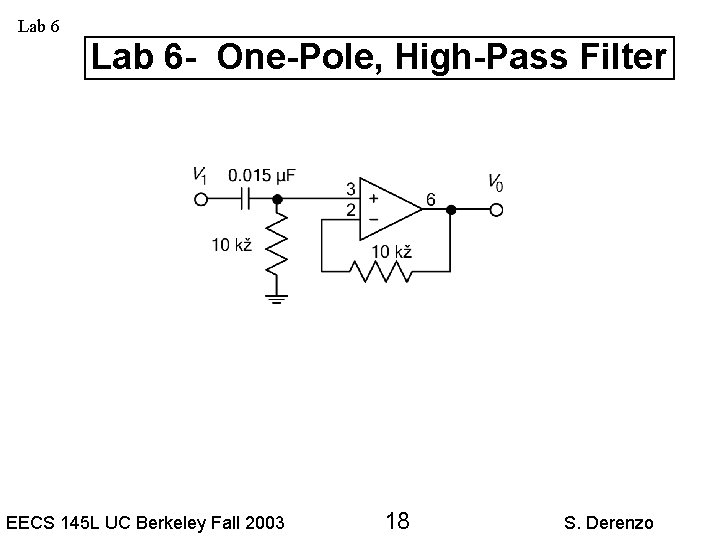
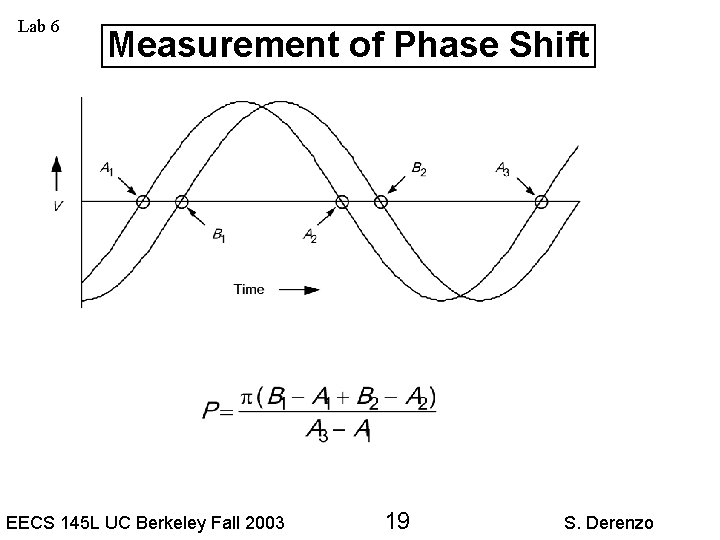
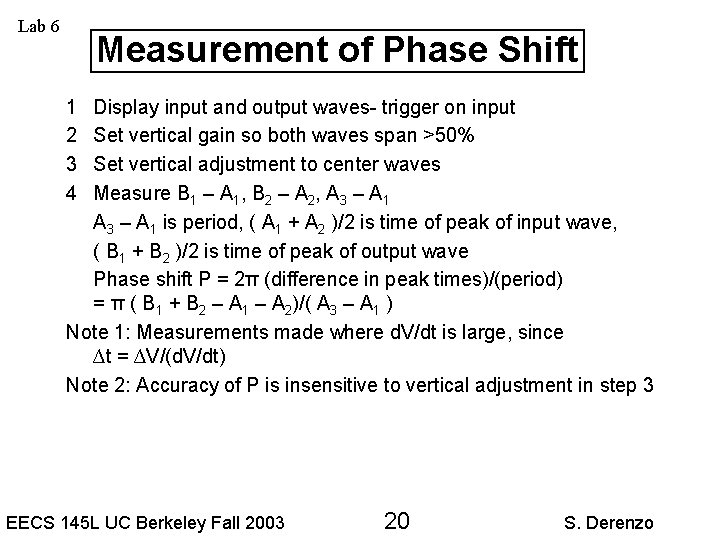
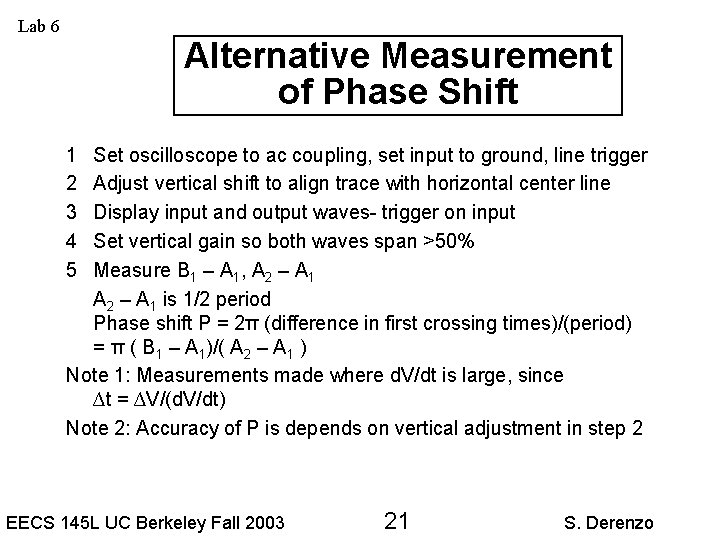
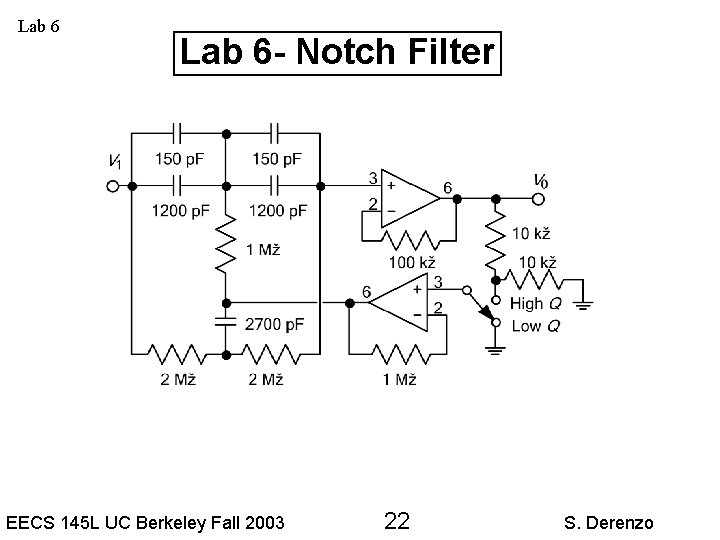
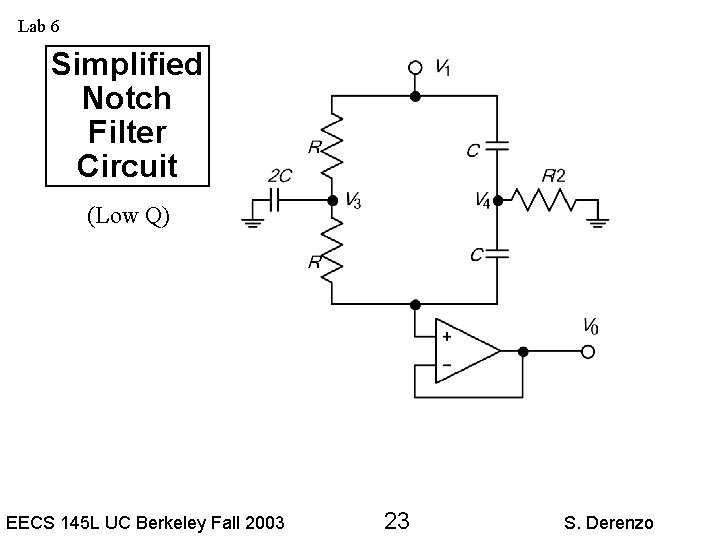
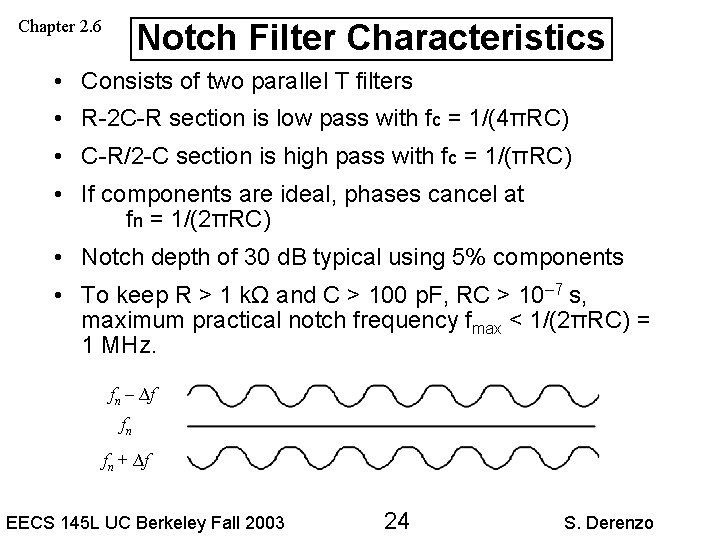
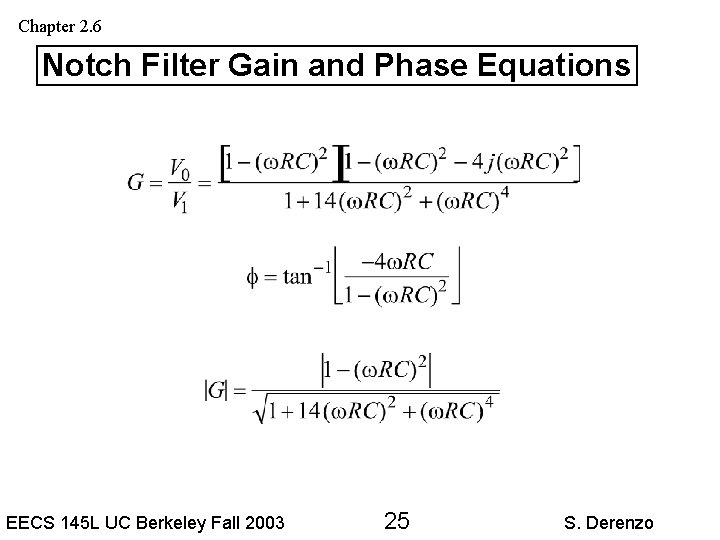
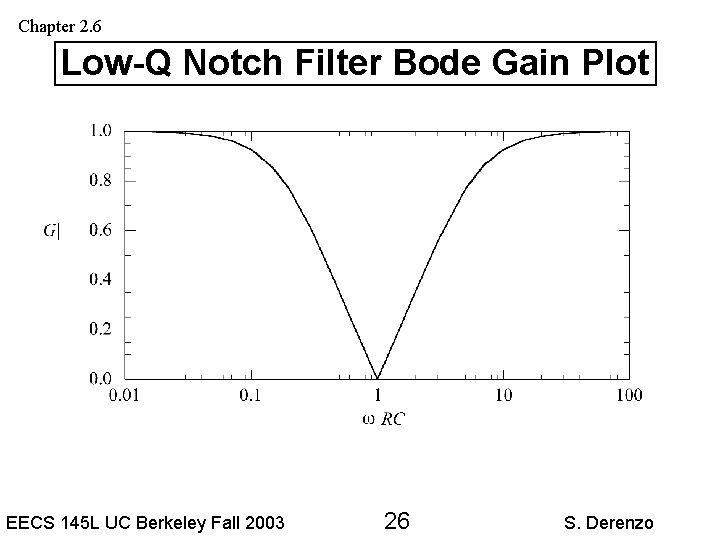
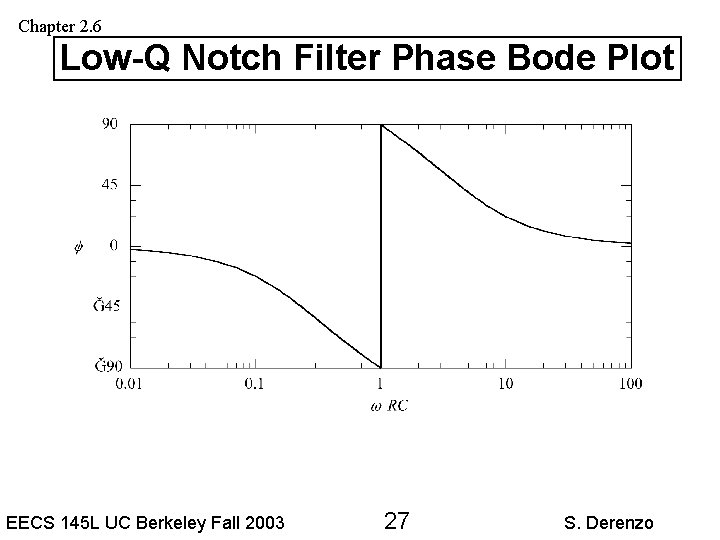
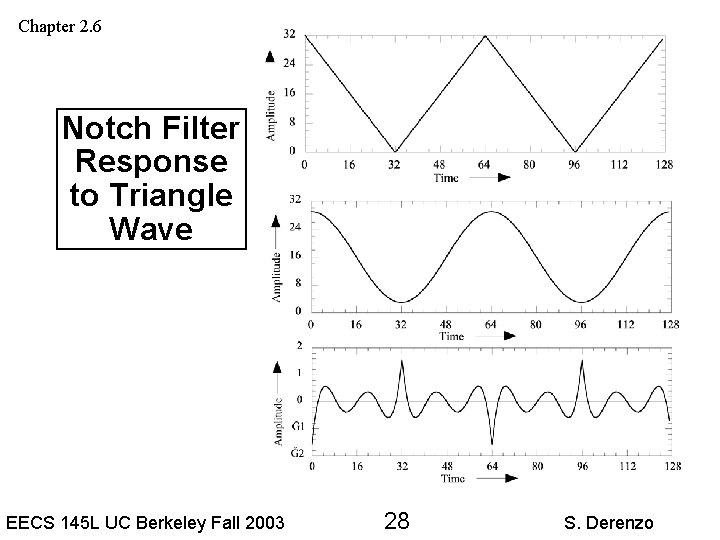
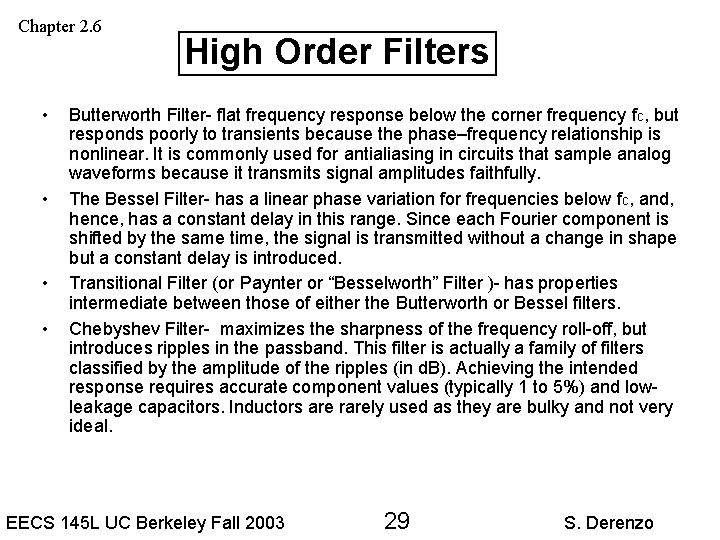
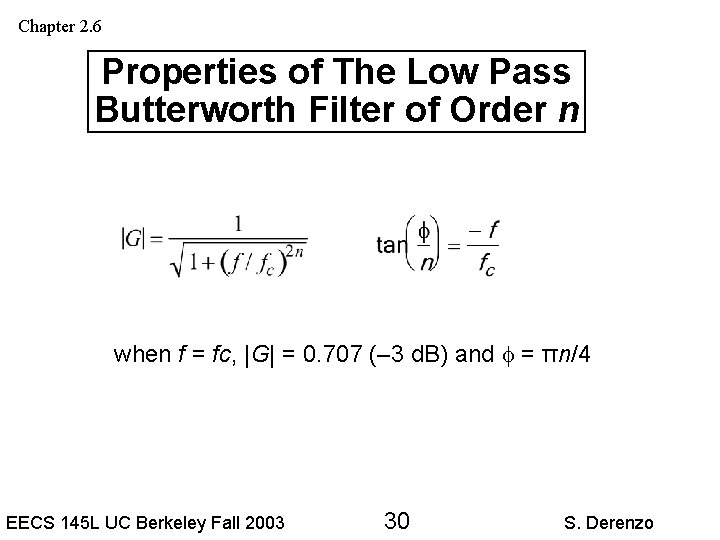
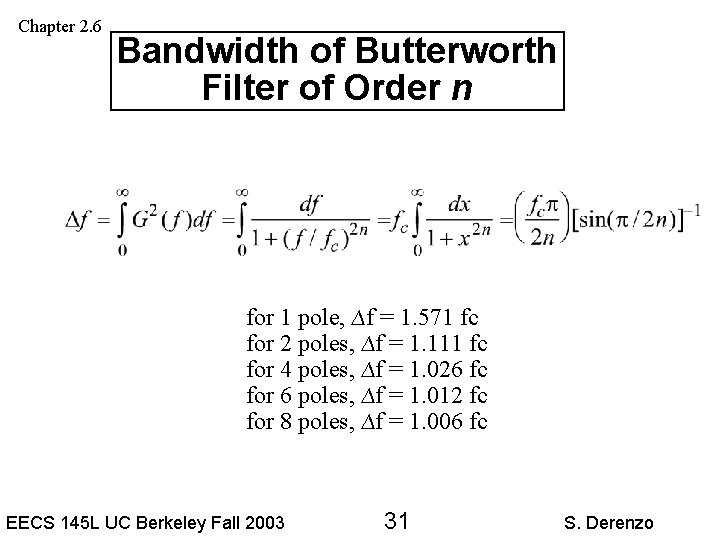
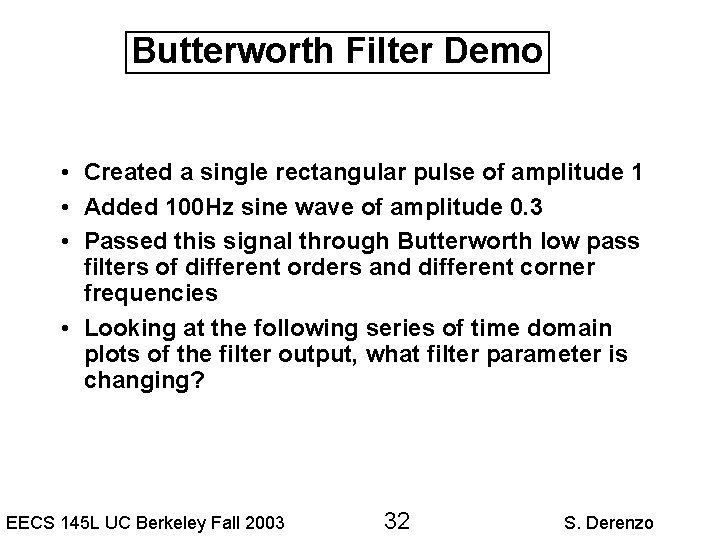
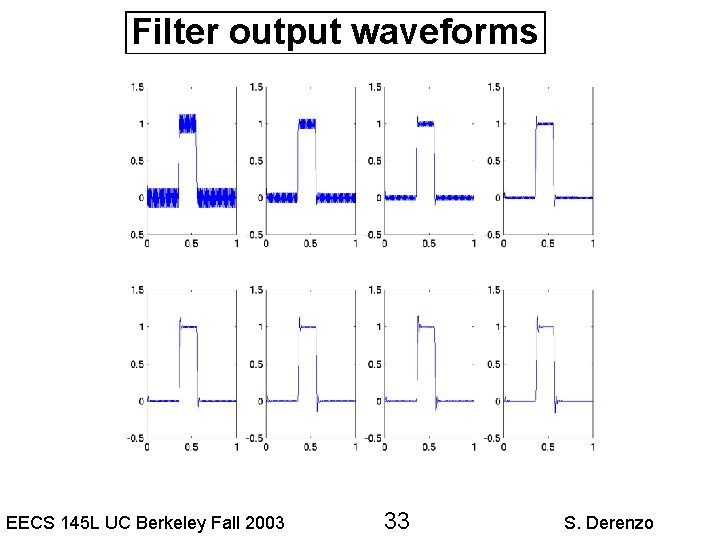
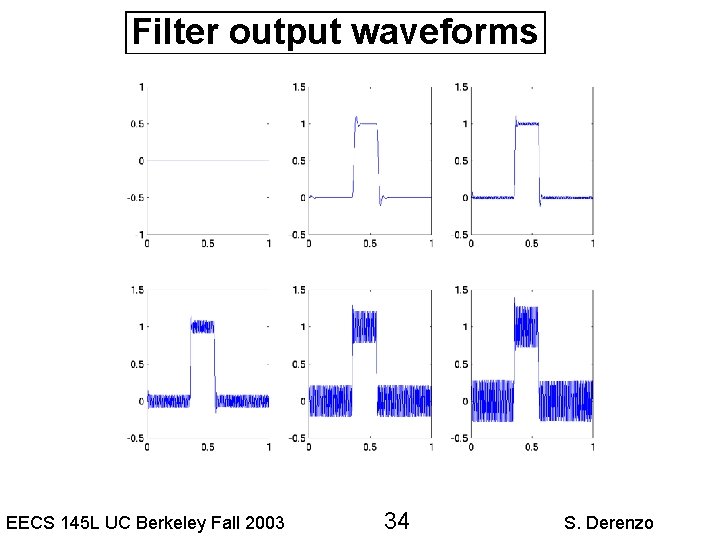
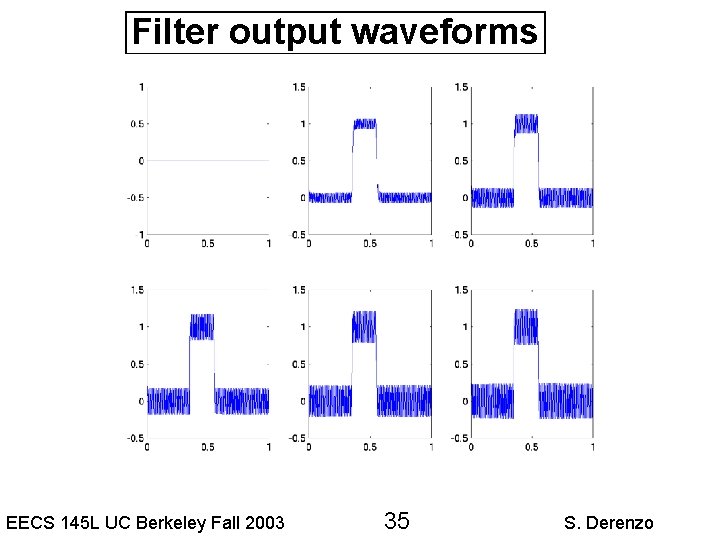
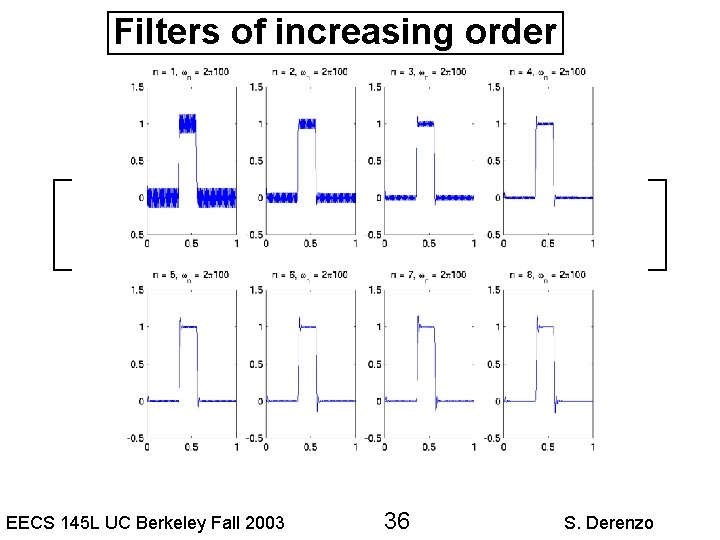
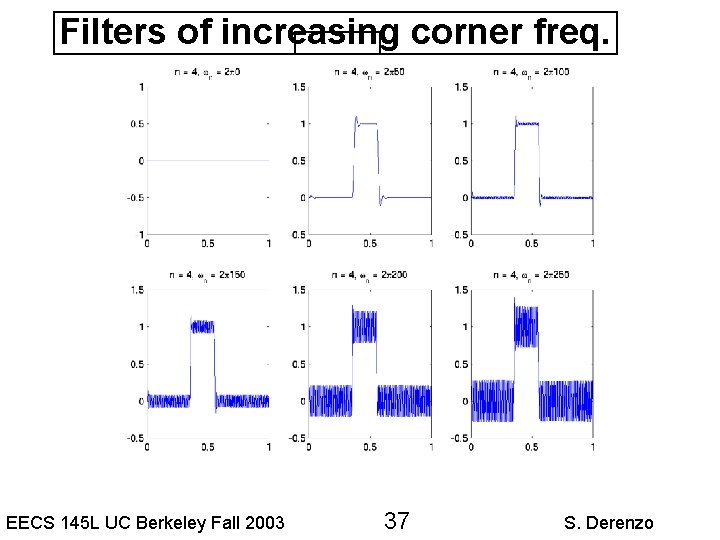
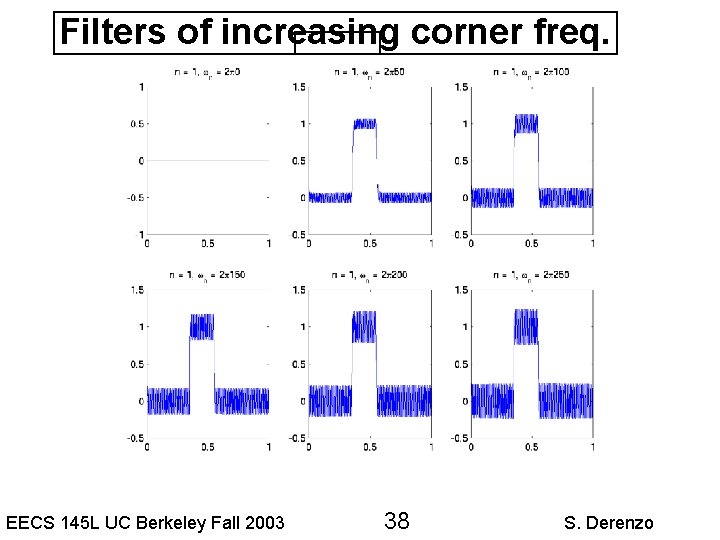
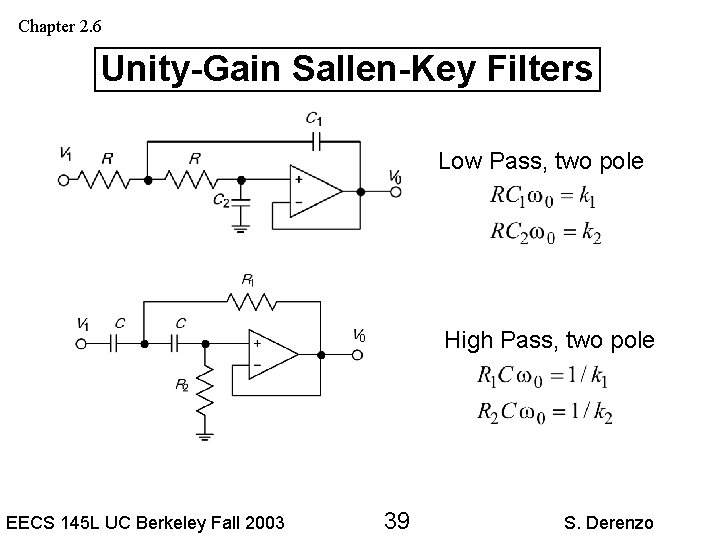
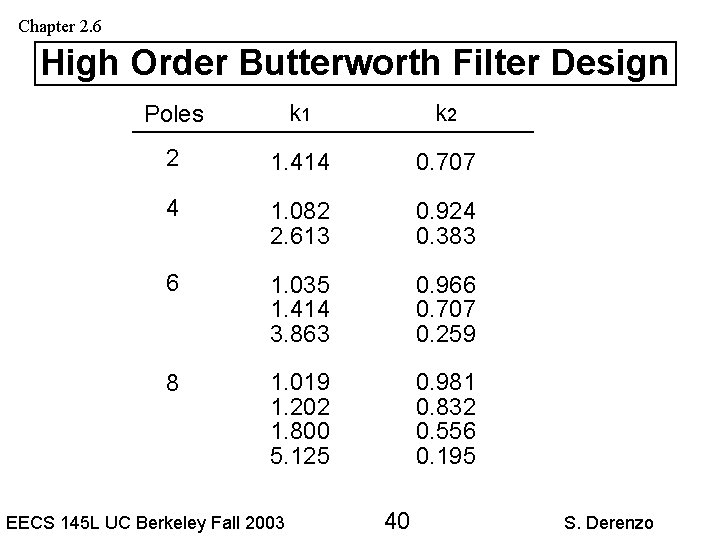
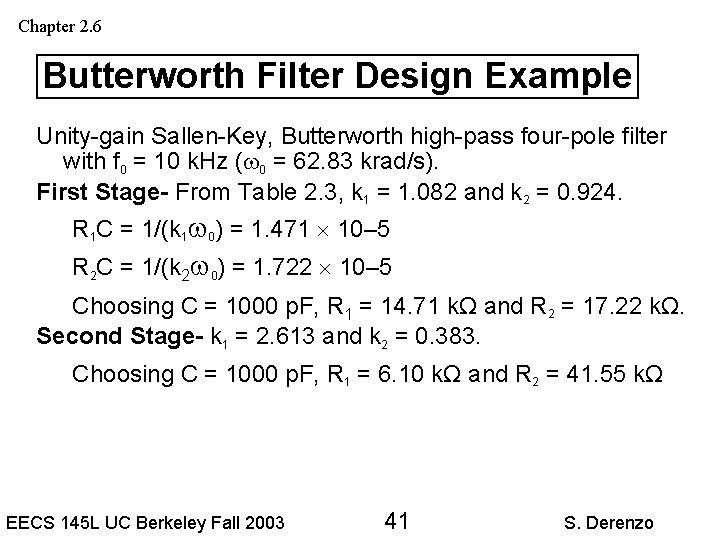
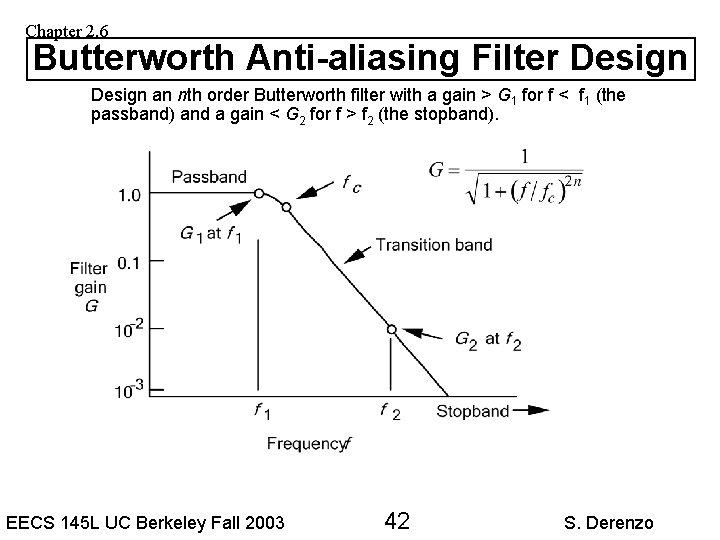
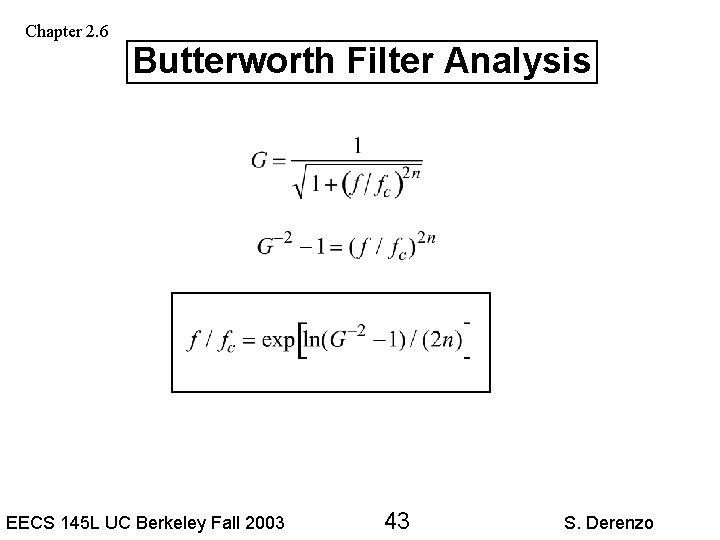
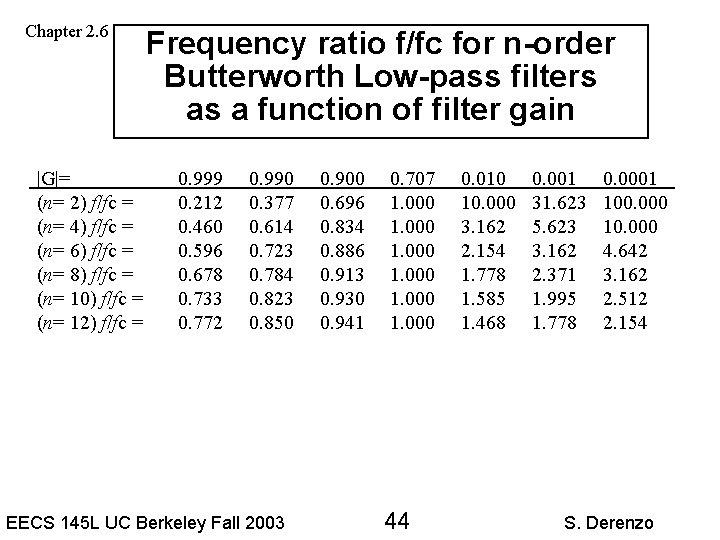
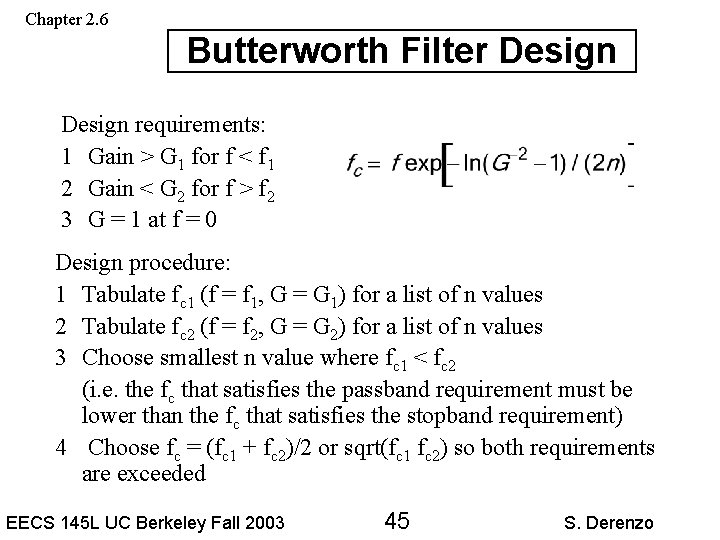
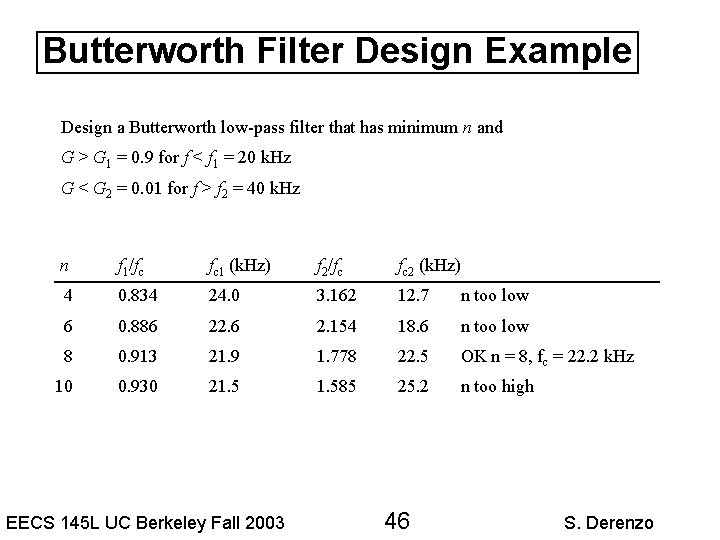
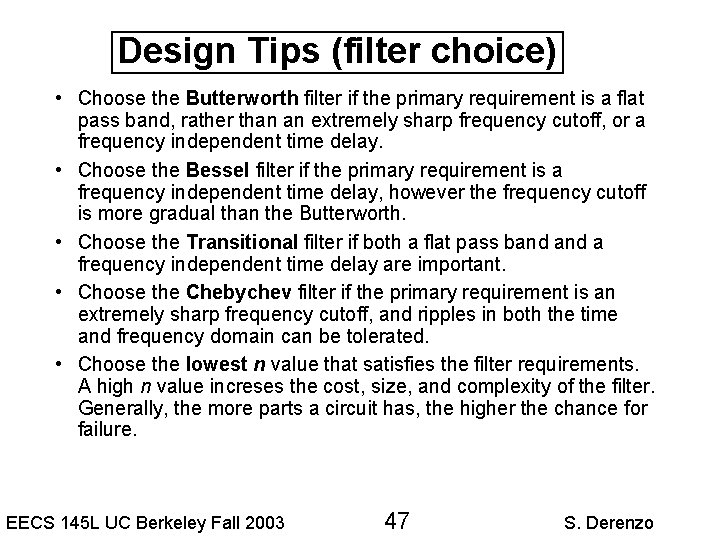
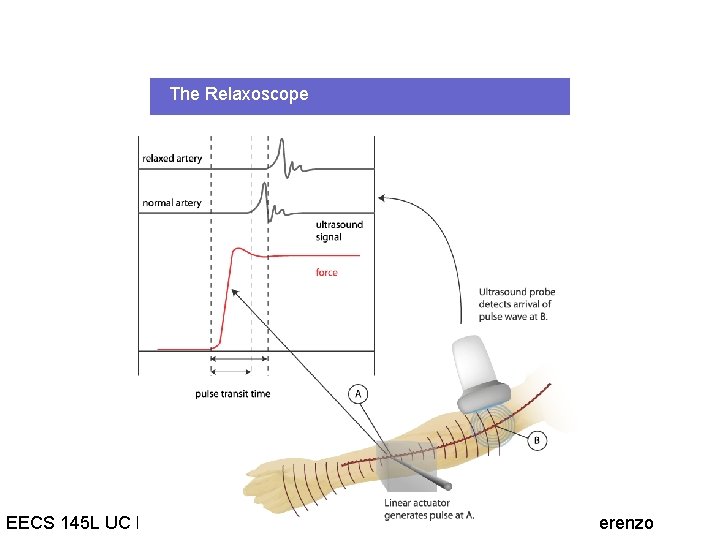
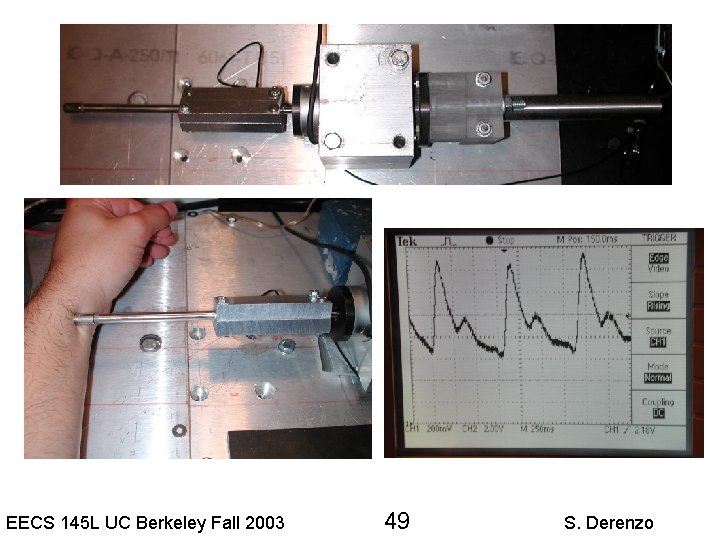
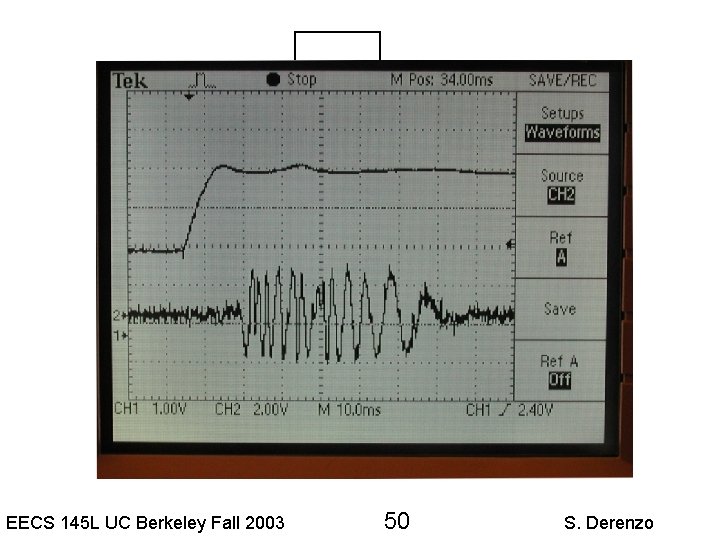
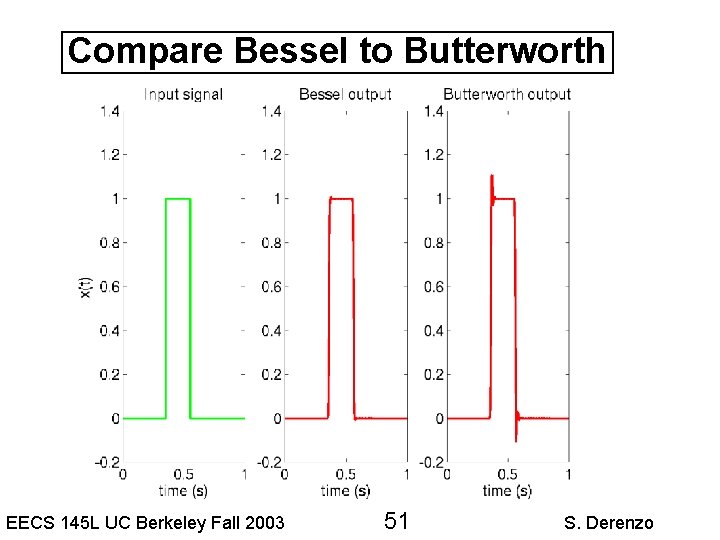
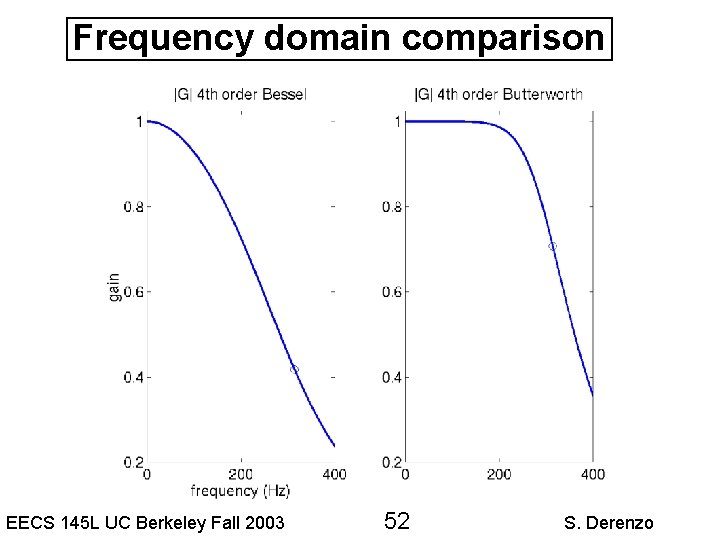
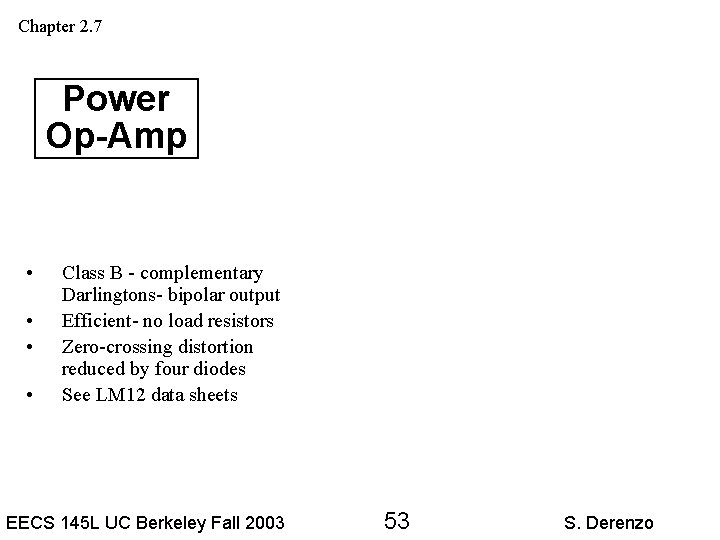
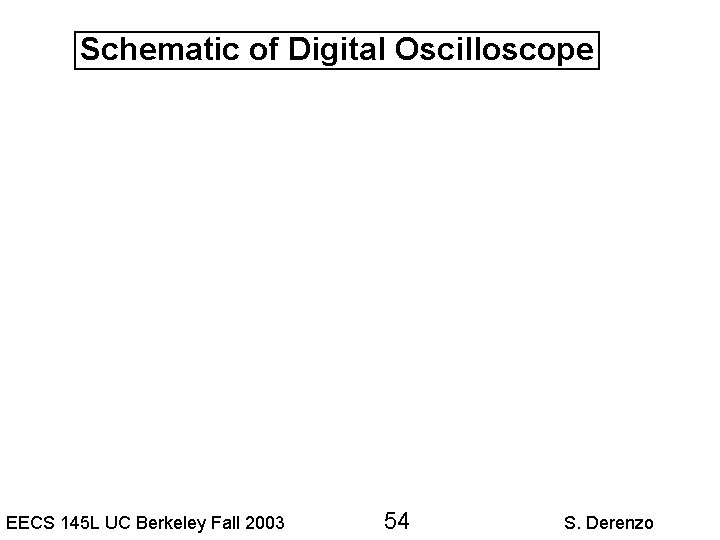
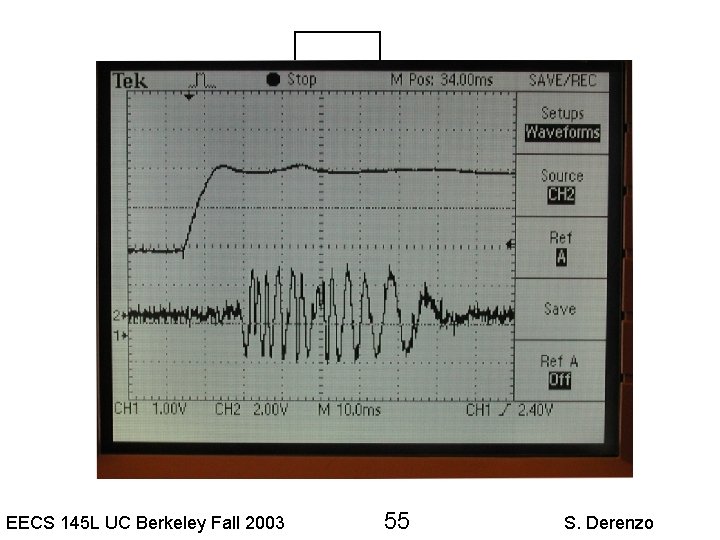
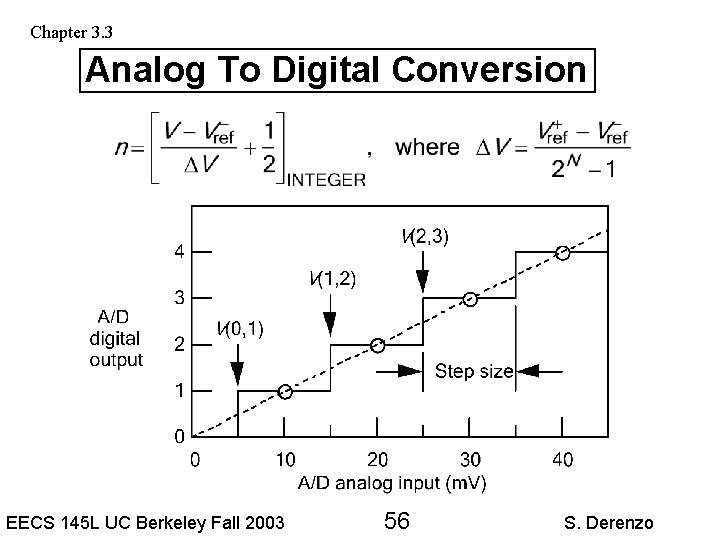
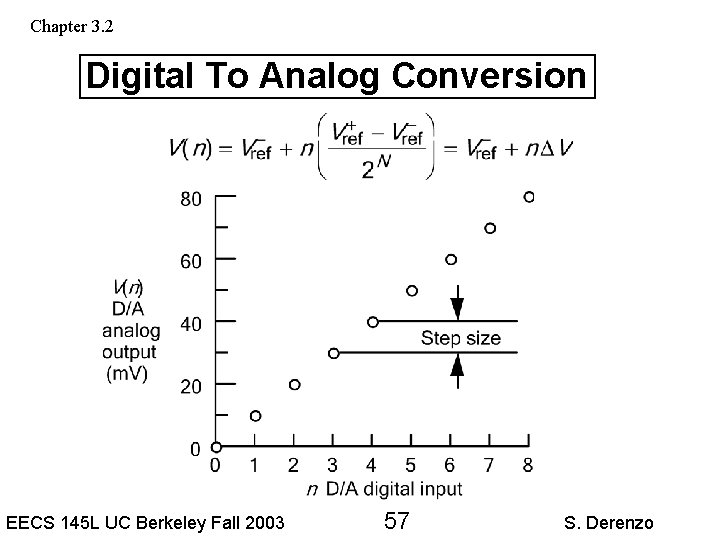
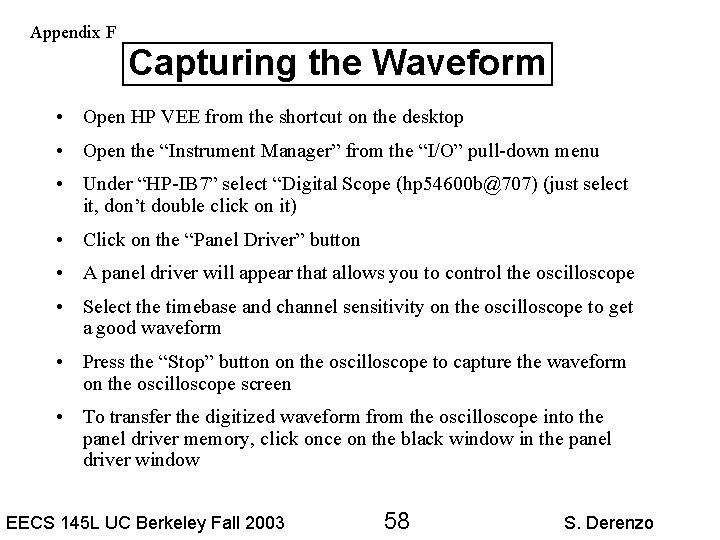
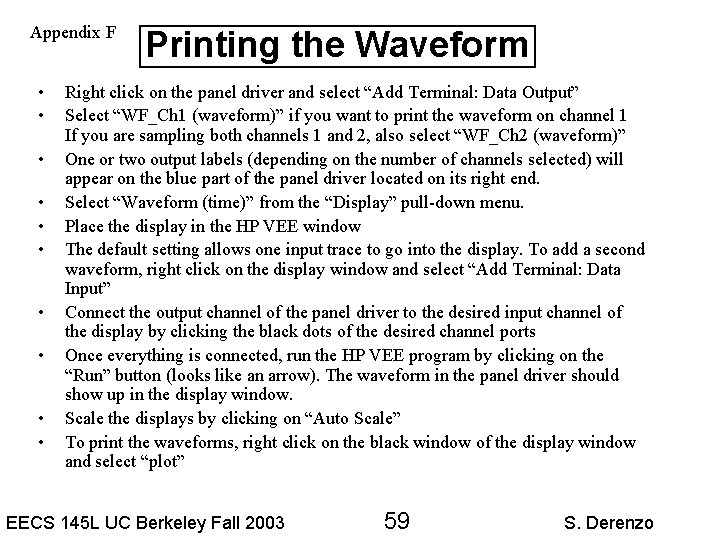
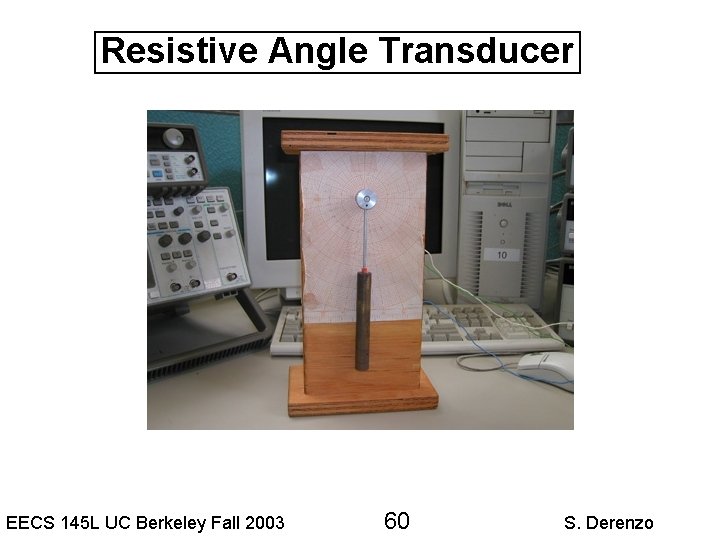
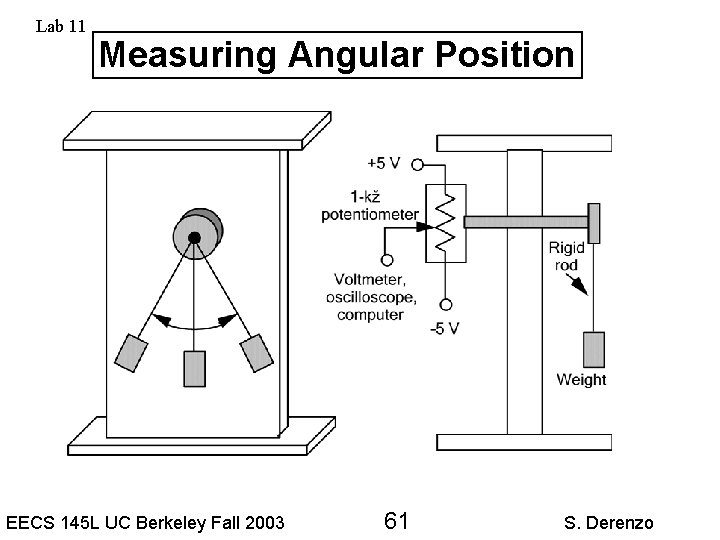
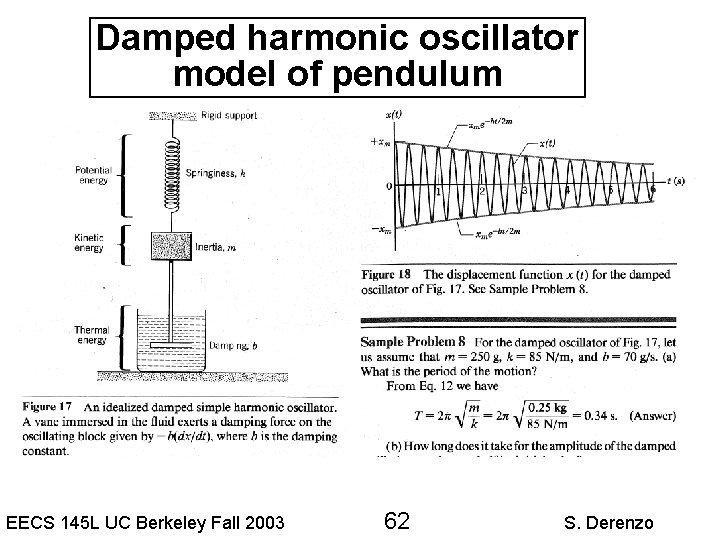
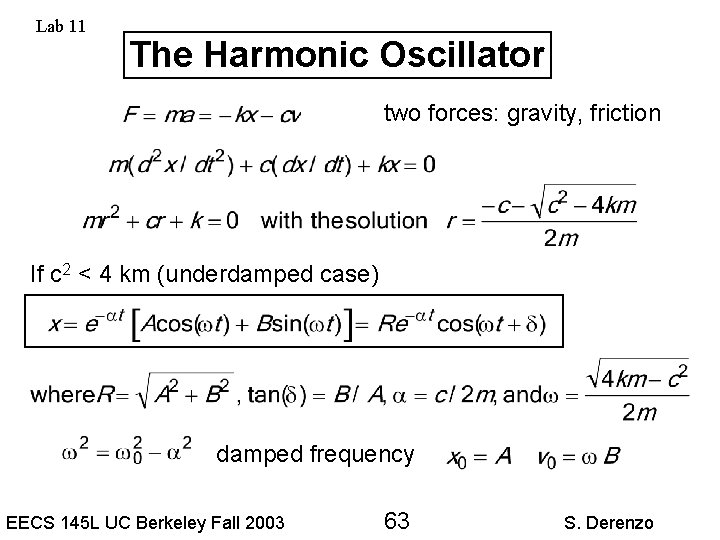
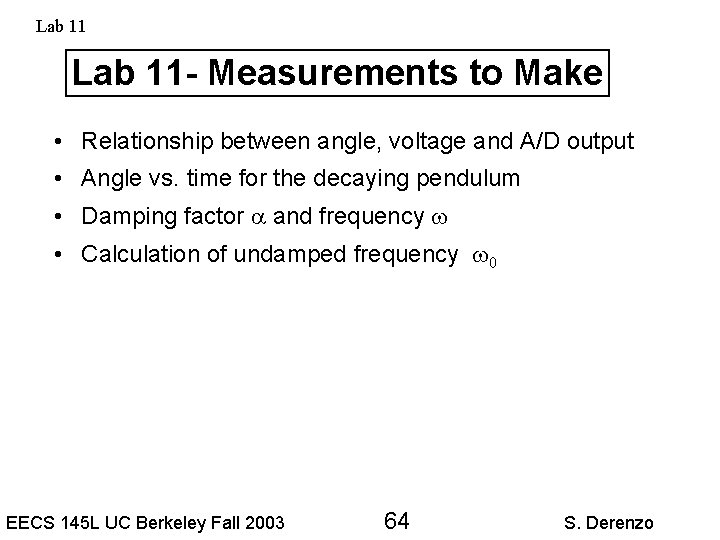
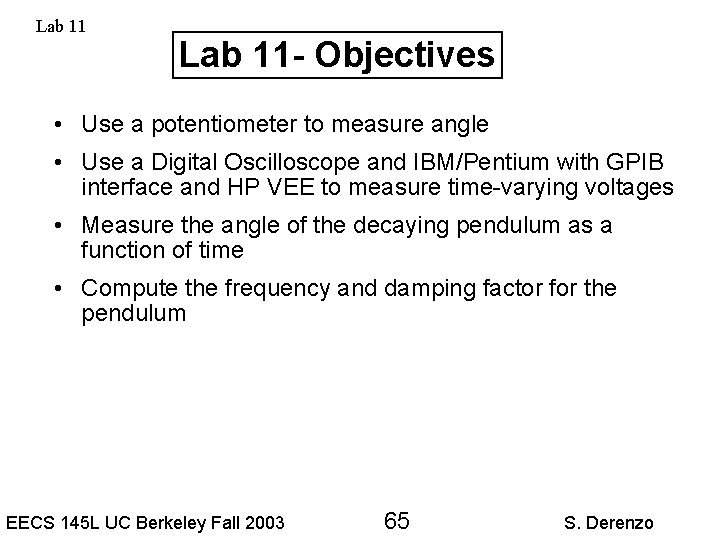
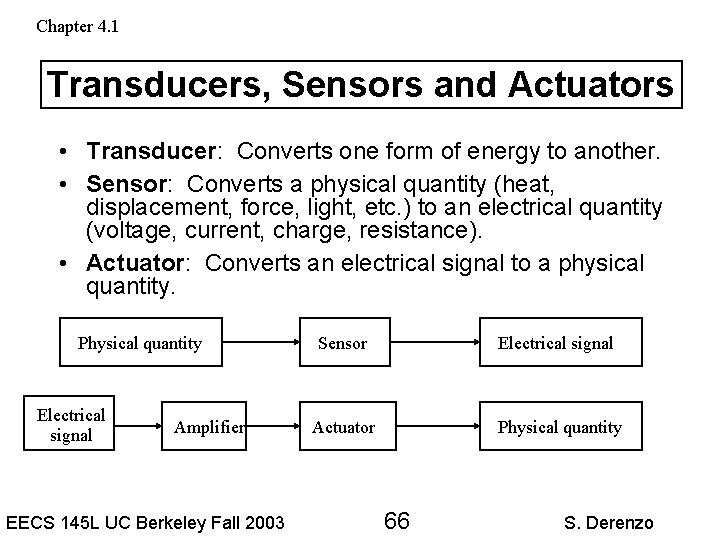
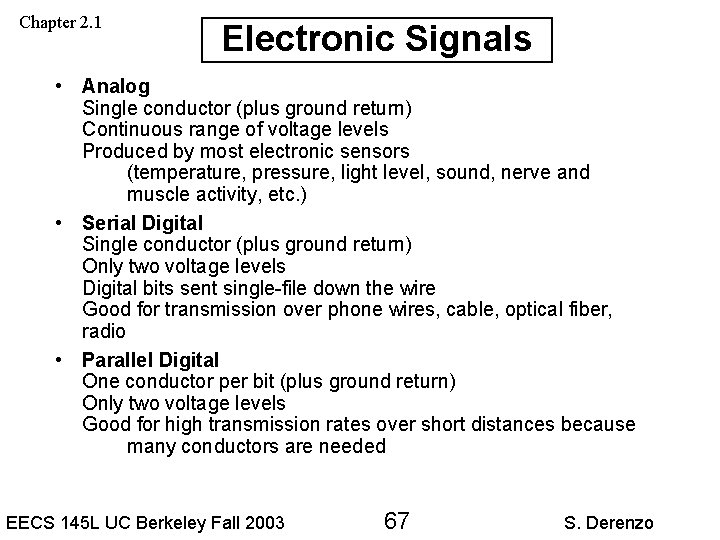
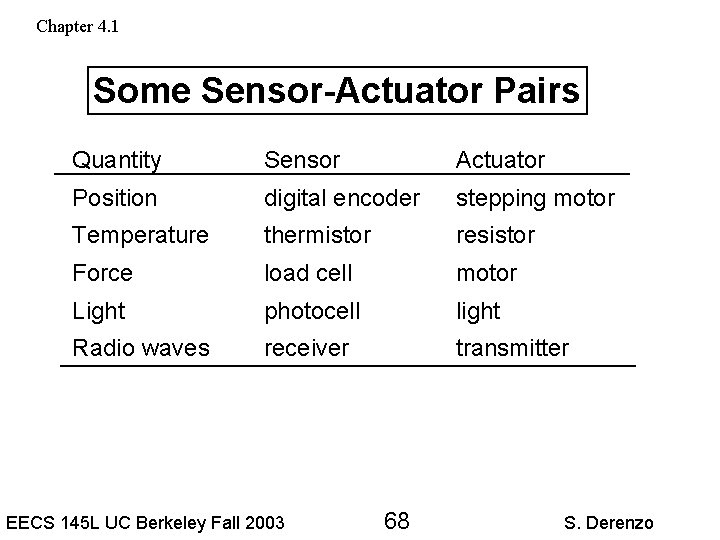
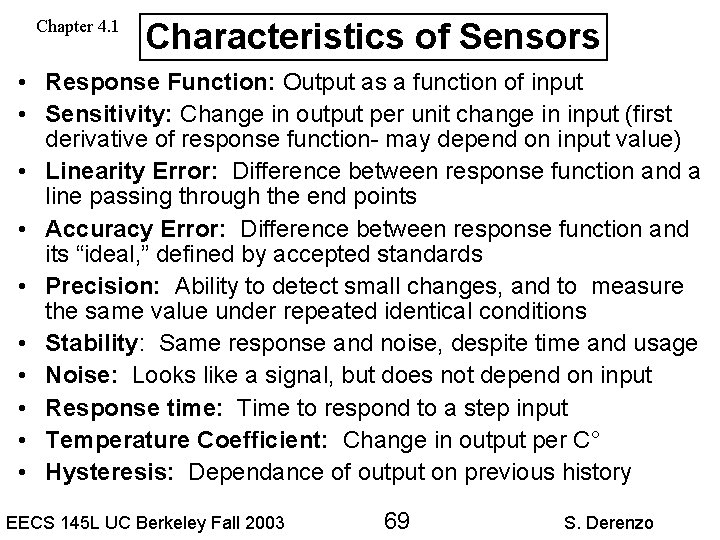
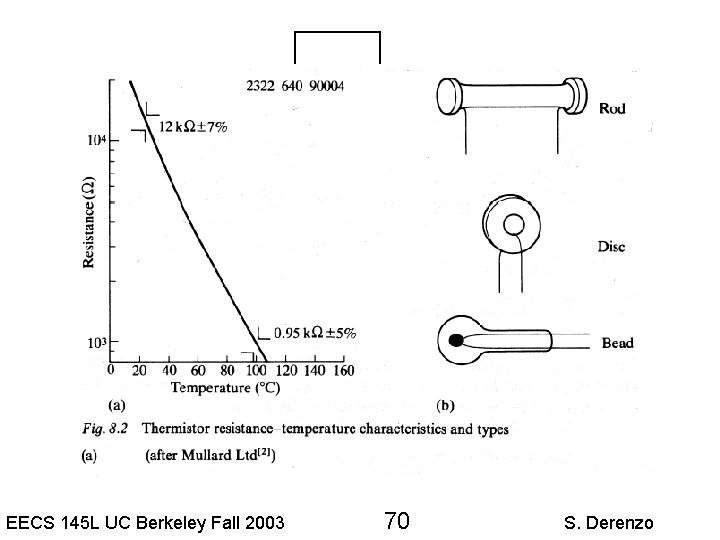
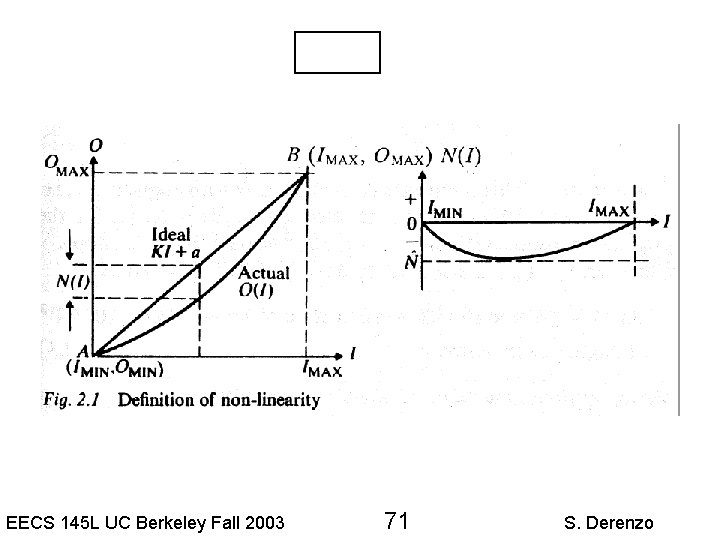
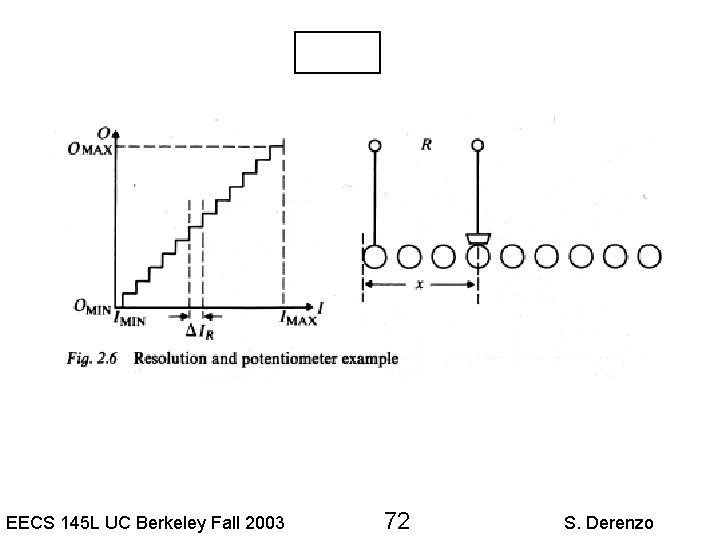
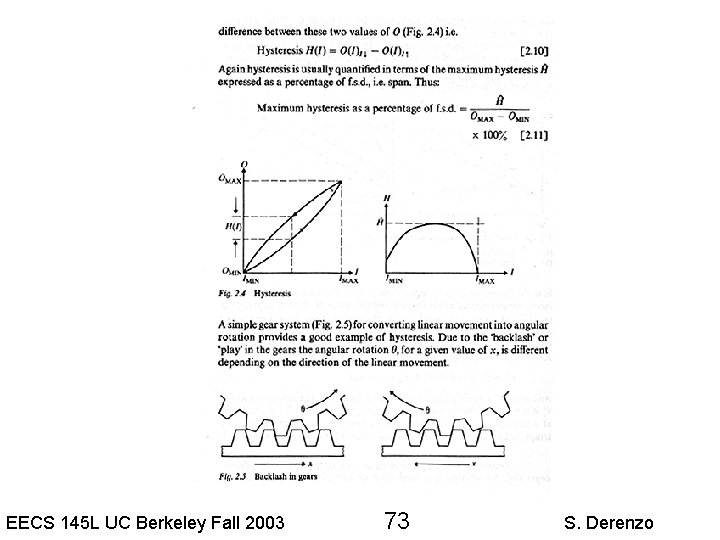
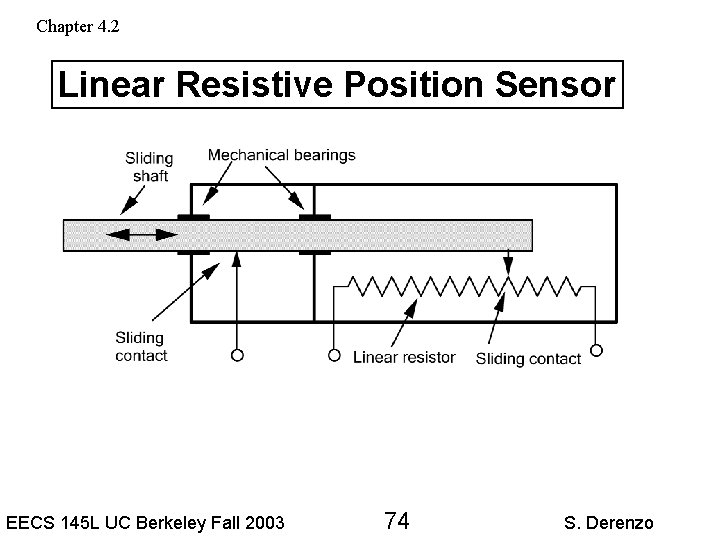
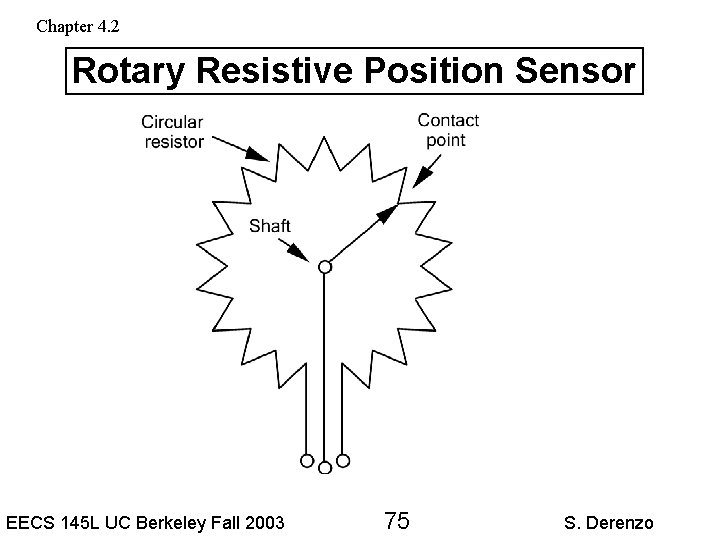
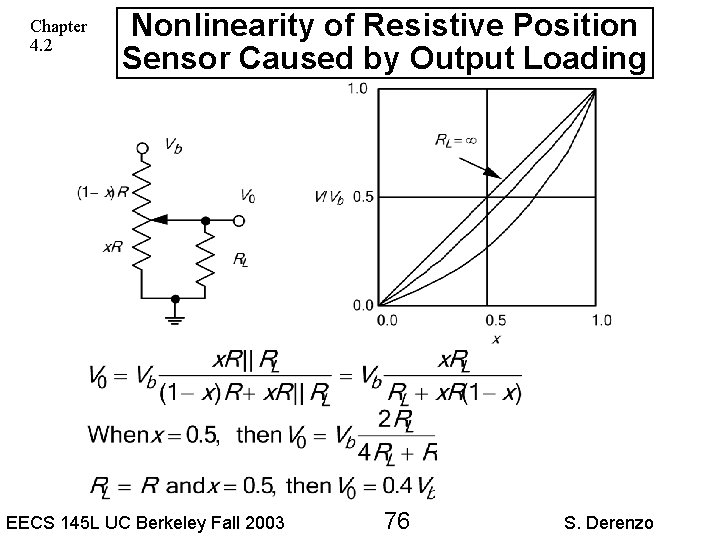
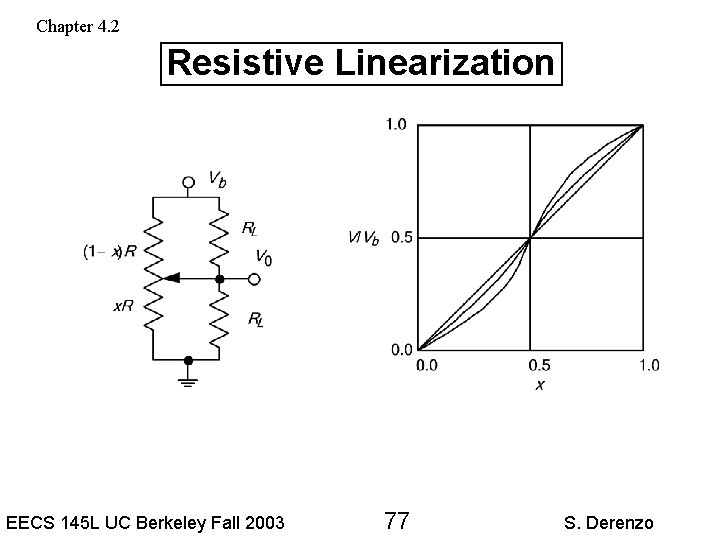
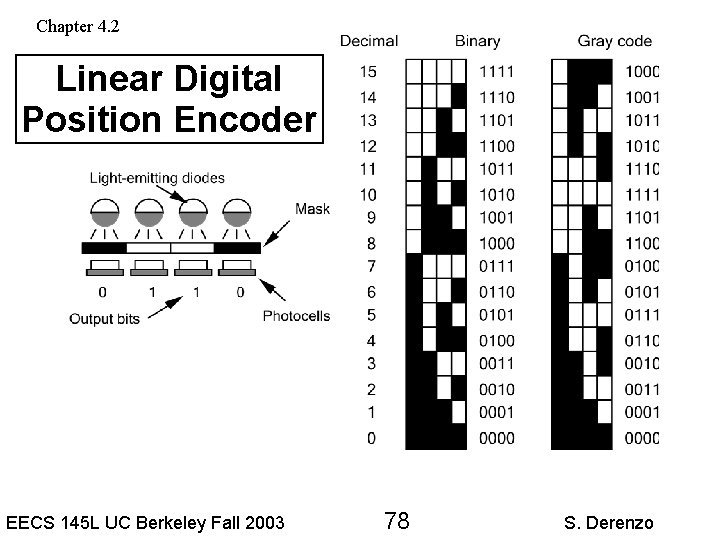
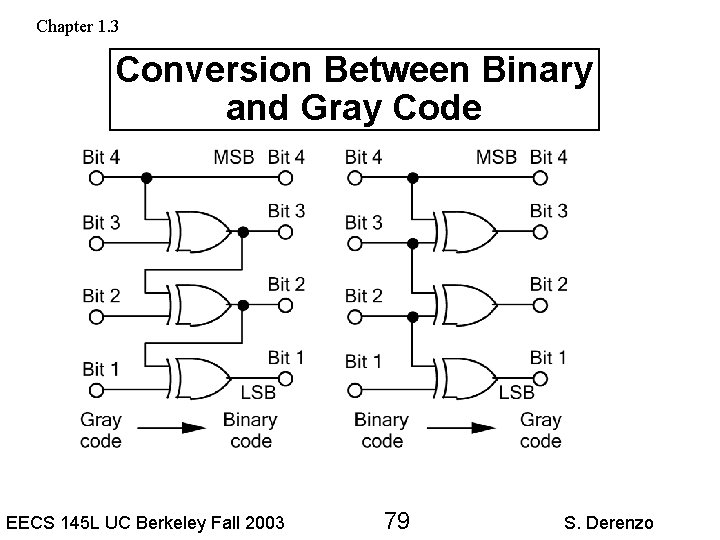
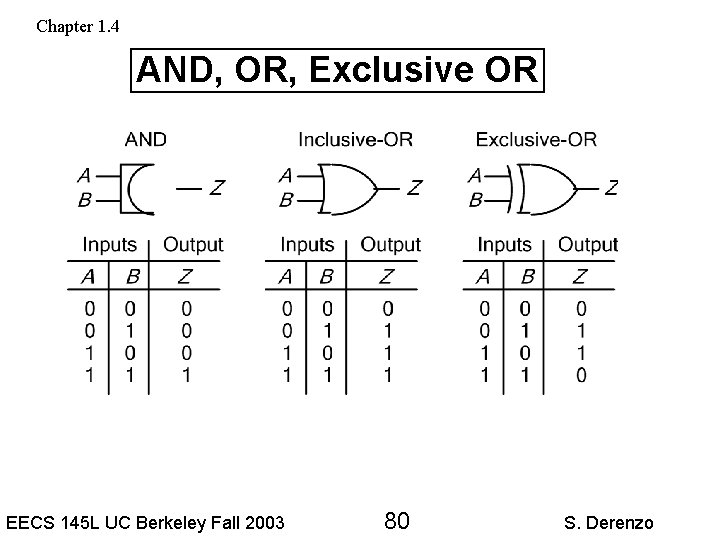
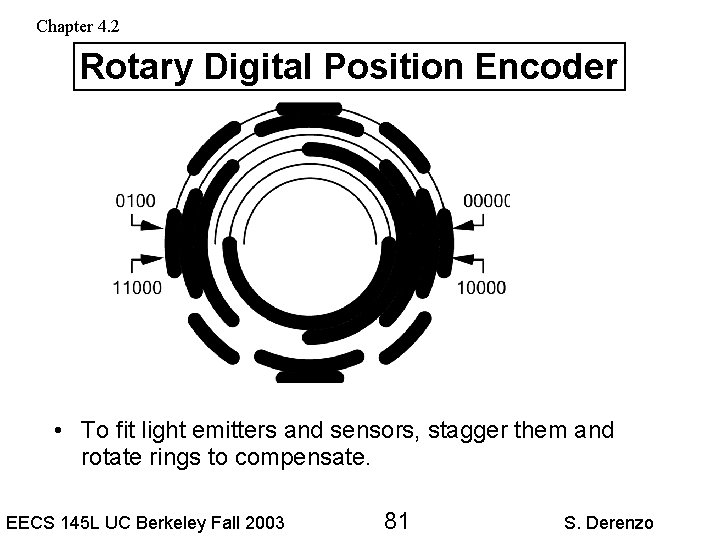
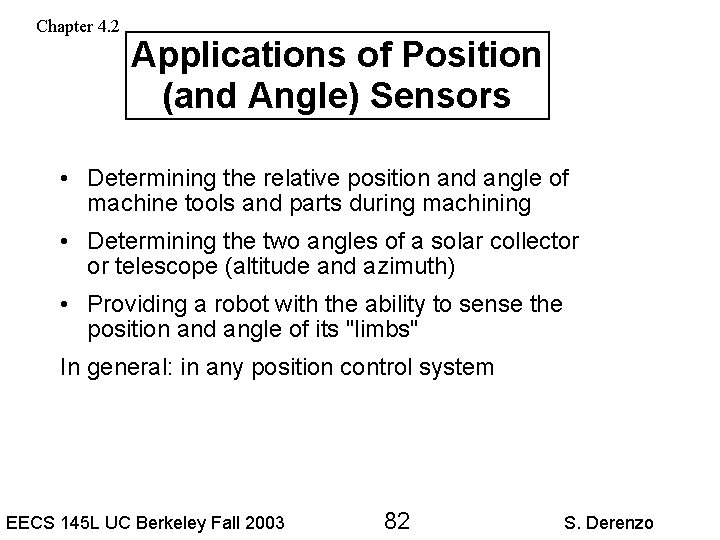
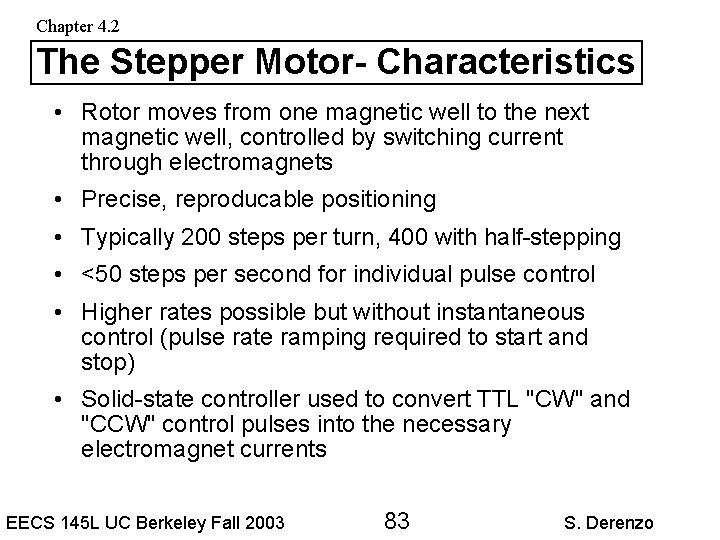
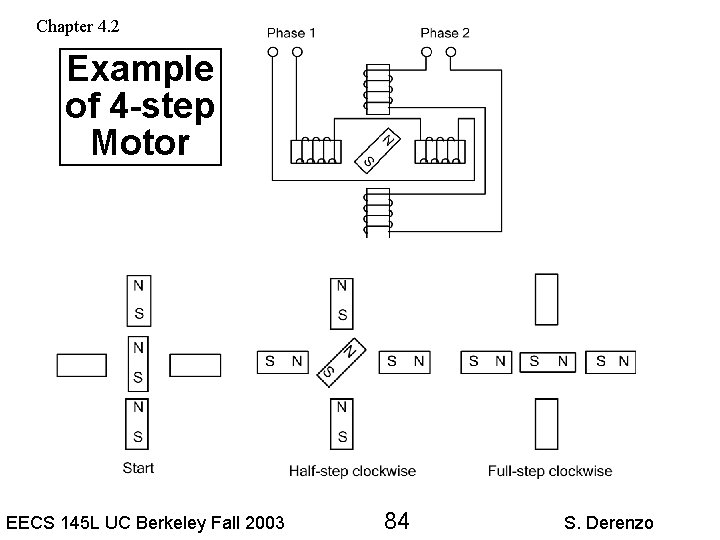
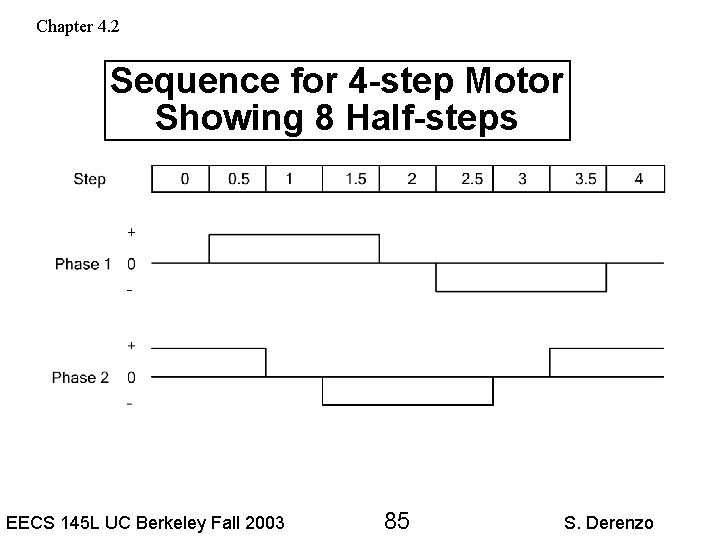
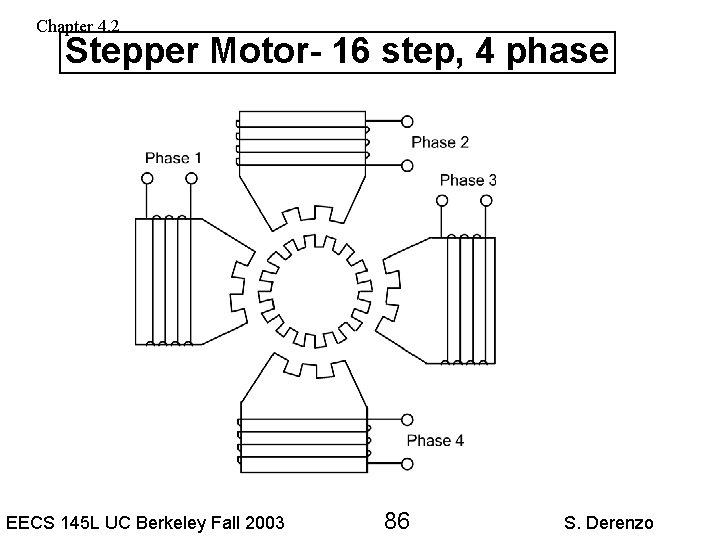
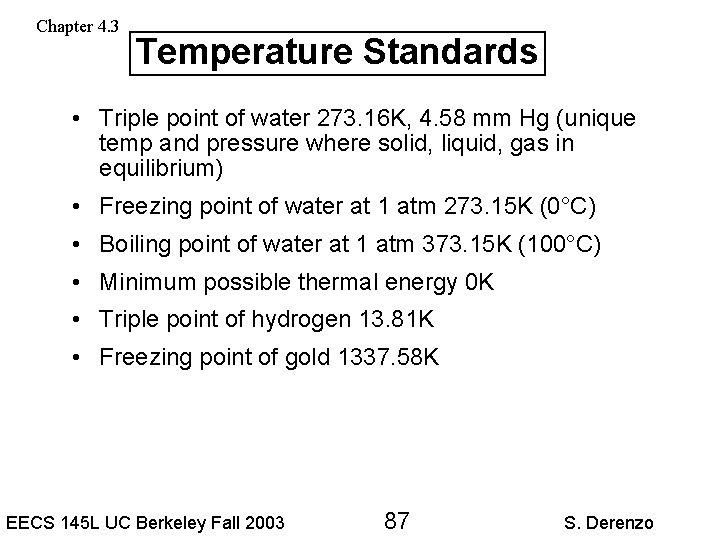
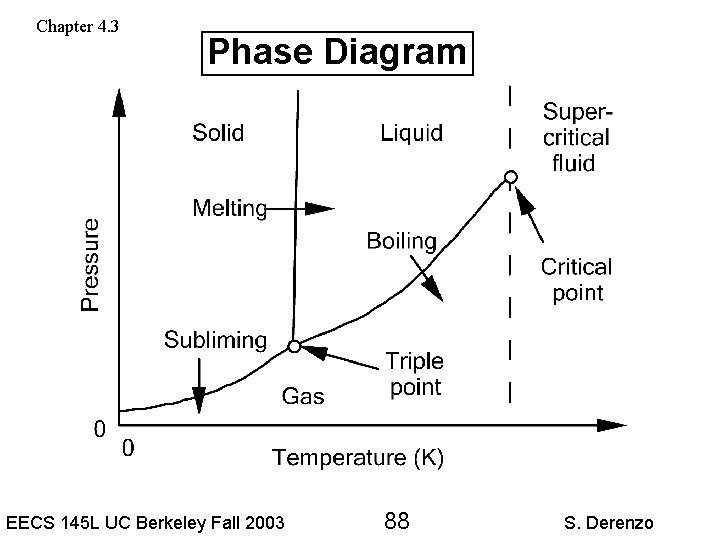
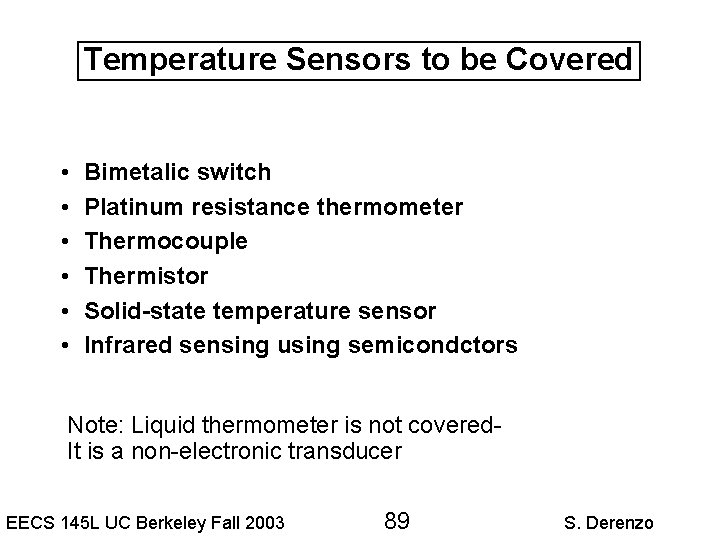
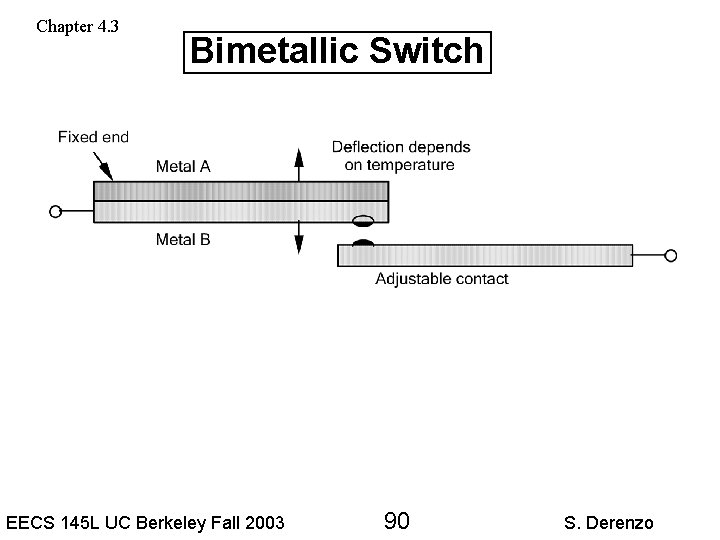
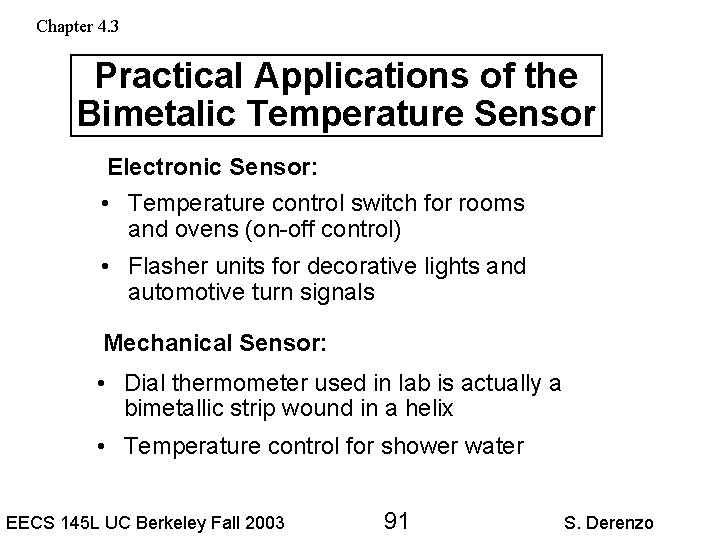
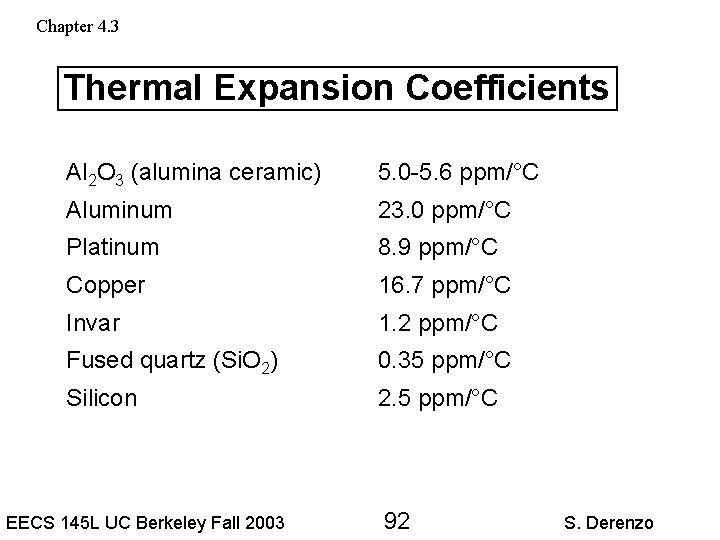
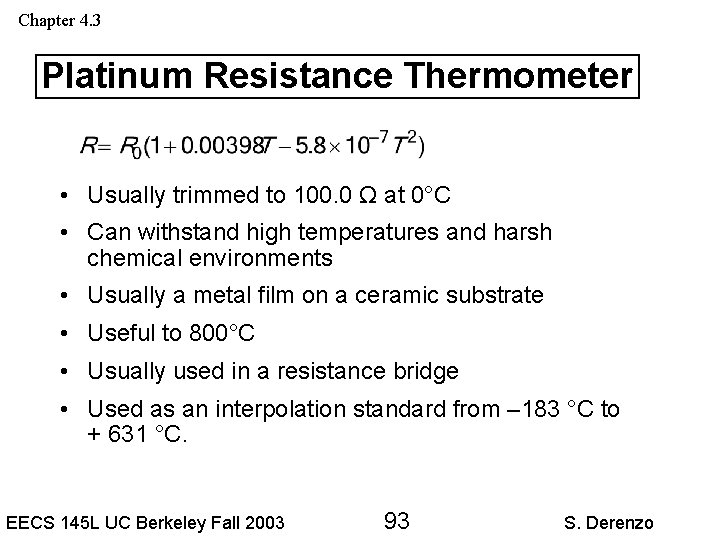
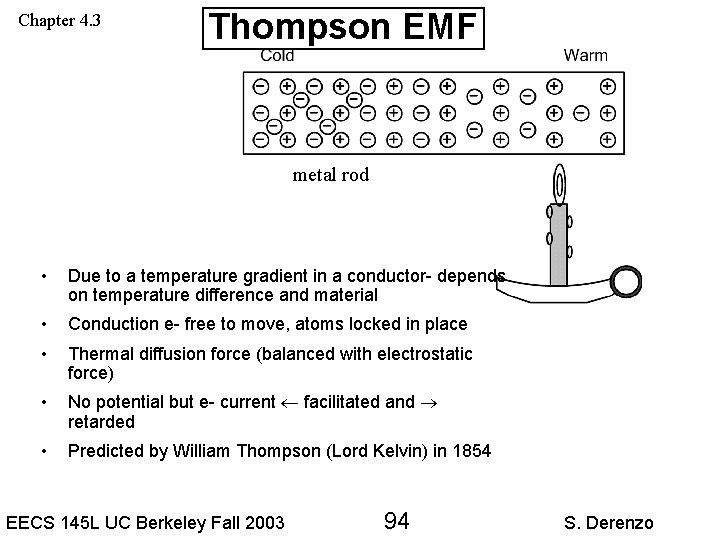
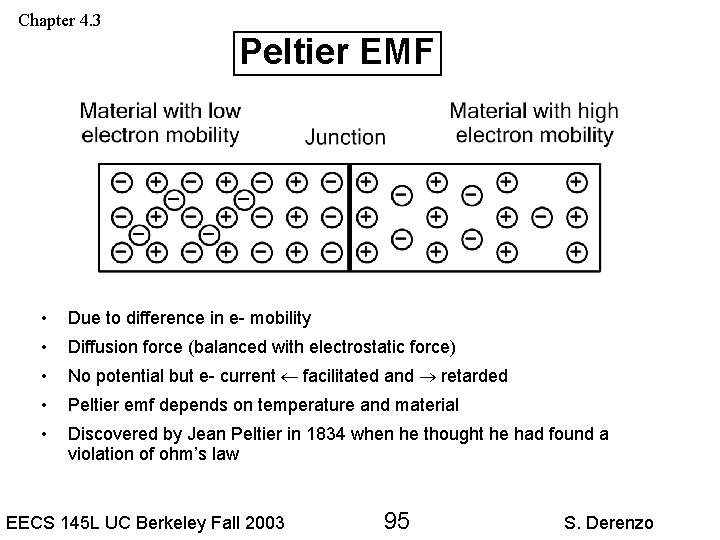
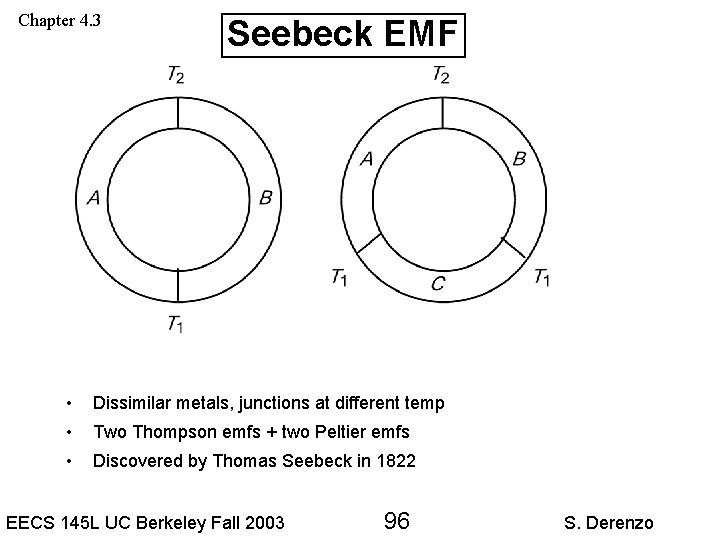
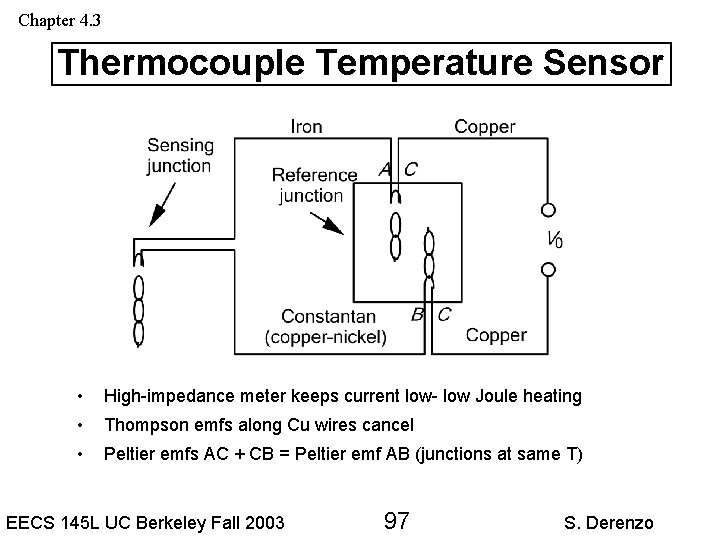
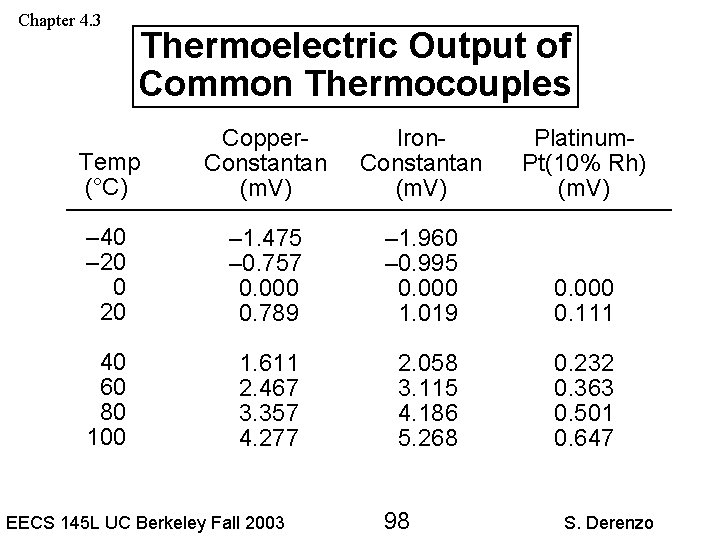
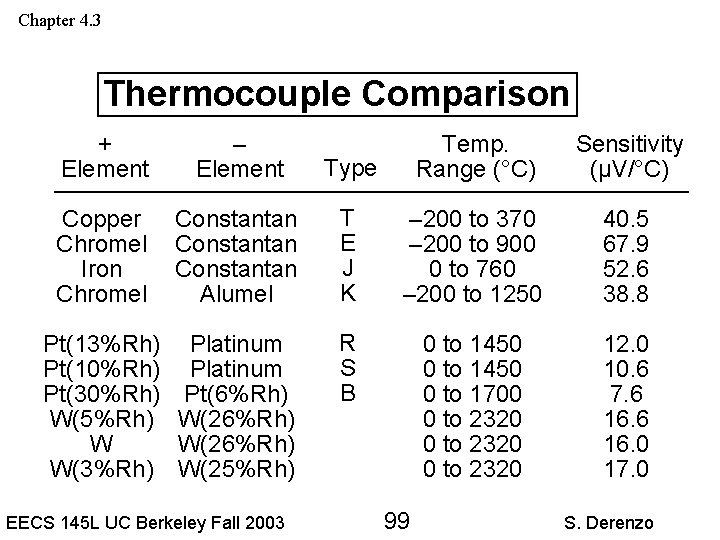
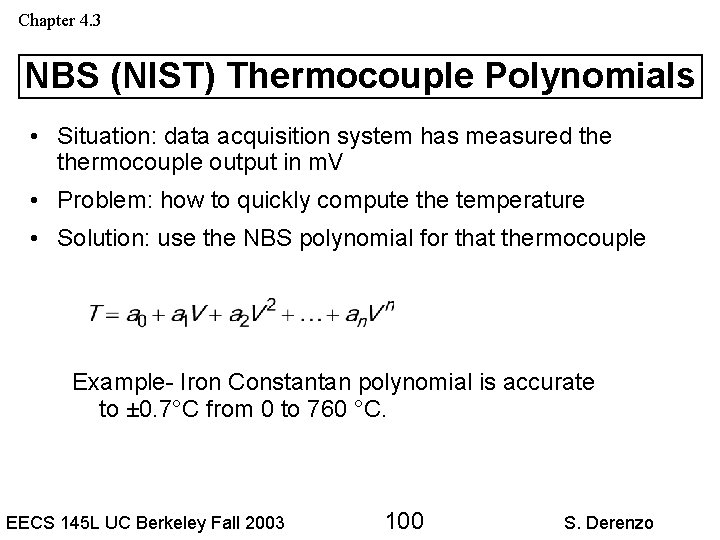
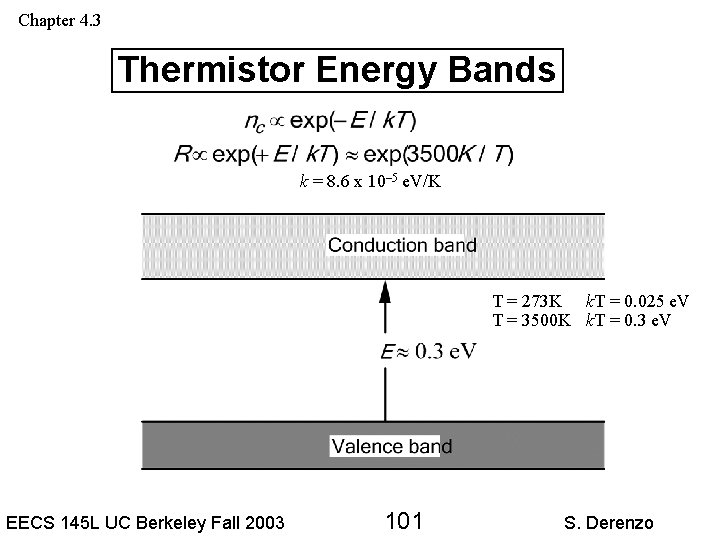
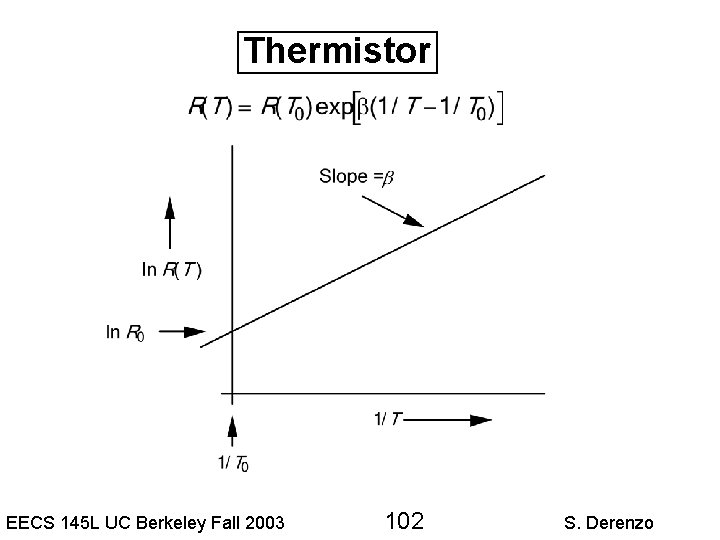
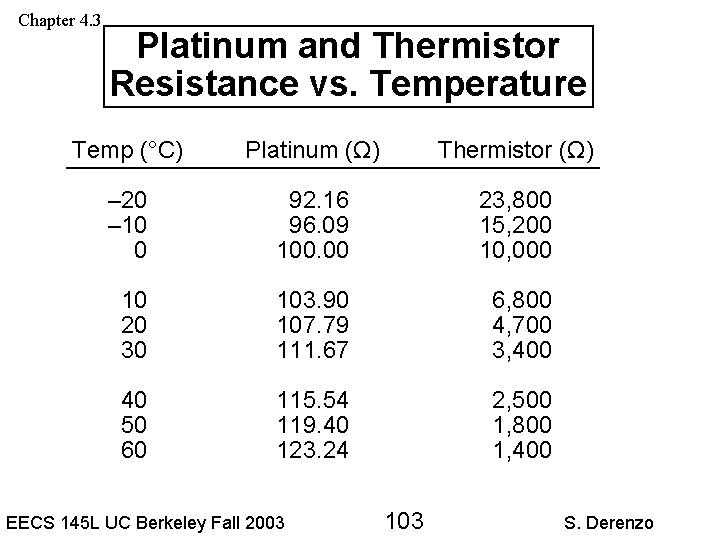
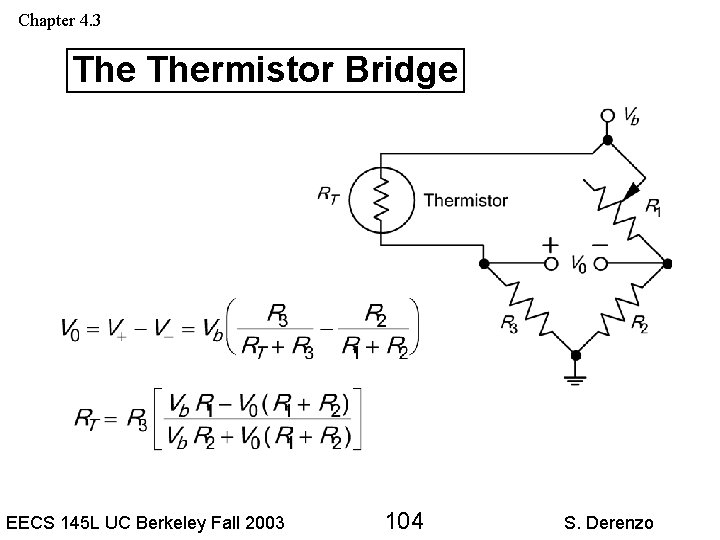
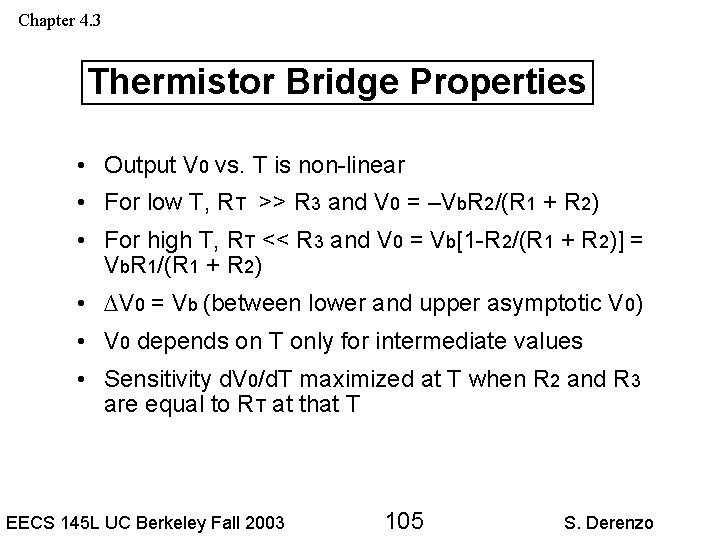
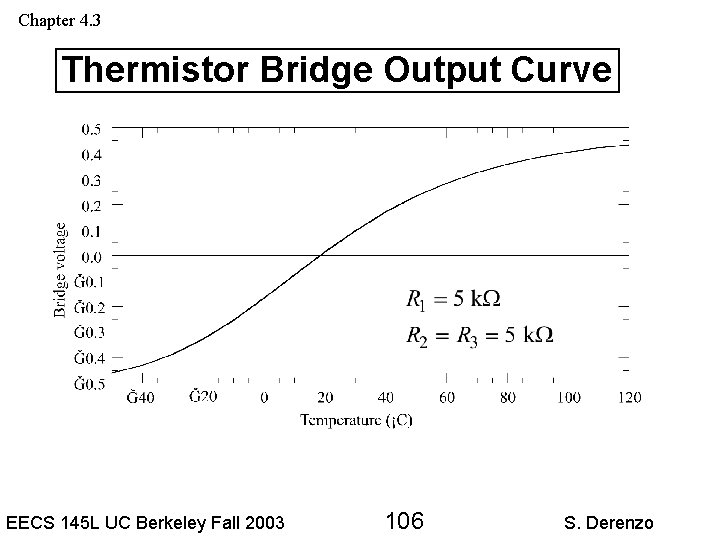
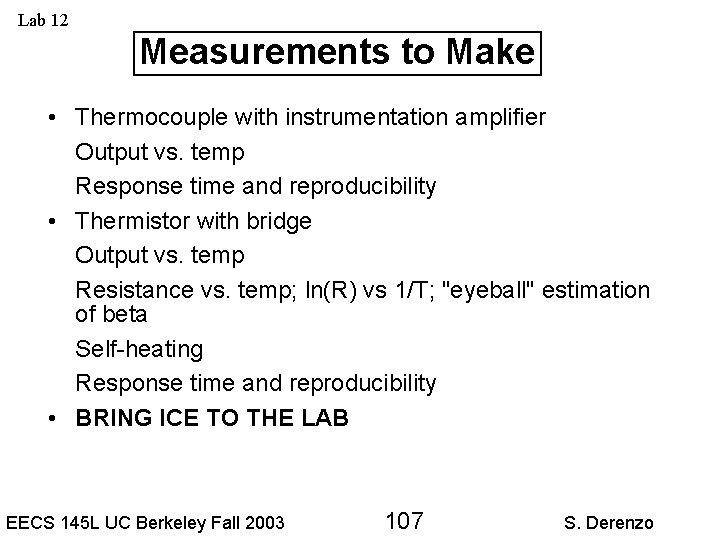
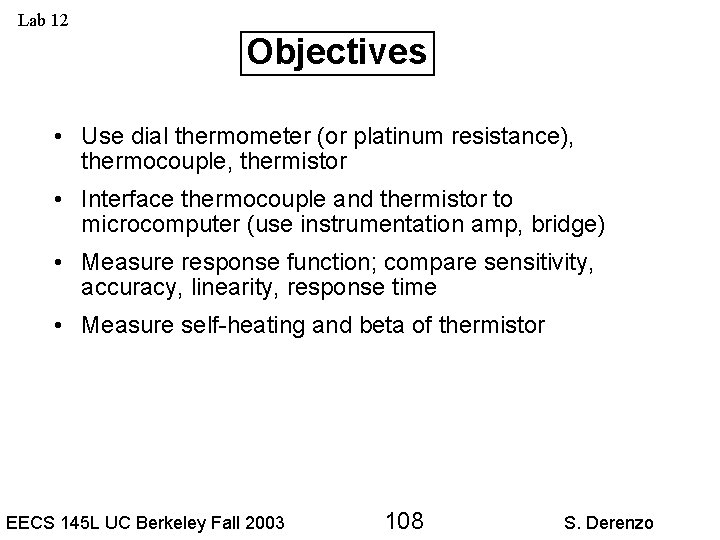
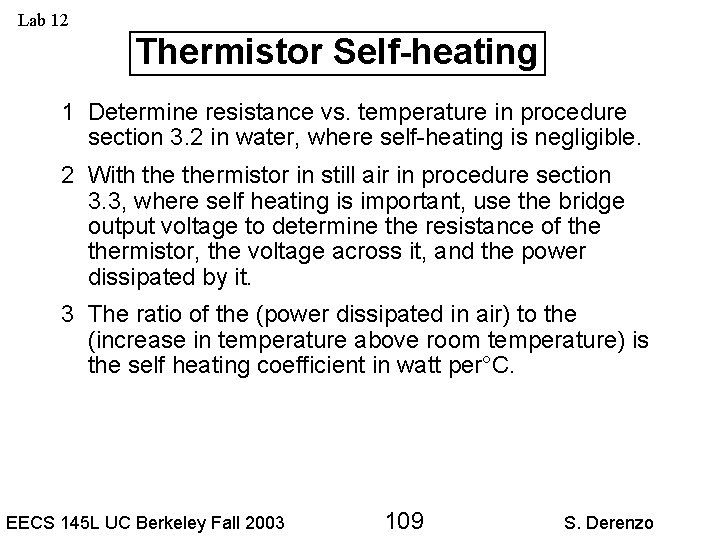
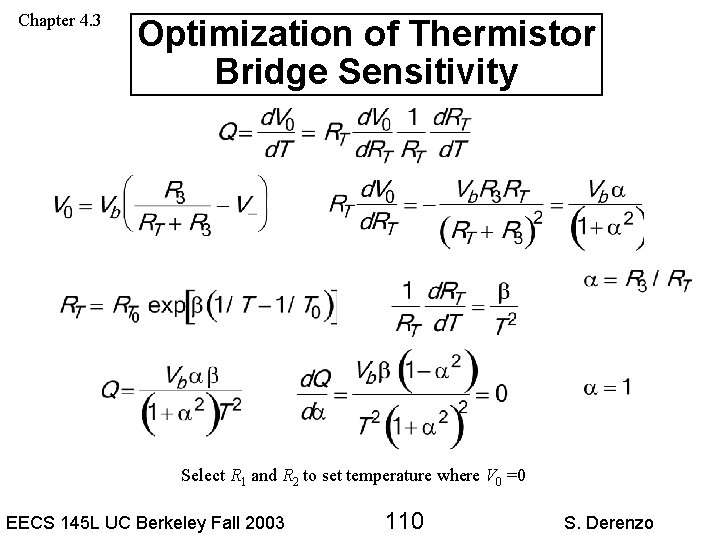
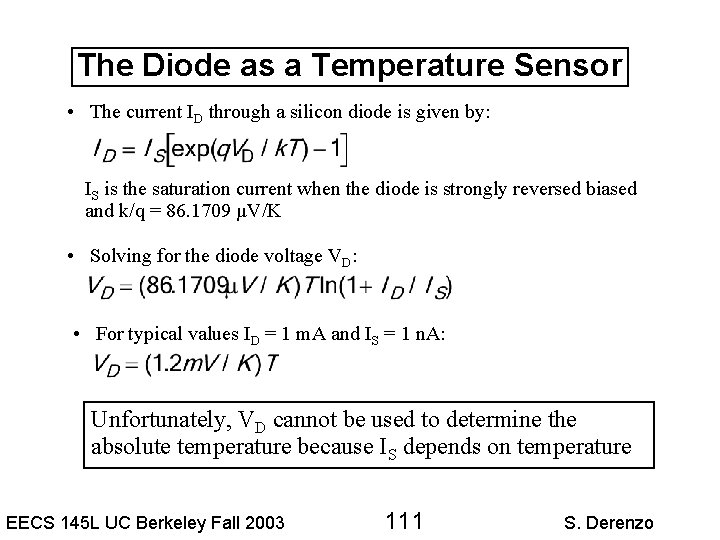
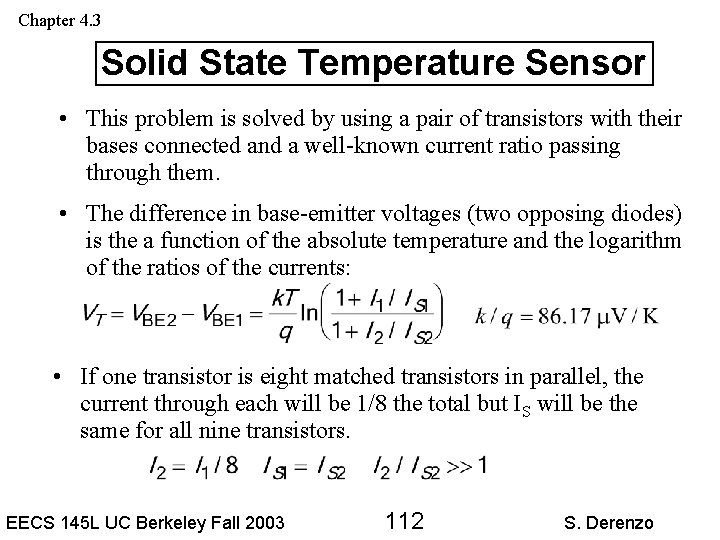
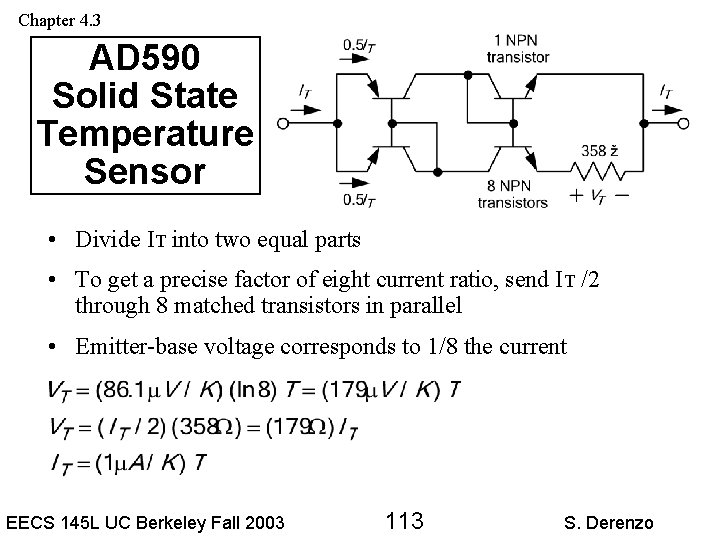
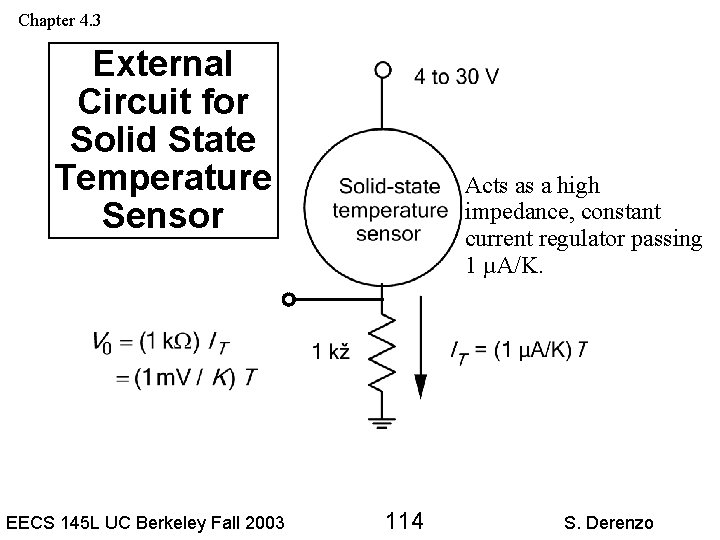
- Slides: 114
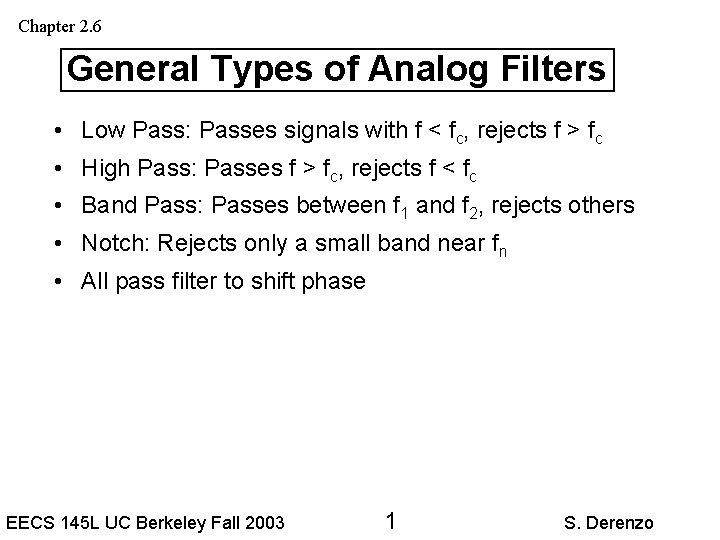
Chapter 2. 6 General Types of Analog Filters • Low Pass: Passes signals with f < fc, rejects f > fc • High Pass: Passes f > fc, rejects f < fc • Band Pass: Passes between f 1 and f 2, rejects others • Notch: Rejects only a small band near fn • All pass filter to shift phase EECS 145 L UC Berkeley Fall 2003 1 S. Derenzo
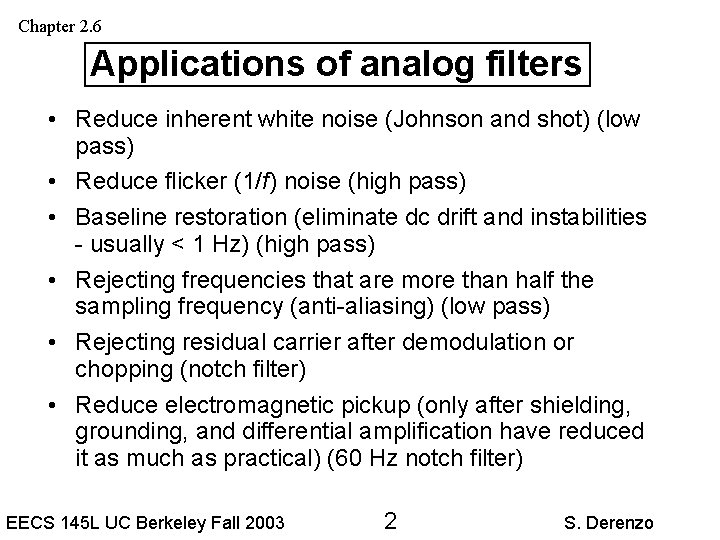
Chapter 2. 6 Applications of analog filters • Reduce inherent white noise (Johnson and shot) (low pass) • Reduce flicker (1/f) noise (high pass) • Baseline restoration (eliminate dc drift and instabilities - usually < 1 Hz) (high pass) • Rejecting frequencies that are more than half the sampling frequency (anti-aliasing) (low pass) • Rejecting residual carrier after demodulation or chopping (notch filter) • Reduce electromagnetic pickup (only after shielding, grounding, and differential amplification have reduced it as much as practical) (60 Hz notch filter) EECS 145 L UC Berkeley Fall 2003 2 S. Derenzo
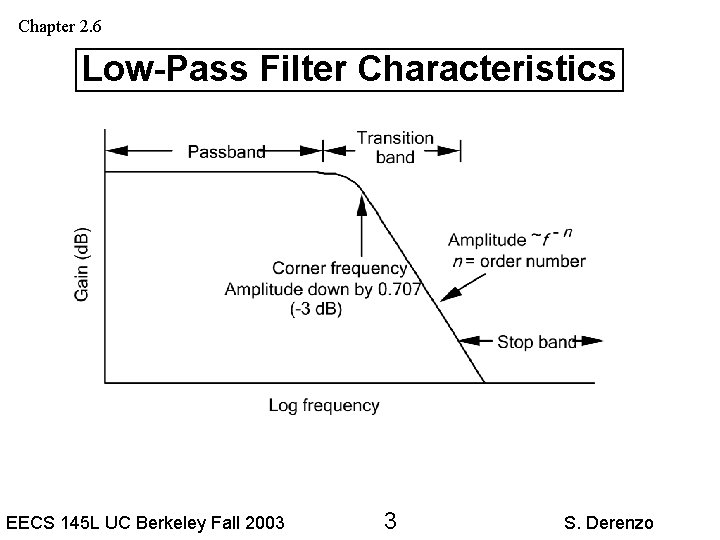
Chapter 2. 6 Low-Pass Filter Characteristics EECS 145 L UC Berkeley Fall 2003 3 S. Derenzo
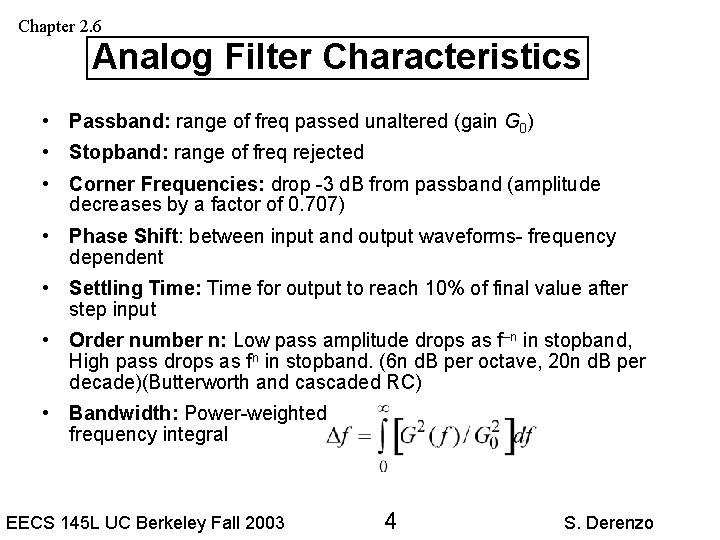
Chapter 2. 6 Analog Filter Characteristics • Passband: range of freq passed unaltered (gain G 0) • Stopband: range of freq rejected • Corner Frequencies: drop -3 d. B from passband (amplitude decreases by a factor of 0. 707) • Phase Shift: between input and output waveforms- frequency dependent • Settling Time: Time for output to reach 10% of final value after step input • Order number n: Low pass amplitude drops as f–n in stopband, High pass drops as fn in stopband. (6 n d. B per octave, 20 n d. B per decade)(Butterworth and cascaded RC) • Bandwidth: Power-weighted frequency integral EECS 145 L UC Berkeley Fall 2003 4 S. Derenzo
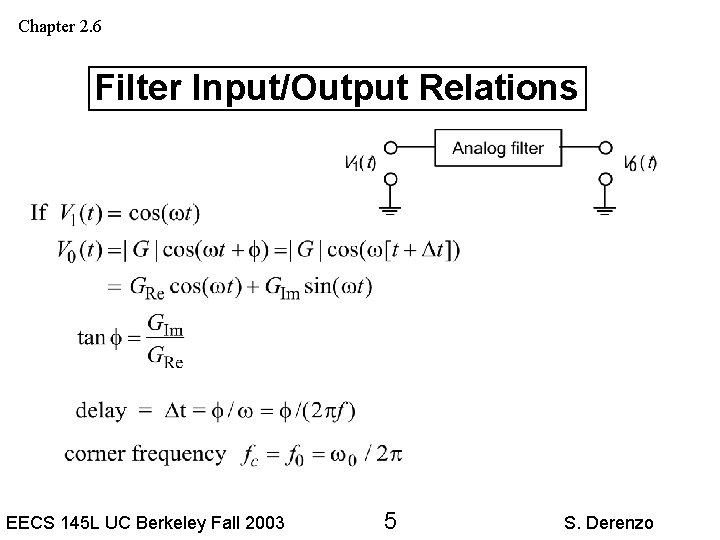
Chapter 2. 6 Filter Input/Output Relations EECS 145 L UC Berkeley Fall 2003 5 S. Derenzo
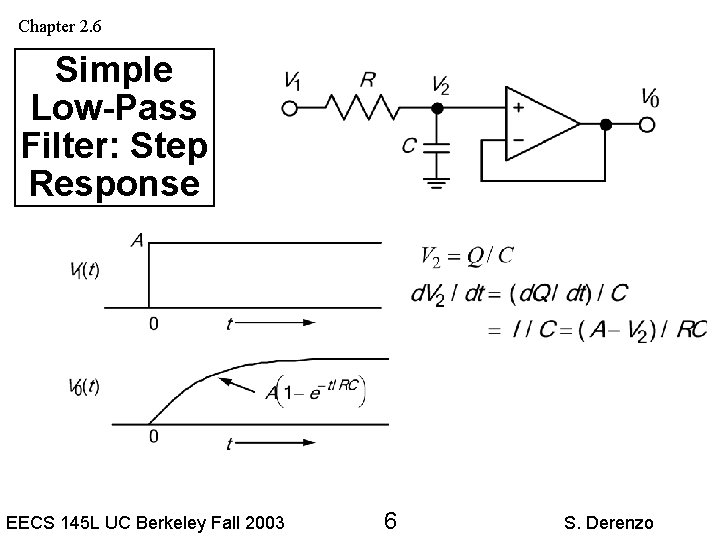
Chapter 2. 6 Simple Low-Pass Filter: Step Response EECS 145 L UC Berkeley Fall 2003 6 S. Derenzo
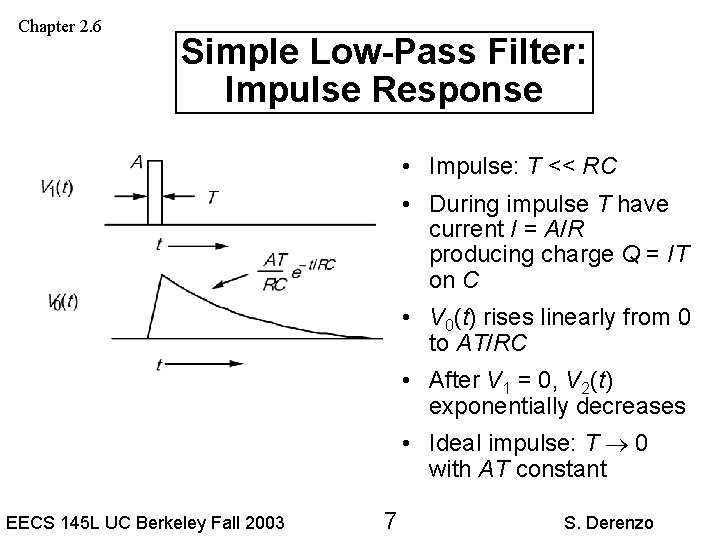
Chapter 2. 6 Simple Low-Pass Filter: Impulse Response • Impulse: T << RC • During impulse T have current I = A/R producing charge Q = IT on C • V 0(t) rises linearly from 0 to AT/RC • After V 1 = 0, V 2(t) exponentially decreases • Ideal impulse: T 0 with AT constant EECS 145 L UC Berkeley Fall 2003 7 S. Derenzo
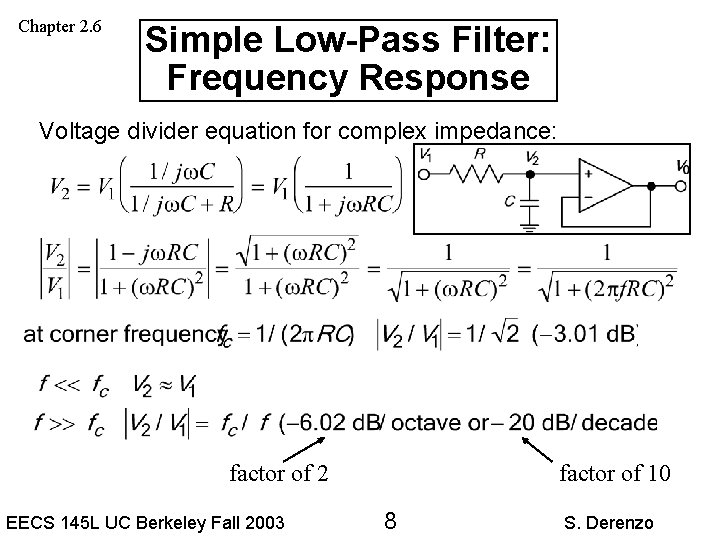
Chapter 2. 6 Simple Low-Pass Filter: Frequency Response Voltage divider equation for complex impedance: factor of 2 EECS 145 L UC Berkeley Fall 2003 factor of 10 8 S. Derenzo
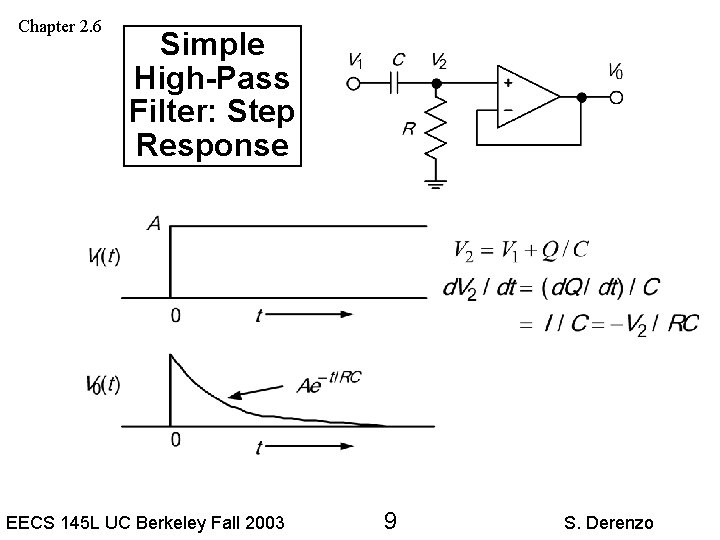
Chapter 2. 6 Simple High-Pass Filter: Step Response EECS 145 L UC Berkeley Fall 2003 9 S. Derenzo
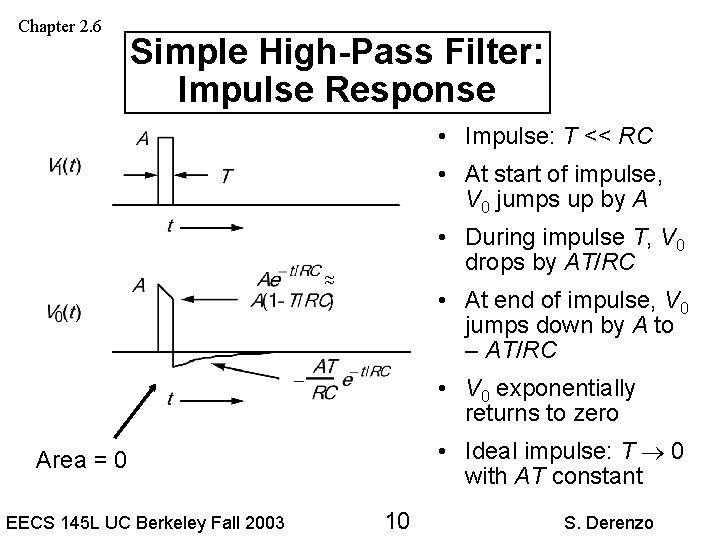
Chapter 2. 6 Simple High-Pass Filter: Impulse Response • Impulse: T << RC • At start of impulse, V 0 jumps up by A • During impulse T, V 0 drops by AT/RC ≈ • At end of impulse, V 0 jumps down by A to – AT/RC • V 0 exponentially returns to zero • Ideal impulse: T 0 with AT constant Area = 0 EECS 145 L UC Berkeley Fall 2003 10 S. Derenzo
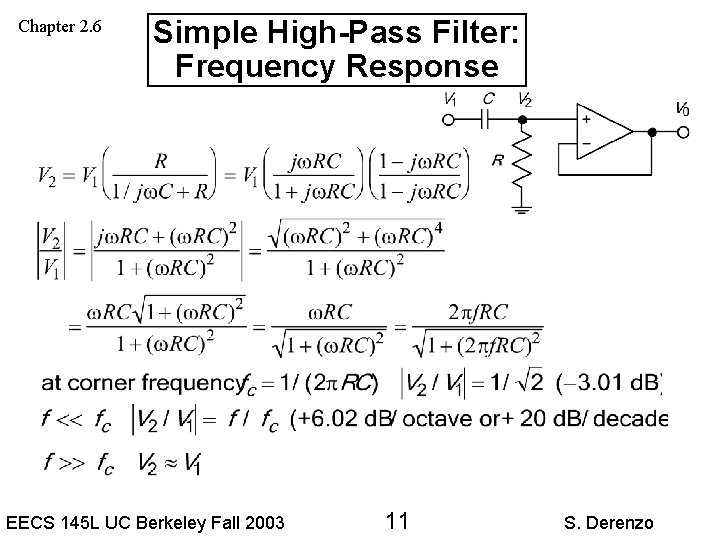
Chapter 2. 6 Simple High-Pass Filter: Frequency Response EECS 145 L UC Berkeley Fall 2003 11 S. Derenzo
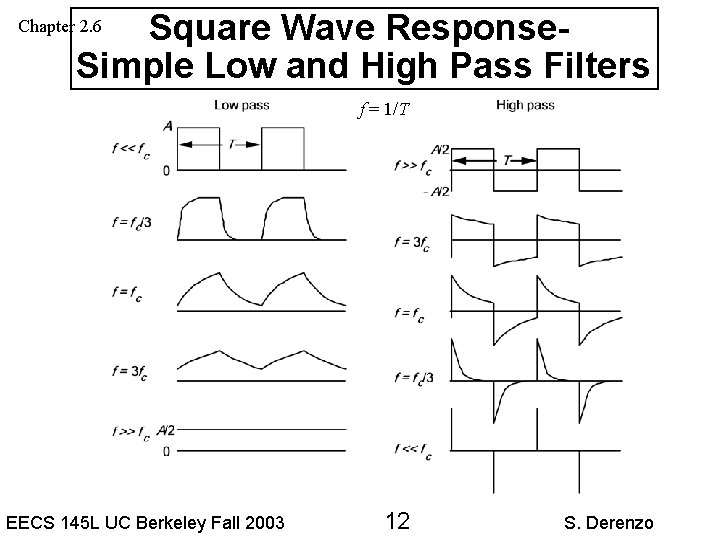
Square Wave Response. Simple Low and High Pass Filters Chapter 2. 6 f = 1/T EECS 145 L UC Berkeley Fall 2003 12 S. Derenzo
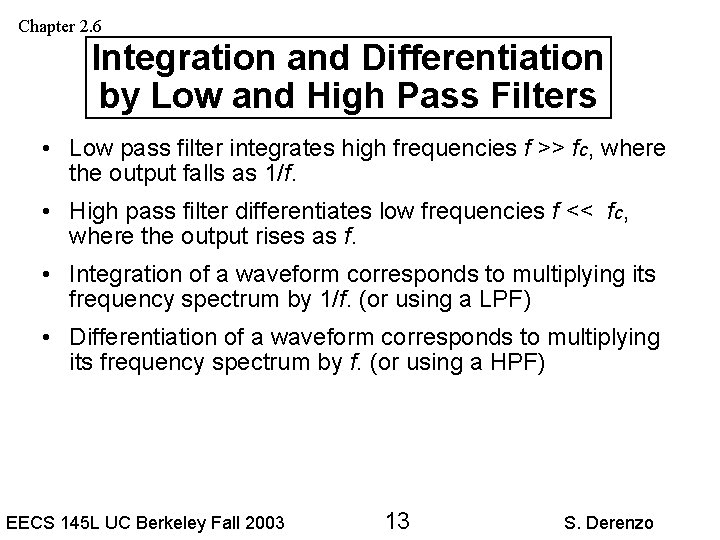
Chapter 2. 6 Integration and Differentiation by Low and High Pass Filters • Low pass filter integrates high frequencies f >> fc, where the output falls as 1/f. • High pass filter differentiates low frequencies f << fc, where the output rises as f. • Integration of a waveform corresponds to multiplying its frequency spectrum by 1/f. (or using a LPF) • Differentiation of a waveform corresponds to multiplying its frequency spectrum by f. (or using a HPF) EECS 145 L UC Berkeley Fall 2003 13 S. Derenzo
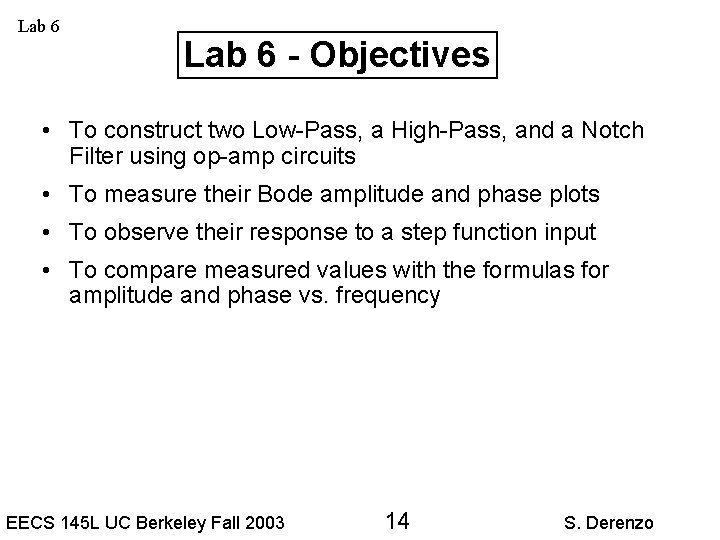
Lab 6 - Objectives • To construct two Low-Pass, a High-Pass, and a Notch Filter using op-amp circuits • To measure their Bode amplitude and phase plots • To observe their response to a step function input • To compare measured values with the formulas for amplitude and phase vs. frequency EECS 145 L UC Berkeley Fall 2003 14 S. Derenzo
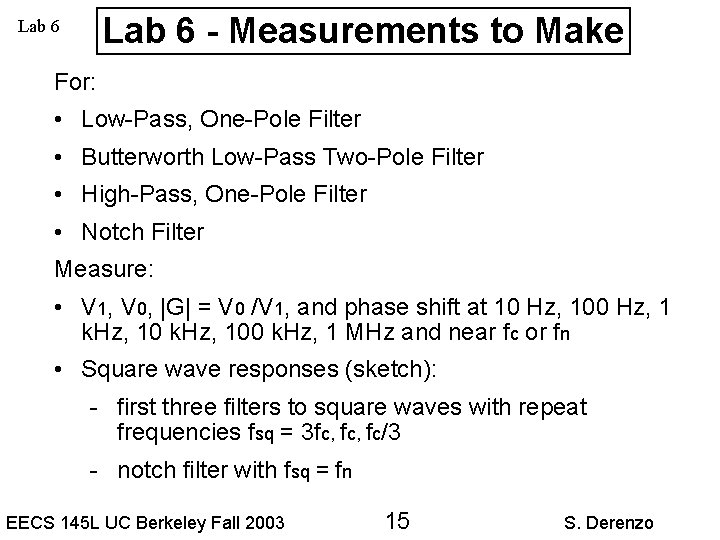
Lab 6 - Measurements to Make Lab 6 For: • Low-Pass, One-Pole Filter • Butterworth Low-Pass Two-Pole Filter • High-Pass, One-Pole Filter • Notch Filter Measure: • V 1, V 0, |G| = V 0 /V 1, and phase shift at 10 Hz, 100 Hz, 1 k. Hz, 100 k. Hz, 1 MHz and near fc or fn • Square wave responses (sketch): - first three filters to square waves with repeat frequencies fsq = 3 fc, fc/3 - notch filter with fsq = fn EECS 145 L UC Berkeley Fall 2003 15 S. Derenzo
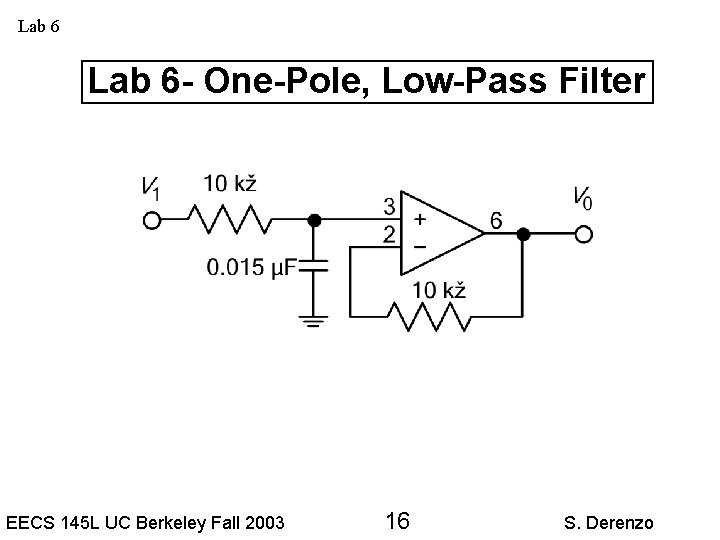
Lab 6 - One-Pole, Low-Pass Filter EECS 145 L UC Berkeley Fall 2003 16 S. Derenzo
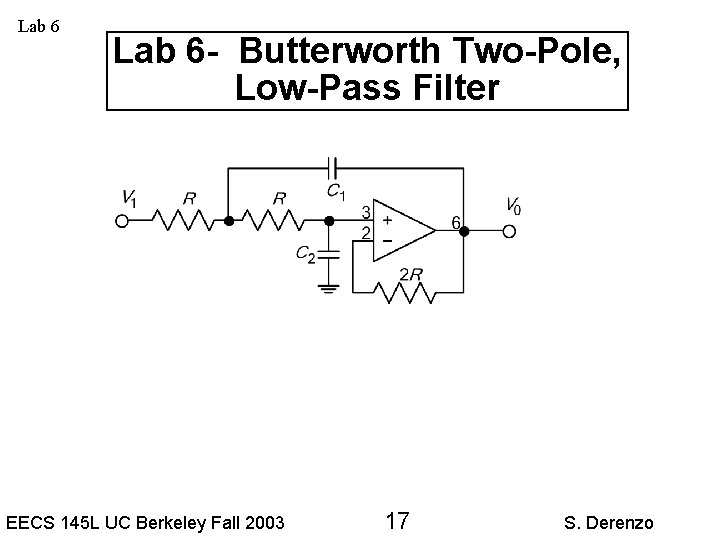
Lab 6 - Butterworth Two-Pole, Low-Pass Filter EECS 145 L UC Berkeley Fall 2003 17 S. Derenzo
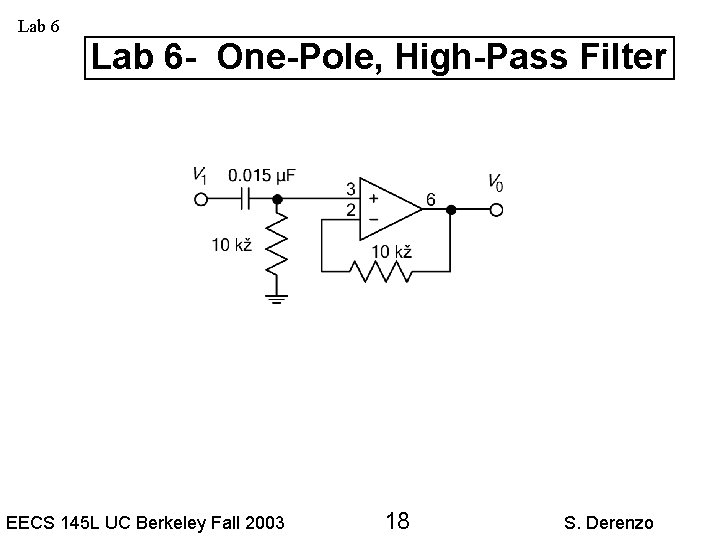
Lab 6 - One-Pole, High-Pass Filter EECS 145 L UC Berkeley Fall 2003 18 S. Derenzo
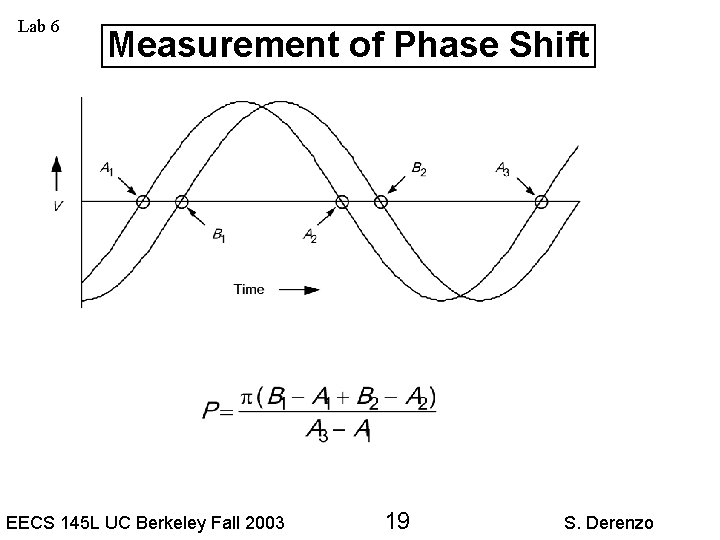
Lab 6 Measurement of Phase Shift EECS 145 L UC Berkeley Fall 2003 19 S. Derenzo
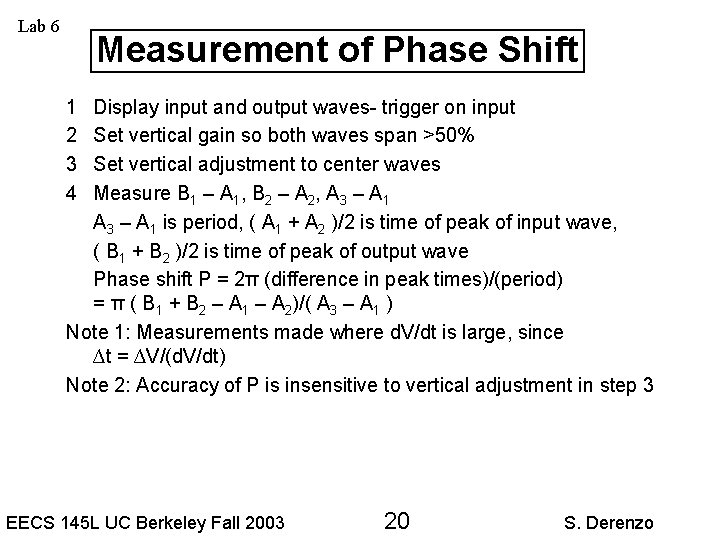
Lab 6 Measurement of Phase Shift 1 2 3 4 Display input and output waves- trigger on input Set vertical gain so both waves span >50% Set vertical adjustment to center waves Measure B 1 – A 1, B 2 – A 2, A 3 – A 1 is period, ( A 1 + A 2 )/2 is time of peak of input wave, ( B 1 + B 2 )/2 is time of peak of output wave Phase shift P = 2π (difference in peak times)/(period) = π ( B 1 + B 2 – A 1 – A 2)/( A 3 – A 1 ) Note 1: Measurements made where d. V/dt is large, since ∆t = ∆V/(d. V/dt) Note 2: Accuracy of P is insensitive to vertical adjustment in step 3 EECS 145 L UC Berkeley Fall 2003 20 S. Derenzo
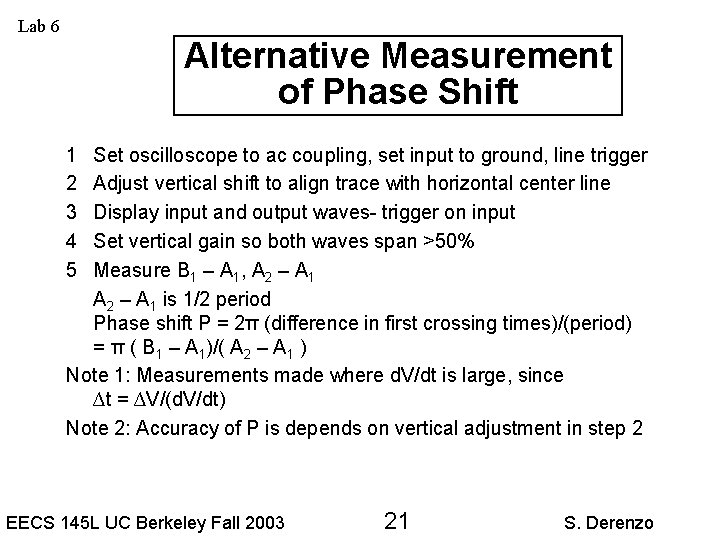
Lab 6 Alternative Measurement of Phase Shift 1 2 3 4 5 Set oscilloscope to ac coupling, set input to ground, line trigger Adjust vertical shift to align trace with horizontal center line Display input and output waves- trigger on input Set vertical gain so both waves span >50% Measure B 1 – A 1, A 2 – A 1 is 1/2 period Phase shift P = 2π (difference in first crossing times)/(period) = π ( B 1 – A 1)/( A 2 – A 1 ) Note 1: Measurements made where d. V/dt is large, since ∆t = ∆V/(d. V/dt) Note 2: Accuracy of P is depends on vertical adjustment in step 2 EECS 145 L UC Berkeley Fall 2003 21 S. Derenzo
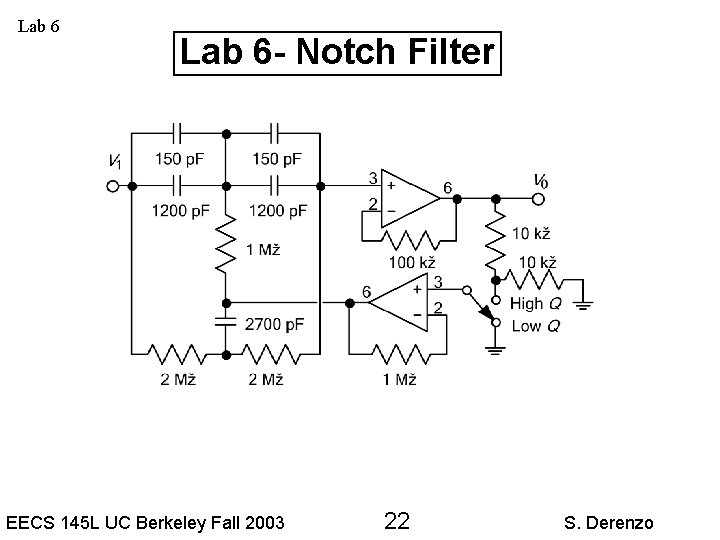
Lab 6 - Notch Filter EECS 145 L UC Berkeley Fall 2003 22 S. Derenzo
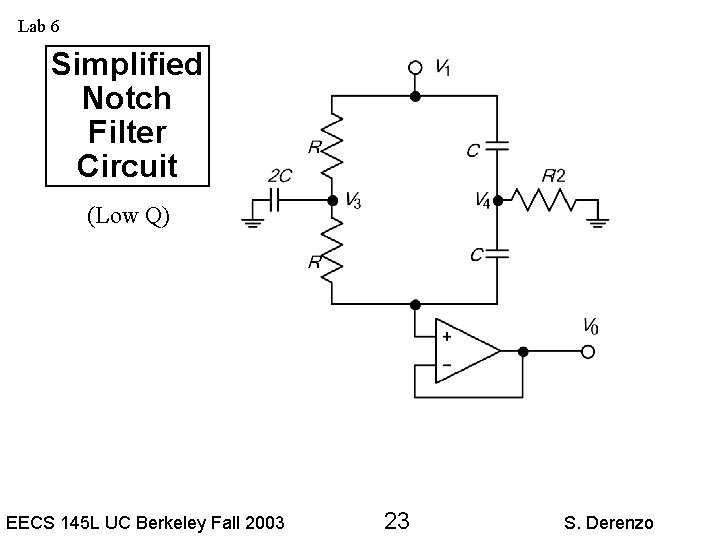
Lab 6 Simplified Notch Filter Circuit (Low Q) EECS 145 L UC Berkeley Fall 2003 23 S. Derenzo
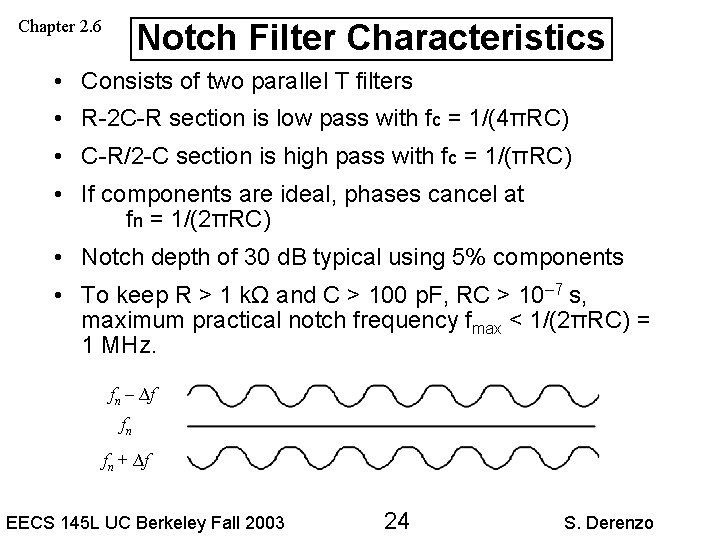
Chapter 2. 6 Notch Filter Characteristics • Consists of two parallel T filters • R-2 C-R section is low pass with fc = 1/(4πRC) • C-R/2 -C section is high pass with fc = 1/(πRC) • If components are ideal, phases cancel at fn = 1/(2πRC) • Notch depth of 30 d. B typical using 5% components • To keep R > 1 kΩ and C > 100 p. F, RC > 10– 7 s, maximum practical notch frequency fmax < 1/(2πRC) = 1 MHz. fn – ∆f fn fn + ∆f EECS 145 L UC Berkeley Fall 2003 24 S. Derenzo
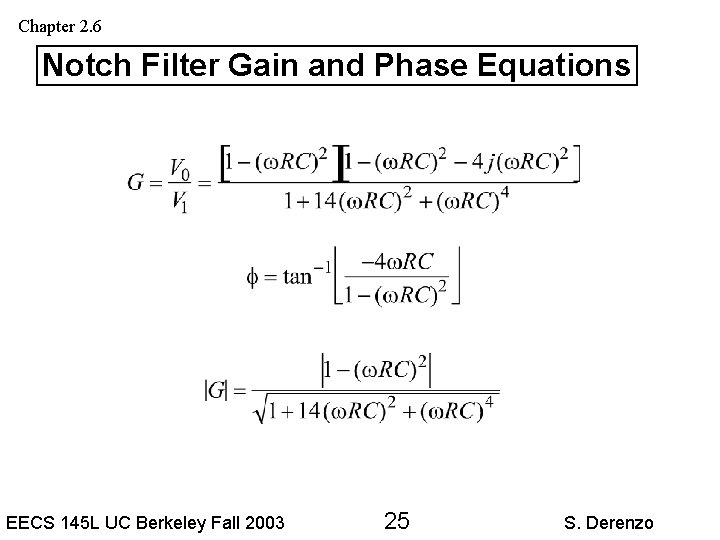
Chapter 2. 6 Notch Filter Gain and Phase Equations EECS 145 L UC Berkeley Fall 2003 25 S. Derenzo
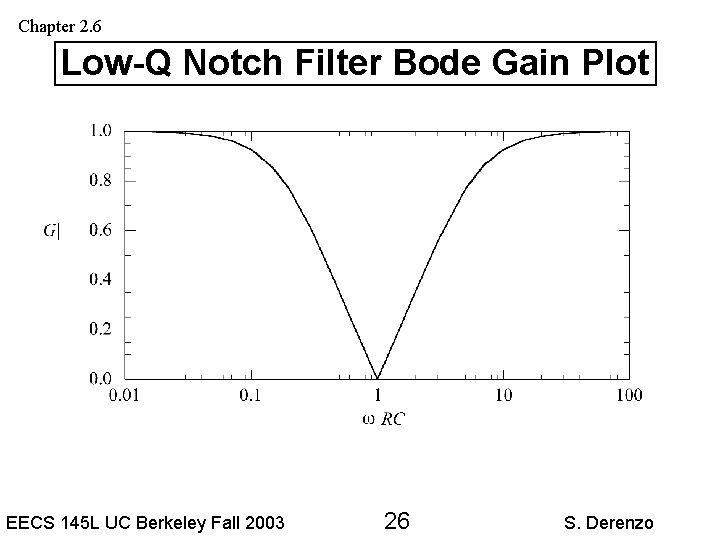
Chapter 2. 6 Low-Q Notch Filter Bode Gain Plot EECS 145 L UC Berkeley Fall 2003 26 S. Derenzo
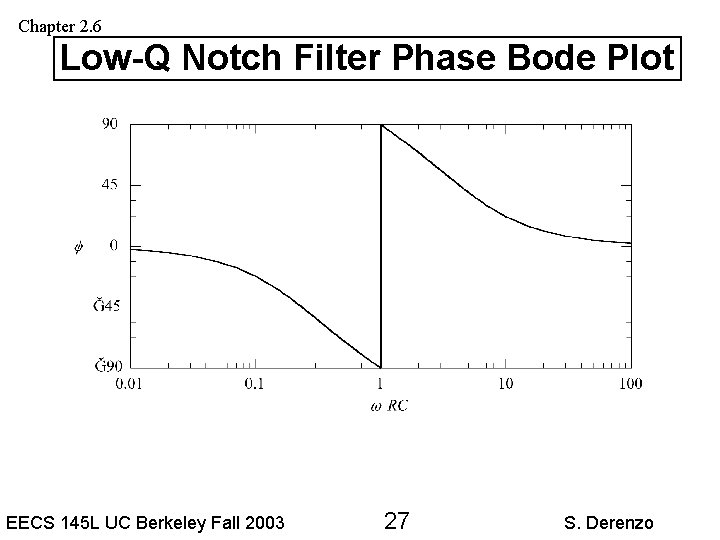
Chapter 2. 6 Low-Q Notch Filter Phase Bode Plot EECS 145 L UC Berkeley Fall 2003 27 S. Derenzo
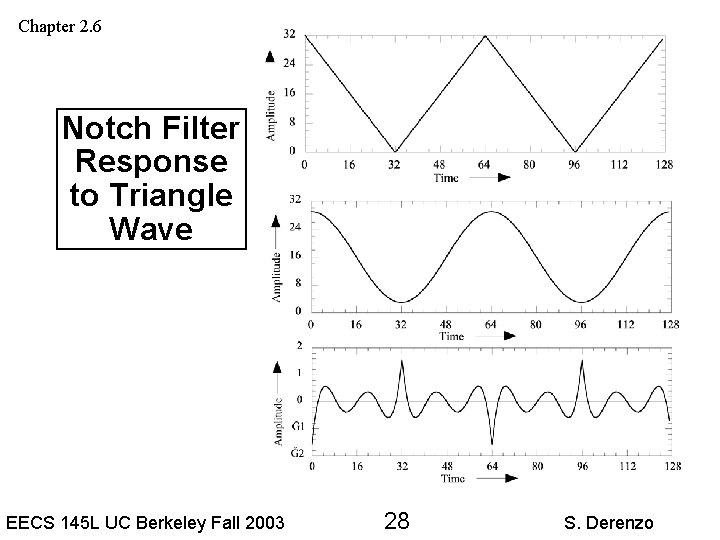
Chapter 2. 6 Notch Filter Response to Triangle Wave EECS 145 L UC Berkeley Fall 2003 28 S. Derenzo
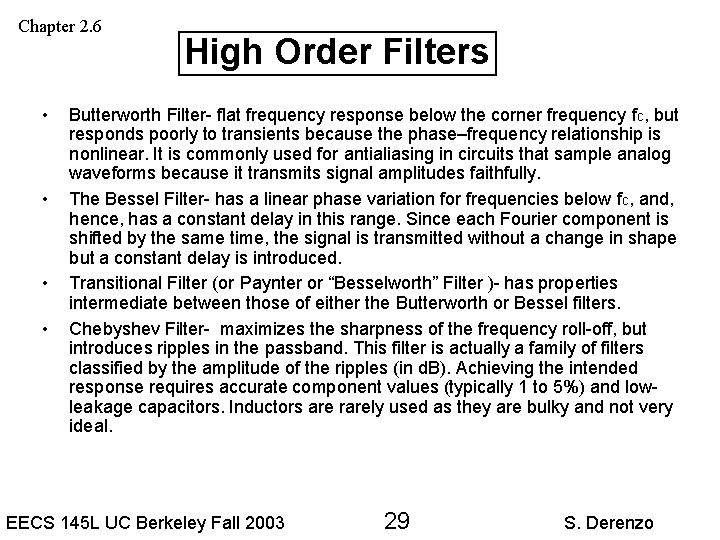
Chapter 2. 6 • • High Order Filters Butterworth Filter- flat frequency response below the corner frequency fc, but responds poorly to transients because the phase–frequency relationship is nonlinear. It is commonly used for antialiasing in circuits that sample analog waveforms because it transmits signal amplitudes faithfully. The Bessel Filter- has a linear phase variation for frequencies below fc, and, hence, has a constant delay in this range. Since each Fourier component is shifted by the same time, the signal is transmitted without a change in shape but a constant delay is introduced. Transitional Filter (or Paynter or “Besselworth” Filter )- has properties intermediate between those of either the Butterworth or Bessel filters. Chebyshev Filter- maximizes the sharpness of the frequency roll-off, but introduces ripples in the passband. This filter is actually a family of filters classified by the amplitude of the ripples (in d. B). Achieving the intended response requires accurate component values (typically 1 to 5%) and lowleakage capacitors. Inductors are rarely used as they are bulky and not very ideal. EECS 145 L UC Berkeley Fall 2003 29 S. Derenzo
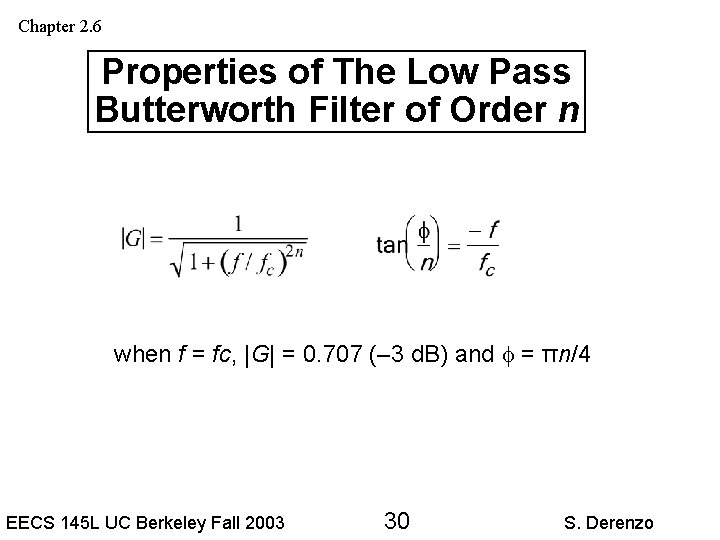
Chapter 2. 6 Properties of The Low Pass Butterworth Filter of Order n when f = fc, |G| = 0. 707 (– 3 d. B) and = πn/4 EECS 145 L UC Berkeley Fall 2003 30 S. Derenzo
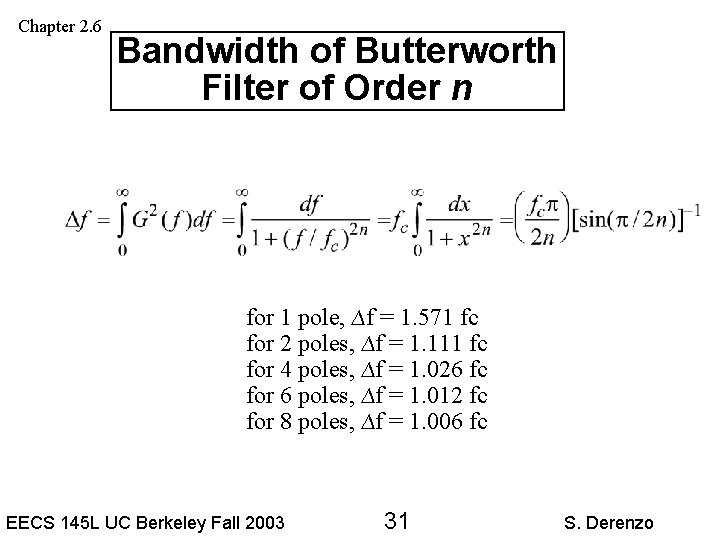
Chapter 2. 6 Bandwidth of Butterworth Filter of Order n for 1 pole, ∆f = 1. 571 fc for 2 poles, ∆f = 1. 111 fc for 4 poles, ∆f = 1. 026 fc for 6 poles, ∆f = 1. 012 fc for 8 poles, ∆f = 1. 006 fc EECS 145 L UC Berkeley Fall 2003 31 S. Derenzo
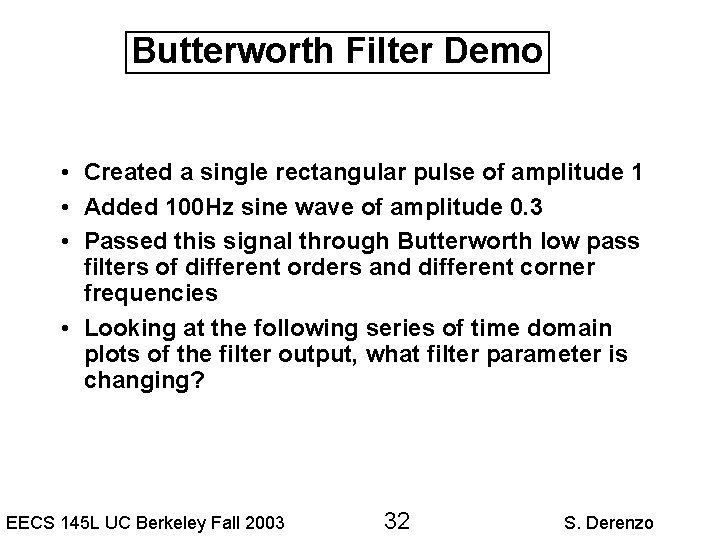
Butterworth Filter Demo • Created a single rectangular pulse of amplitude 1 • Added 100 Hz sine wave of amplitude 0. 3 • Passed this signal through Butterworth low pass filters of different orders and different corner frequencies • Looking at the following series of time domain plots of the filter output, what filter parameter is changing? EECS 145 L UC Berkeley Fall 2003 32 S. Derenzo
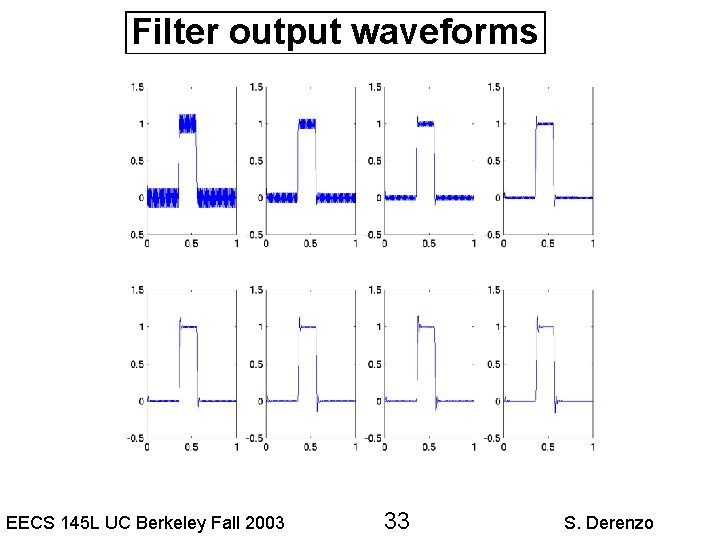
Filter output waveforms EECS 145 L UC Berkeley Fall 2003 33 S. Derenzo
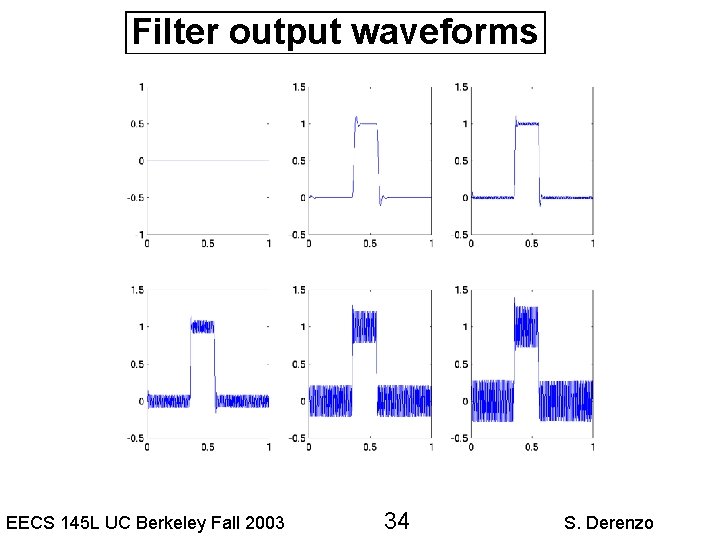
Filter output waveforms EECS 145 L UC Berkeley Fall 2003 34 S. Derenzo
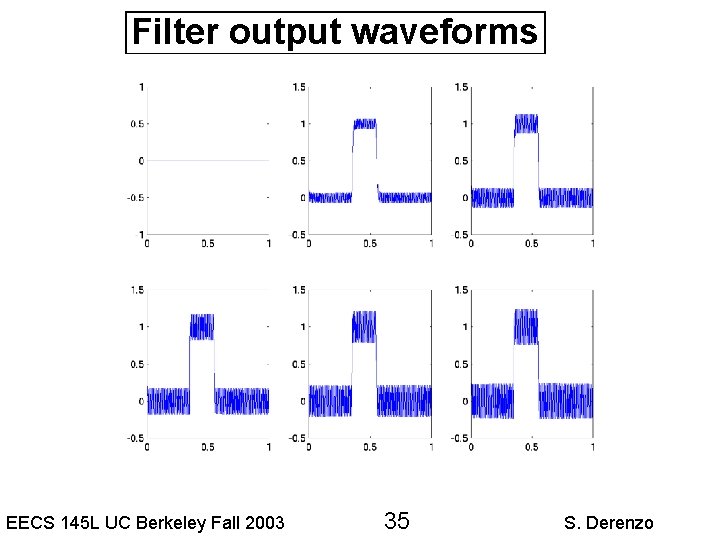
Filter output waveforms EECS 145 L UC Berkeley Fall 2003 35 S. Derenzo
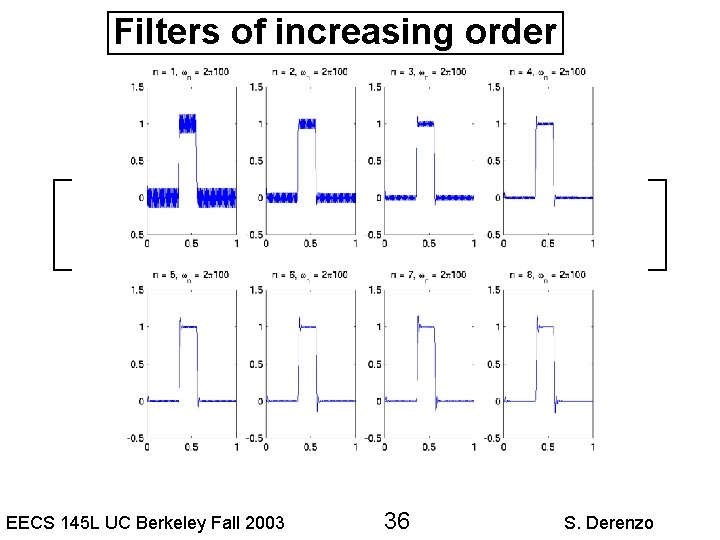
Filters of increasing order EECS 145 L UC Berkeley Fall 2003 36 S. Derenzo
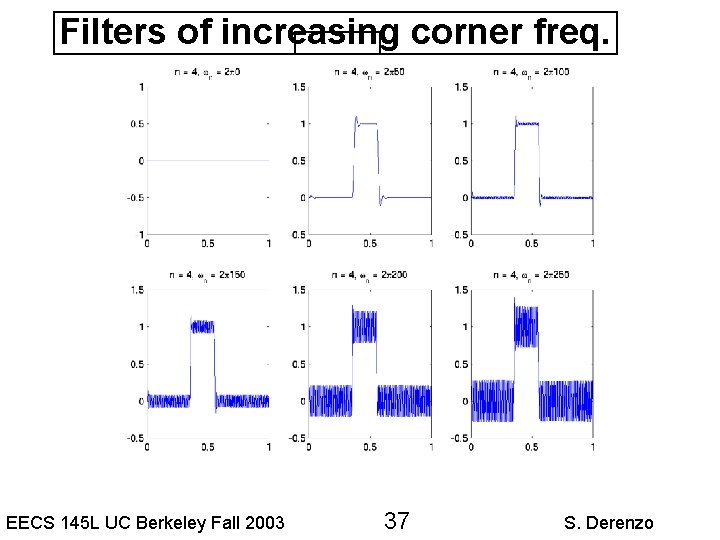
Filters of increasing corner freq. EECS 145 L UC Berkeley Fall 2003 37 S. Derenzo
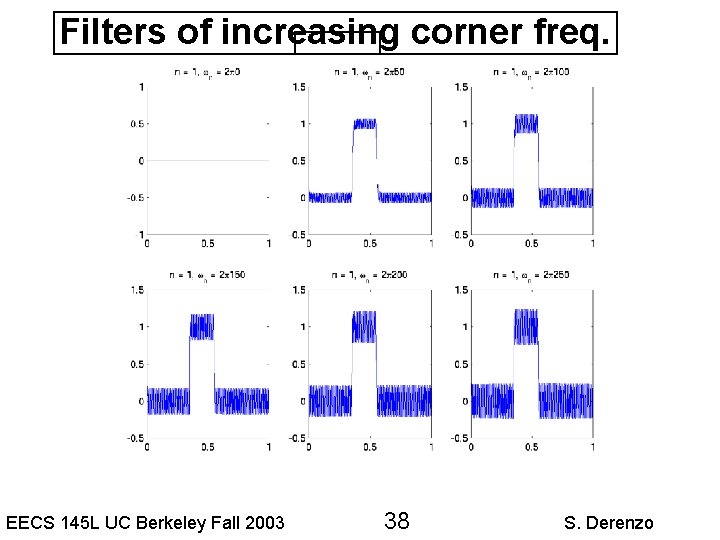
Filters of increasing corner freq. EECS 145 L UC Berkeley Fall 2003 38 S. Derenzo
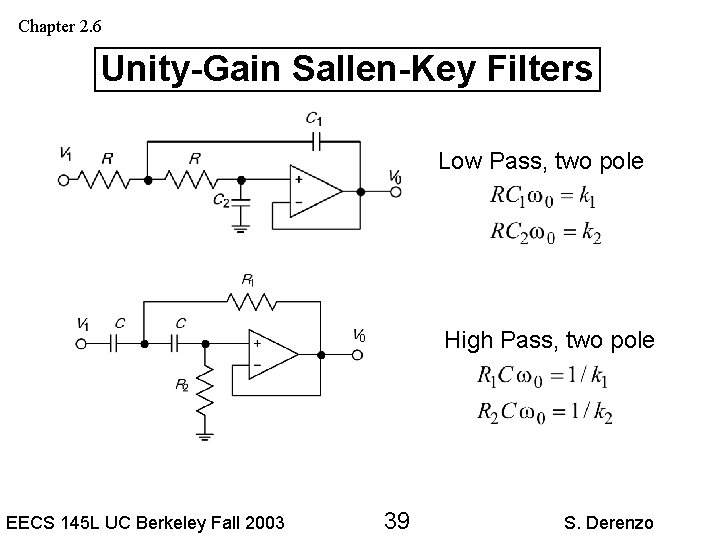
Chapter 2. 6 Unity-Gain Sallen-Key Filters Low Pass, two pole High Pass, two pole EECS 145 L UC Berkeley Fall 2003 39 S. Derenzo
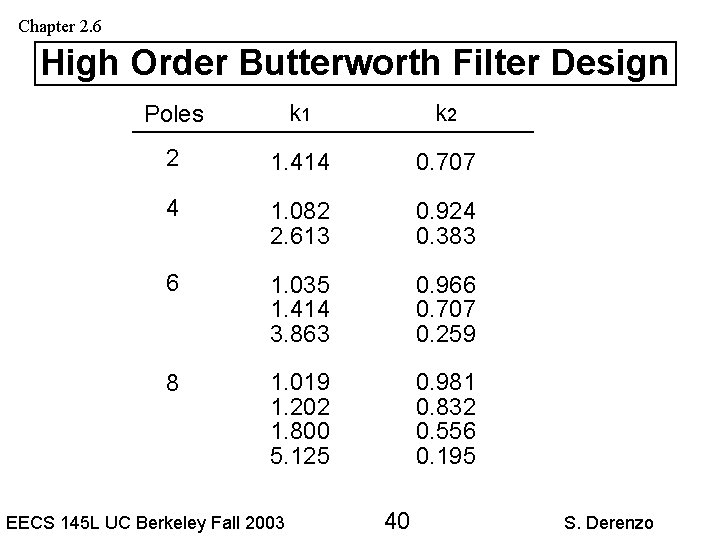
Chapter 2. 6 High Order Butterworth Filter Design Poles k 1 k 2 2 1. 414 0. 707 4 1. 082 2. 613 0. 924 0. 383 6 1. 035 1. 414 3. 863 0. 966 0. 707 0. 259 8 1. 019 1. 202 1. 800 5. 125 0. 981 0. 832 0. 556 0. 195 EECS 145 L UC Berkeley Fall 2003 40 S. Derenzo
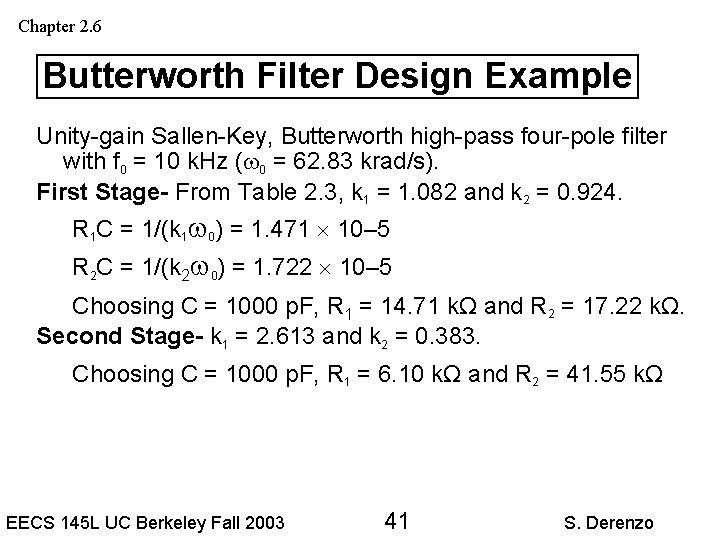
Chapter 2. 6 Butterworth Filter Design Example Unity-gain Sallen-Key, Butterworth high-pass four-pole filter with f 0 = 10 k. Hz ( 0 = 62. 83 krad/s). First Stage- From Table 2. 3, k 1 = 1. 082 and k 2 = 0. 924. R 1 C = 1/(k 1 0) = 1. 471 10– 5 R 2 C = 1/(k 2 0) = 1. 722 10– 5 Choosing C = 1000 p. F, R 1 = 14. 71 kΩ and R 2 = 17. 22 kΩ. Second Stage- k 1 = 2. 613 and k 2 = 0. 383. Choosing C = 1000 p. F, R 1 = 6. 10 kΩ and R 2 = 41. 55 kΩ EECS 145 L UC Berkeley Fall 2003 41 S. Derenzo
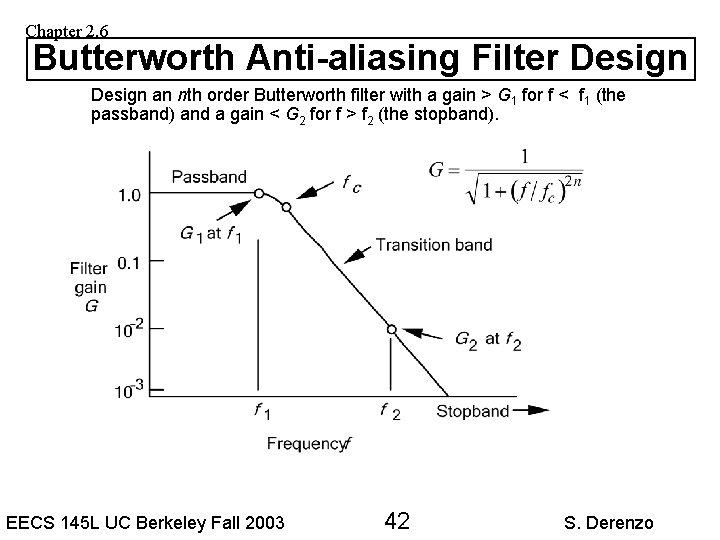
Chapter 2. 6 Butterworth Anti-aliasing Filter Design an nth order Butterworth filter with a gain > G 1 for f < f 1 (the passband) and a gain < G 2 for f > f 2 (the stopband). EECS 145 L UC Berkeley Fall 2003 42 S. Derenzo
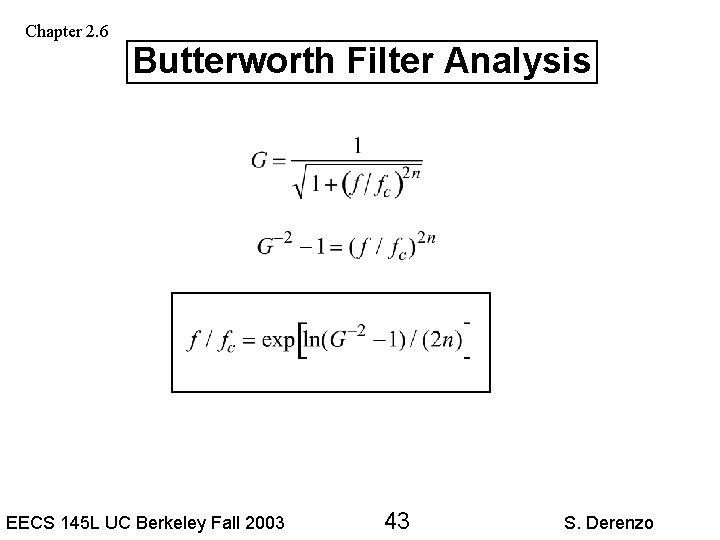
Chapter 2. 6 Butterworth Filter Analysis EECS 145 L UC Berkeley Fall 2003 43 S. Derenzo
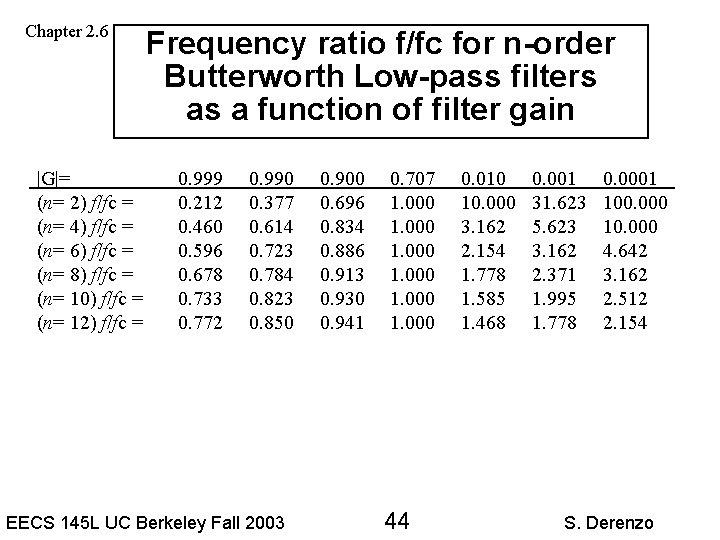
Chapter 2. 6 |G|= (n= 2) f/fc = (n= 4) f/fc = (n= 6) f/fc = (n= 8) f/fc = (n= 10) f/fc = (n= 12) f/fc = Frequency ratio f/fc for n-order Butterworth Low-pass filters as a function of filter gain 0. 999 0. 212 0. 460 0. 596 0. 678 0. 733 0. 772 0. 990 0. 377 0. 614 0. 723 0. 784 0. 823 0. 850 EECS 145 L UC Berkeley Fall 2003 0. 900 0. 696 0. 834 0. 886 0. 913 0. 930 0. 941 0. 707 1. 000 44 0. 010 10. 000 3. 162 2. 154 1. 778 1. 585 1. 468 0. 001 31. 623 5. 623 3. 162 2. 371 1. 995 1. 778 0. 0001 100. 000 10. 000 4. 642 3. 162 2. 512 2. 154 S. Derenzo
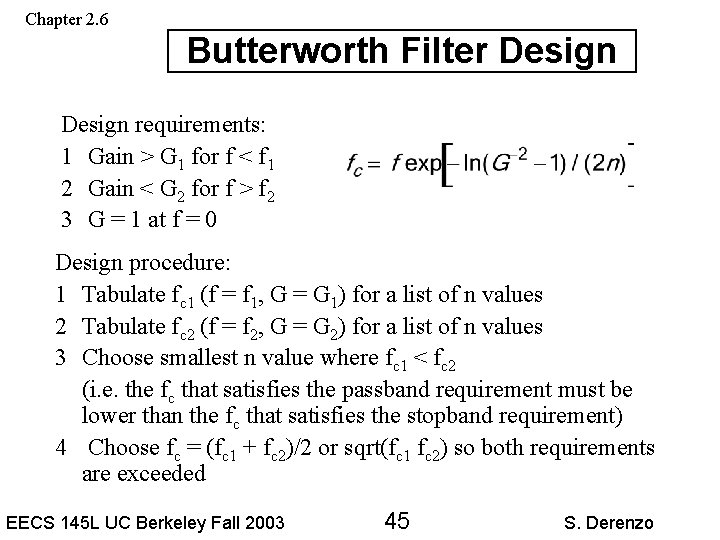
Chapter 2. 6 Butterworth Filter Design requirements: 1 Gain > G 1 for f < f 1 2 Gain < G 2 for f > f 2 3 G = 1 at f = 0 Design procedure: 1 Tabulate fc 1 (f = f 1, G = G 1) for a list of n values 2 Tabulate fc 2 (f = f 2, G = G 2) for a list of n values 3 Choose smallest n value where fc 1 < fc 2 (i. e. the fc that satisfies the passband requirement must be lower than the fc that satisfies the stopband requirement) 4 Choose fc = (fc 1 + fc 2)/2 or sqrt(fc 1 fc 2) so both requirements are exceeded EECS 145 L UC Berkeley Fall 2003 45 S. Derenzo
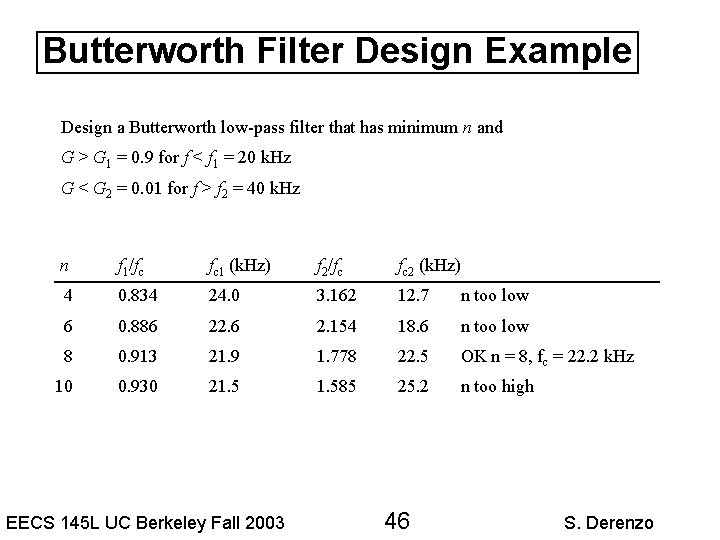
Butterworth Filter Design Example Design a Butterworth low-pass filter that has minimum n and G > G 1 = 0. 9 for f < f 1 = 20 k. Hz G < G 2 = 0. 01 for f > f 2 = 40 k. Hz n f 1/fc fc 1 (k. Hz) f 2/fc fc 2 (k. Hz) 4 0. 834 24. 0 3. 162 12. 7 n too low 6 0. 886 22. 6 2. 154 18. 6 n too low 8 0. 913 21. 9 1. 778 22. 5 OK n = 8, fc = 22. 2 k. Hz 10 0. 930 21. 585 25. 2 n too high EECS 145 L UC Berkeley Fall 2003 46 S. Derenzo
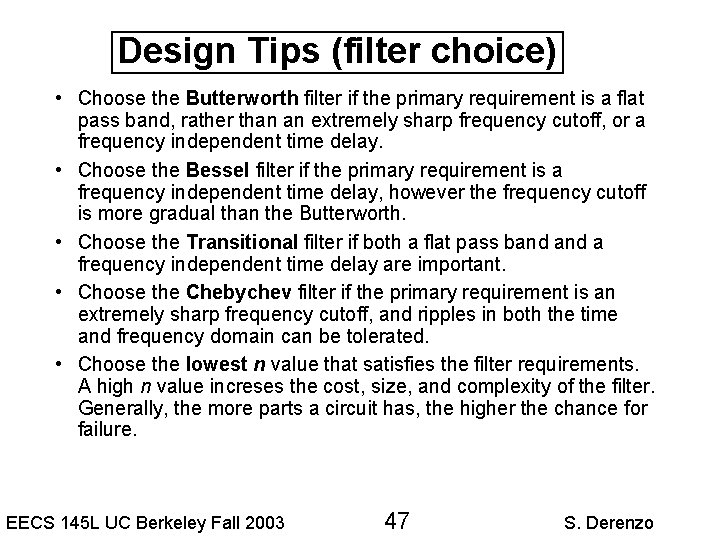
Design Tips (filter choice) • Choose the Butterworth filter if the primary requirement is a flat pass band, rather than an extremely sharp frequency cutoff, or a frequency independent time delay. • Choose the Bessel filter if the primary requirement is a frequency independent time delay, however the frequency cutoff is more gradual than the Butterworth. • Choose the Transitional filter if both a flat pass band a frequency independent time delay are important. • Choose the Chebychev filter if the primary requirement is an extremely sharp frequency cutoff, and ripples in both the time and frequency domain can be tolerated. • Choose the lowest n value that satisfies the filter requirements. A high n value increses the cost, size, and complexity of the filter. Generally, the more parts a circuit has, the higher the chance for failure. EECS 145 L UC Berkeley Fall 2003 47 S. Derenzo
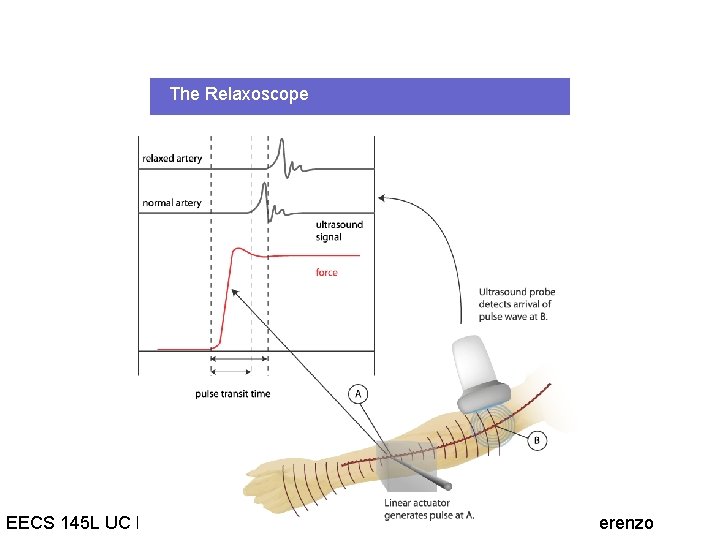
The Relaxoscope EECS 145 L UC Berkeley Fall 2003 48 S. Derenzo
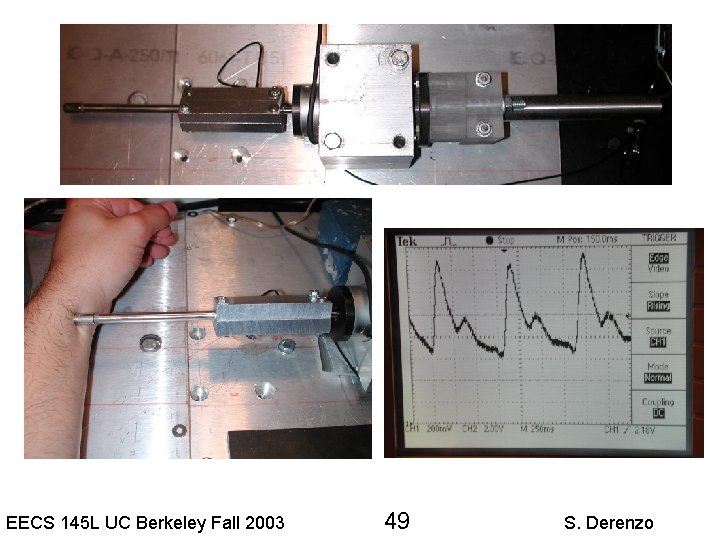
EECS 145 L UC Berkeley Fall 2003 49 S. Derenzo
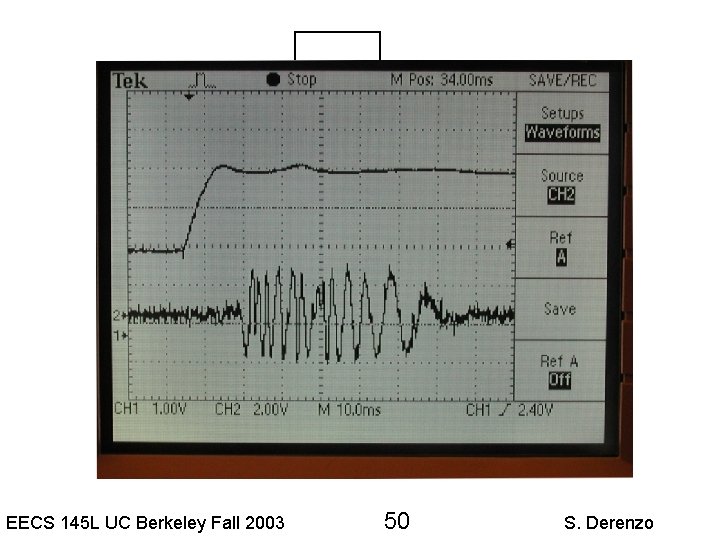
EECS 145 L UC Berkeley Fall 2003 50 S. Derenzo
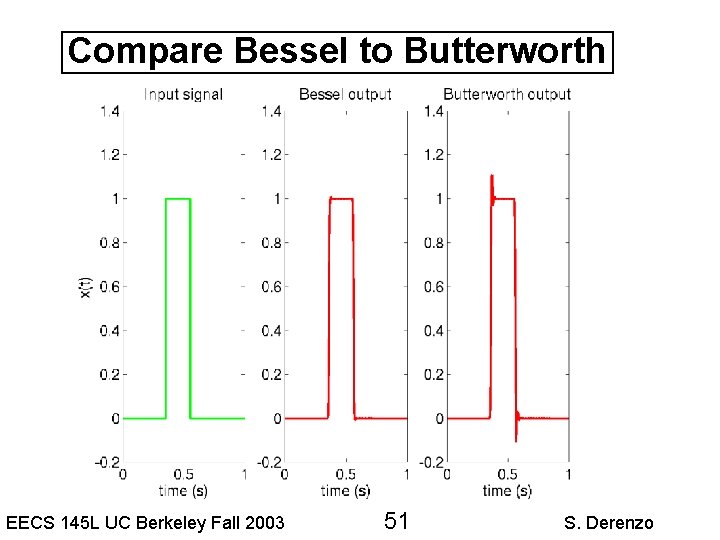
Compare Bessel to Butterworth EECS 145 L UC Berkeley Fall 2003 51 S. Derenzo
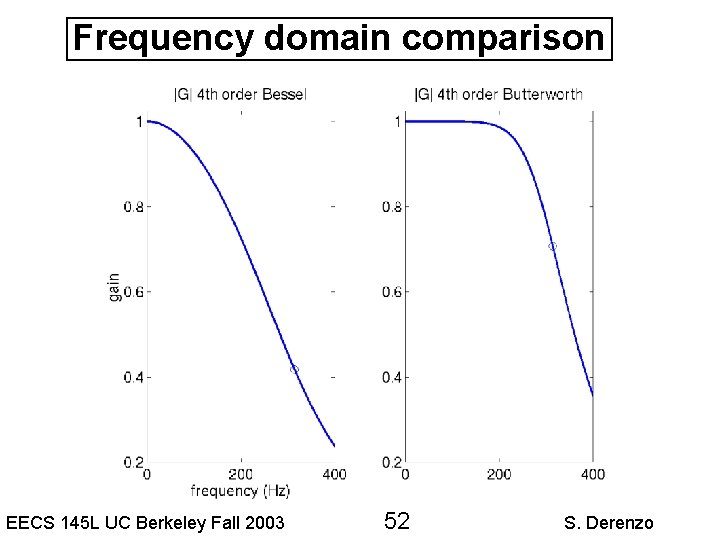
Frequency domain comparison EECS 145 L UC Berkeley Fall 2003 52 S. Derenzo
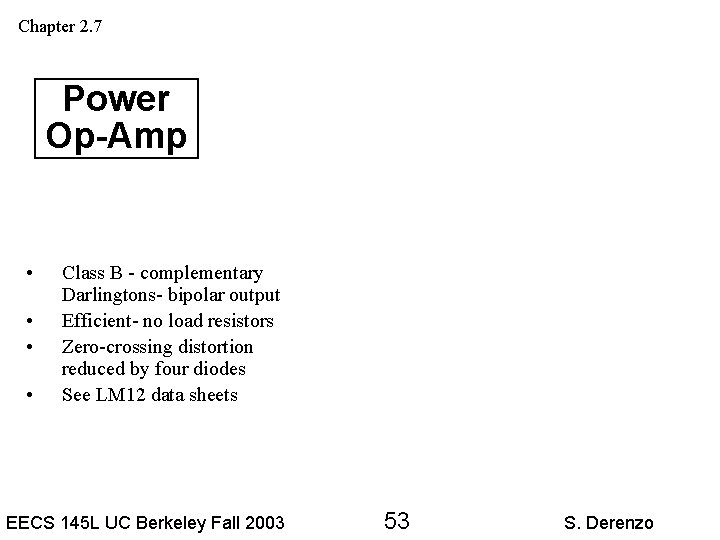
Chapter 2. 7 Power Op-Amp • • Class B - complementary Darlingtons- bipolar output Efficient- no load resistors Zero-crossing distortion reduced by four diodes See LM 12 data sheets EECS 145 L UC Berkeley Fall 2003 53 S. Derenzo
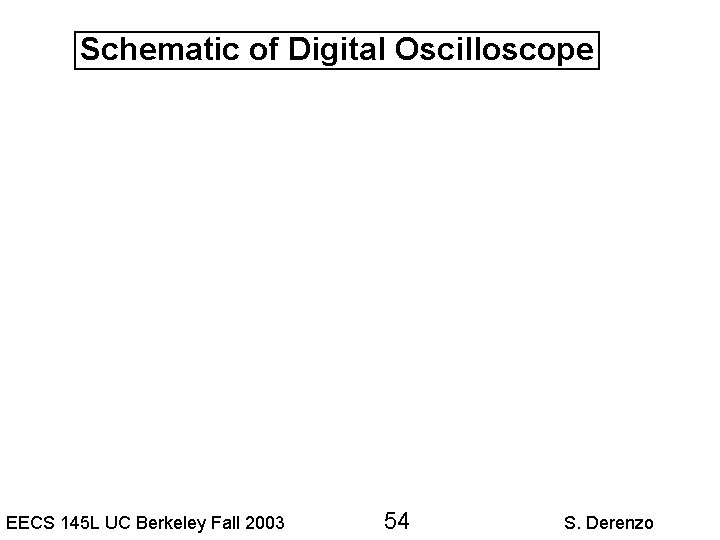
Schematic of Digital Oscilloscope EECS 145 L UC Berkeley Fall 2003 54 S. Derenzo
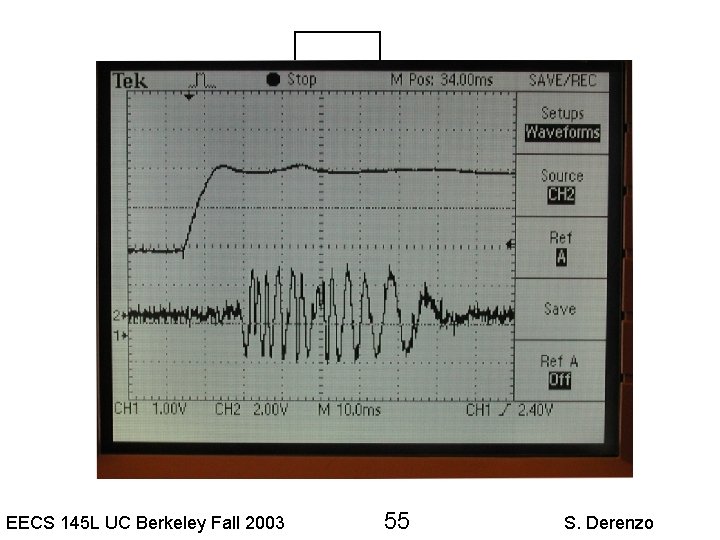
EECS 145 L UC Berkeley Fall 2003 55 S. Derenzo
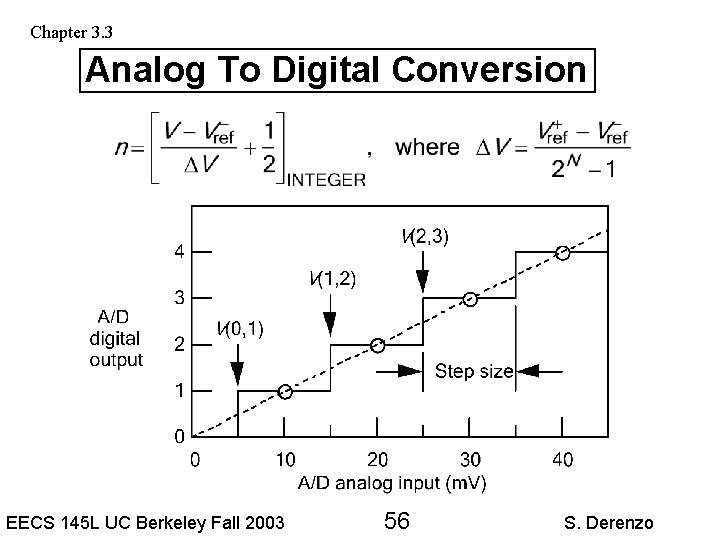
Chapter 3. 3 Analog To Digital Conversion EECS 145 L UC Berkeley Fall 2003 56 S. Derenzo
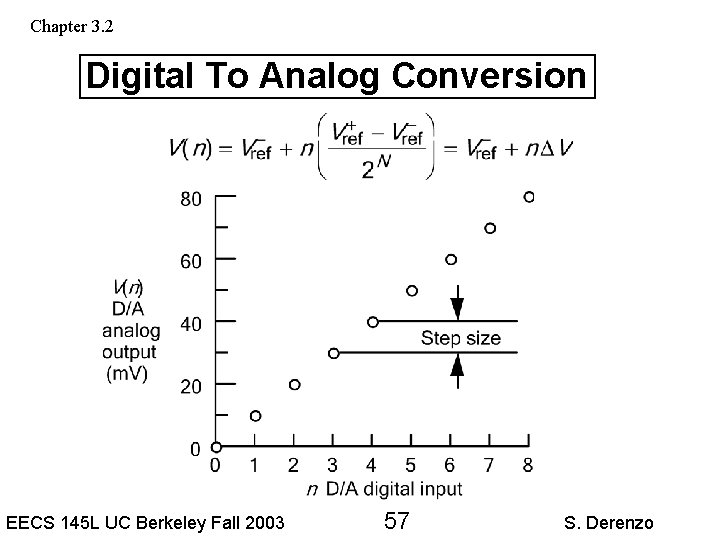
Chapter 3. 2 Digital To Analog Conversion EECS 145 L UC Berkeley Fall 2003 57 S. Derenzo
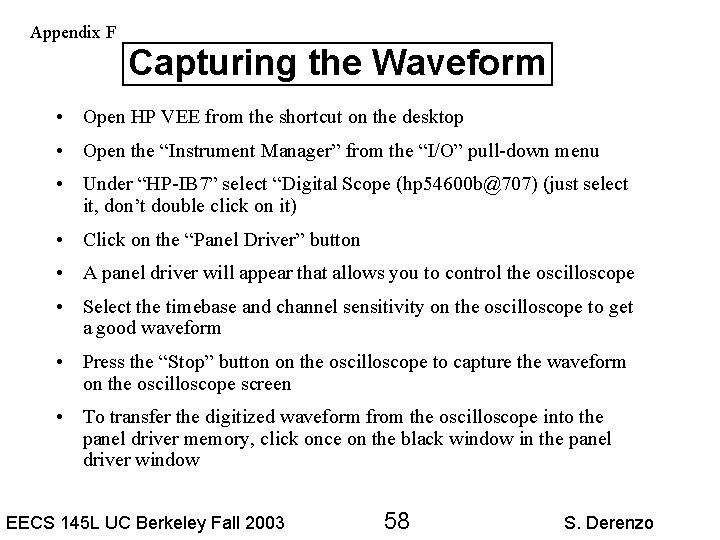
Appendix F Capturing the Waveform • Open HP VEE from the shortcut on the desktop • Open the “Instrument Manager” from the “I/O” pull-down menu • Under “HP-IB 7” select “Digital Scope (hp 54600 b@707) (just select it, don’t double click on it) • Click on the “Panel Driver” button • A panel driver will appear that allows you to control the oscilloscope • Select the timebase and channel sensitivity on the oscilloscope to get a good waveform • Press the “Stop” button on the oscilloscope to capture the waveform on the oscilloscope screen • To transfer the digitized waveform from the oscilloscope into the panel driver memory, click once on the black window in the panel driver window EECS 145 L UC Berkeley Fall 2003 58 S. Derenzo
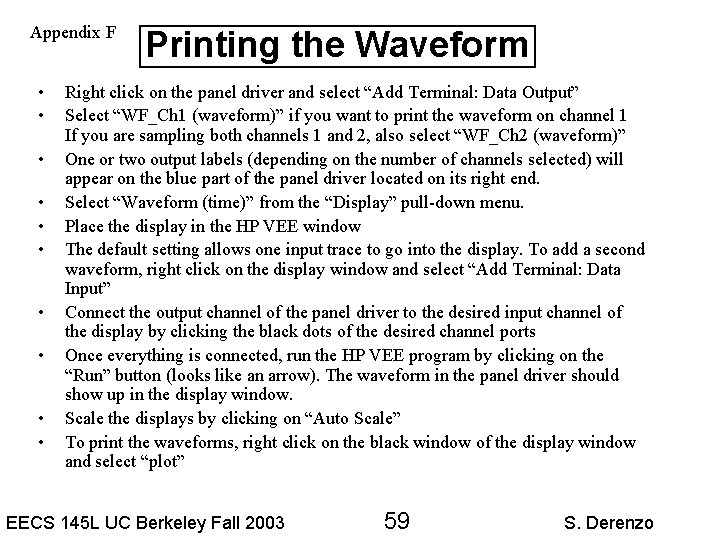
Appendix F • • • Printing the Waveform Right click on the panel driver and select “Add Terminal: Data Output” Select “WF_Ch 1 (waveform)” if you want to print the waveform on channel 1 If you are sampling both channels 1 and 2, also select “WF_Ch 2 (waveform)” One or two output labels (depending on the number of channels selected) will appear on the blue part of the panel driver located on its right end. Select “Waveform (time)” from the “Display” pull-down menu. Place the display in the HP VEE window The default setting allows one input trace to go into the display. To add a second waveform, right click on the display window and select “Add Terminal: Data Input” Connect the output channel of the panel driver to the desired input channel of the display by clicking the black dots of the desired channel ports Once everything is connected, run the HP VEE program by clicking on the “Run” button (looks like an arrow). The waveform in the panel driver should show up in the display window. Scale the displays by clicking on “Auto Scale” To print the waveforms, right click on the black window of the display window and select “plot” EECS 145 L UC Berkeley Fall 2003 59 S. Derenzo
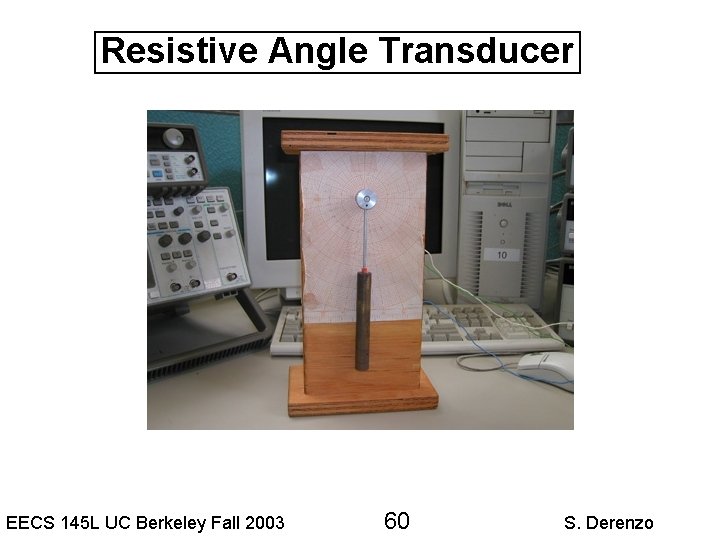
Resistive Angle Transducer EECS 145 L UC Berkeley Fall 2003 60 S. Derenzo
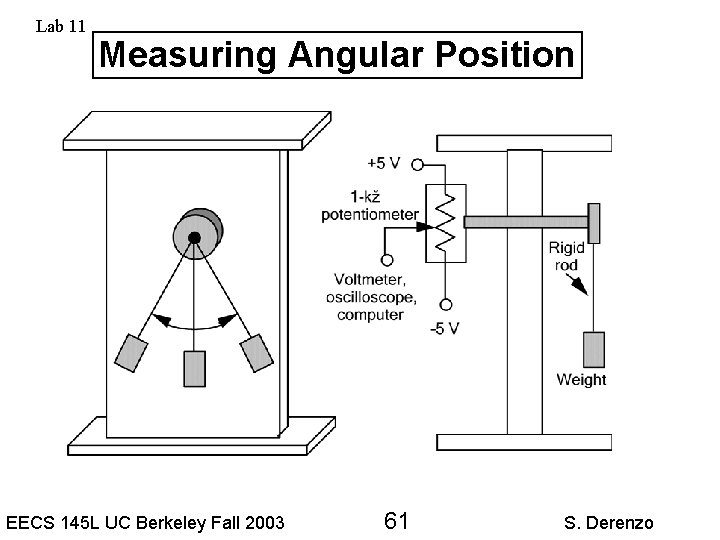
Lab 11 Measuring Angular Position EECS 145 L UC Berkeley Fall 2003 61 S. Derenzo
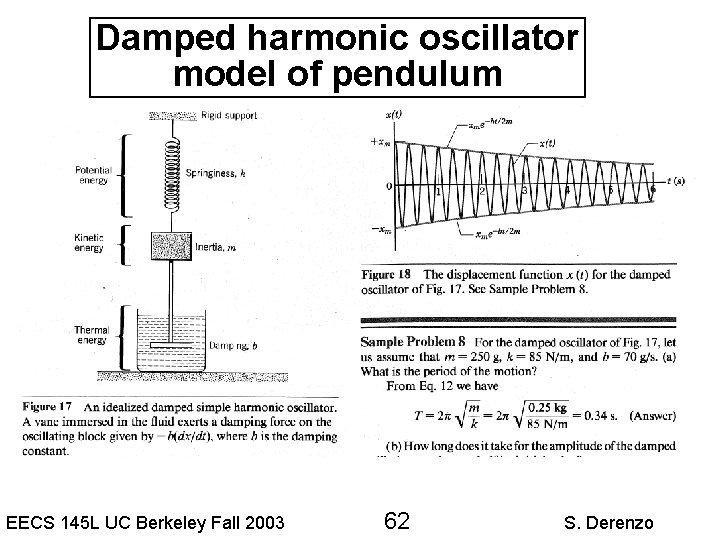
Damped harmonic oscillator model of pendulum EECS 145 L UC Berkeley Fall 2003 62 S. Derenzo
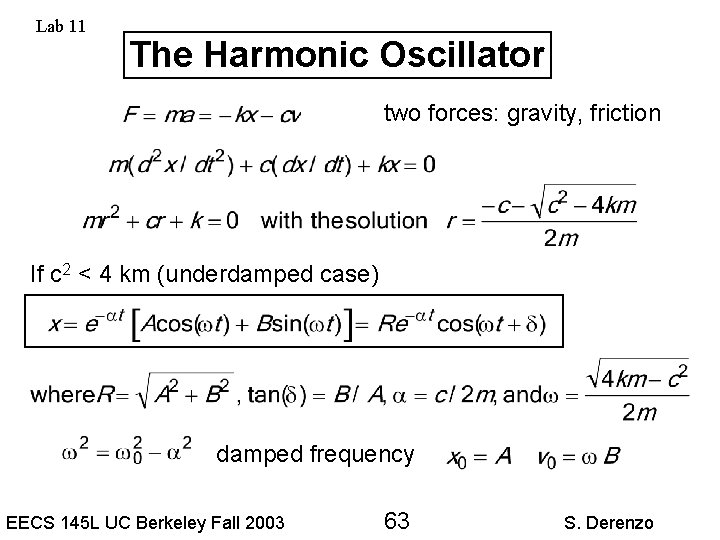
Lab 11 The Harmonic Oscillator two forces: gravity, friction If c 2 < 4 km (underdamped case) damped frequency EECS 145 L UC Berkeley Fall 2003 63 S. Derenzo
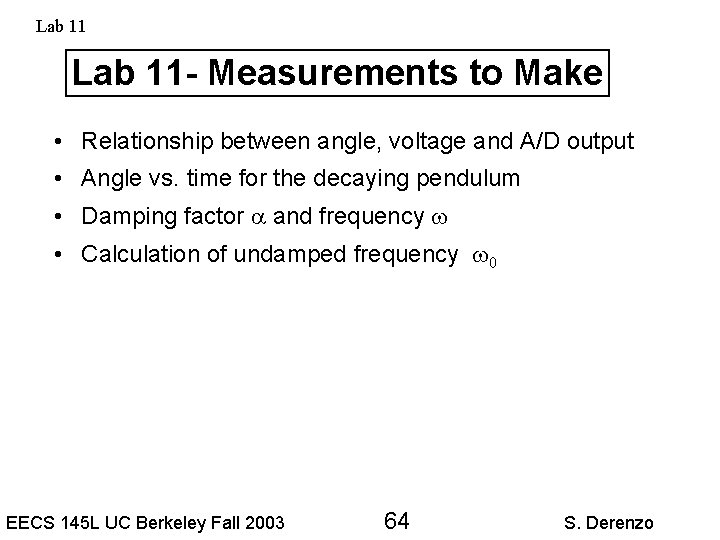
Lab 11 - Measurements to Make • Relationship between angle, voltage and A/D output • Angle vs. time for the decaying pendulum • Damping factor and frequency • Calculation of undamped frequency 0 EECS 145 L UC Berkeley Fall 2003 64 S. Derenzo
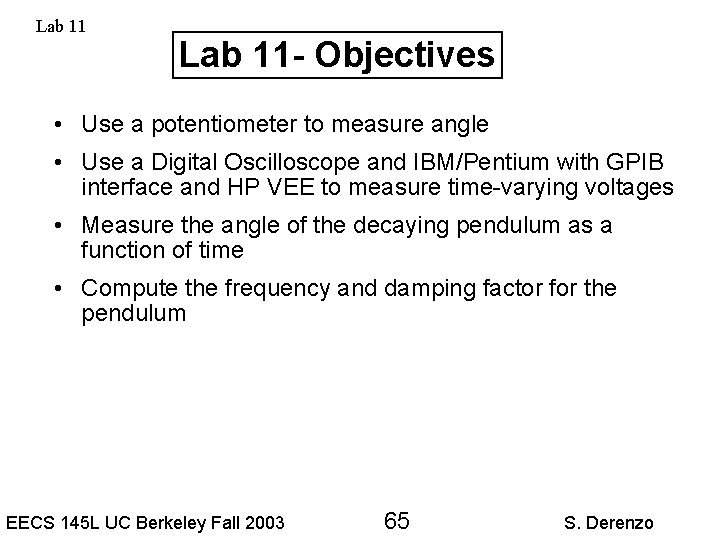
Lab 11 - Objectives • Use a potentiometer to measure angle • Use a Digital Oscilloscope and IBM/Pentium with GPIB interface and HP VEE to measure time-varying voltages • Measure the angle of the decaying pendulum as a function of time • Compute the frequency and damping factor for the pendulum EECS 145 L UC Berkeley Fall 2003 65 S. Derenzo
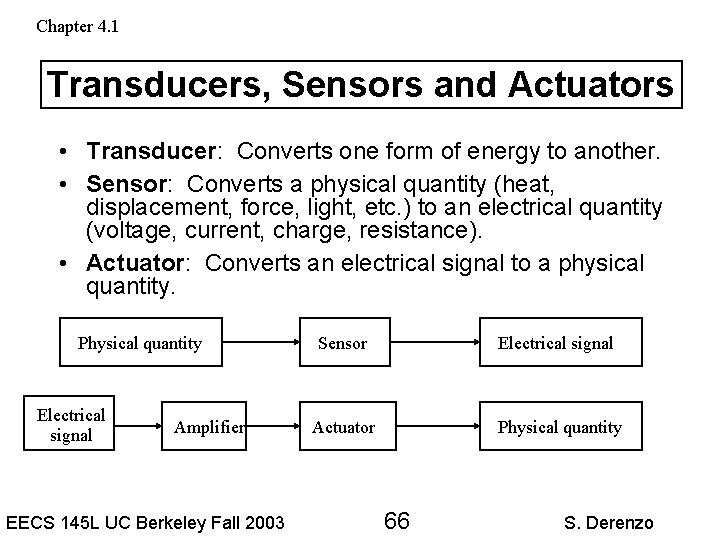
Chapter 4. 1 Transducers, Sensors and Actuators • Transducer: Converts one form of energy to another. • Sensor: Converts a physical quantity (heat, displacement, force, light, etc. ) to an electrical quantity (voltage, current, charge, resistance). • Actuator: Converts an electrical signal to a physical quantity. Physical quantity Electrical signal Amplifier EECS 145 L UC Berkeley Fall 2003 Sensor Electrical signal Actuator Physical quantity 66 S. Derenzo
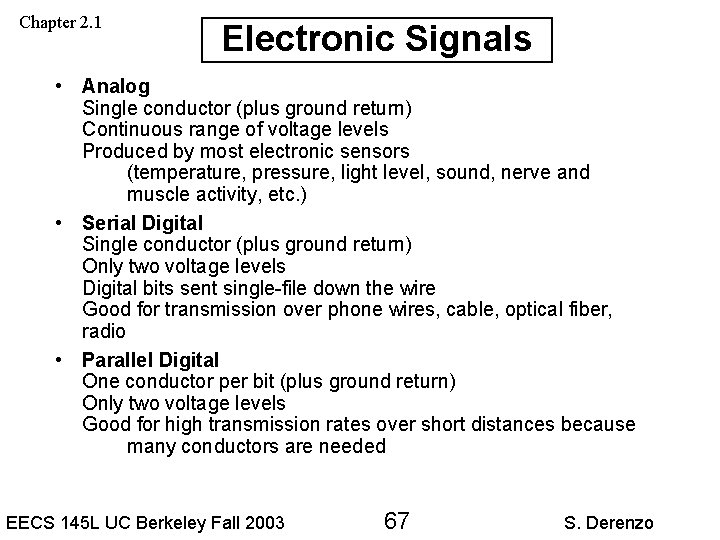
Chapter 2. 1 Electronic Signals • Analog Single conductor (plus ground return) Continuous range of voltage levels Produced by most electronic sensors (temperature, pressure, light level, sound, nerve and muscle activity, etc. ) • Serial Digital Single conductor (plus ground return) Only two voltage levels Digital bits sent single-file down the wire Good for transmission over phone wires, cable, optical fiber, radio • Parallel Digital One conductor per bit (plus ground return) Only two voltage levels Good for high transmission rates over short distances because many conductors are needed EECS 145 L UC Berkeley Fall 2003 67 S. Derenzo
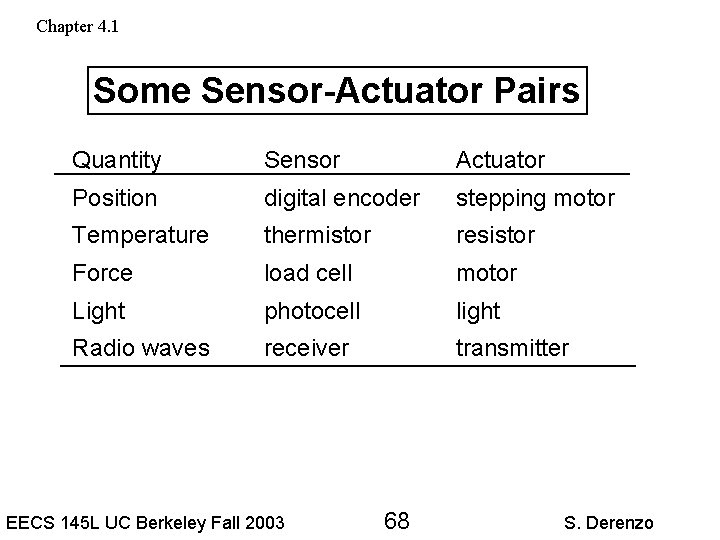
Chapter 4. 1 Some Sensor-Actuator Pairs Quantity Sensor Actuator Position digital encoder stepping motor Temperature thermistor resistor Force load cell motor Light photocell light Radio waves receiver transmitter EECS 145 L UC Berkeley Fall 2003 68 S. Derenzo
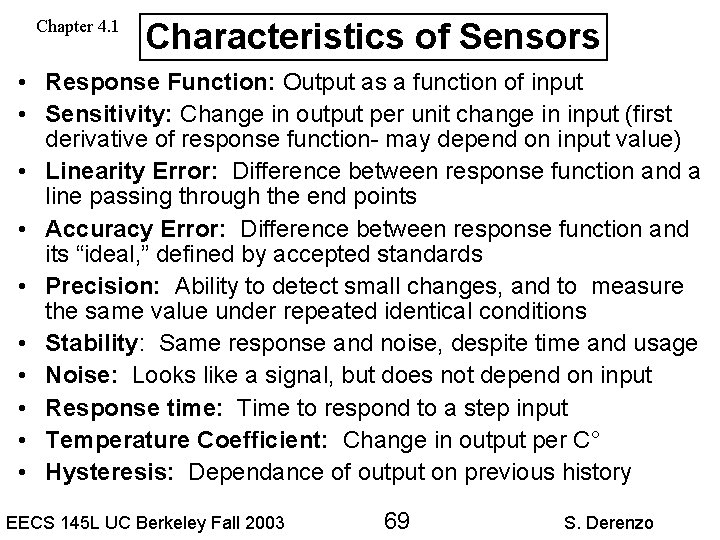
Chapter 4. 1 Characteristics of Sensors • Response Function: Output as a function of input • Sensitivity: Change in output per unit change in input (first derivative of response function- may depend on input value) • Linearity Error: Difference between response function and a line passing through the end points • Accuracy Error: Difference between response function and its “ideal, ” defined by accepted standards • Precision: Ability to detect small changes, and to measure the same value under repeated identical conditions • Stability: Same response and noise, despite time and usage • Noise: Looks like a signal, but does not depend on input • Response time: Time to respond to a step input • Temperature Coefficient: Change in output per C° • Hysteresis: Dependance of output on previous history EECS 145 L UC Berkeley Fall 2003 69 S. Derenzo
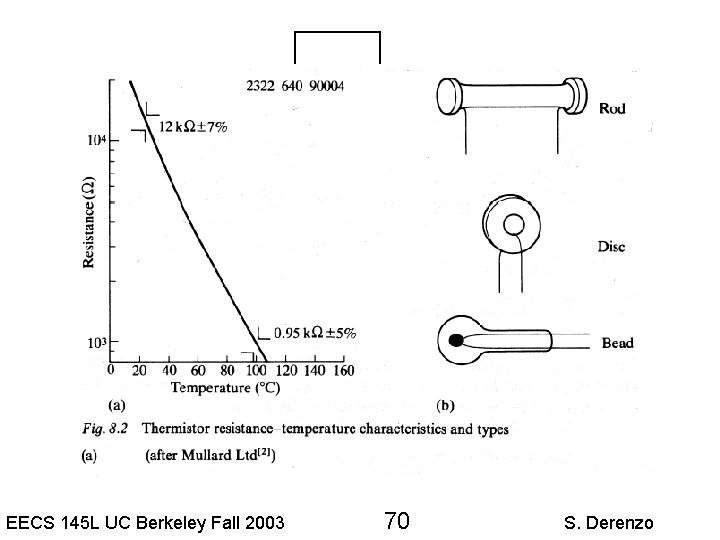
EECS 145 L UC Berkeley Fall 2003 70 S. Derenzo
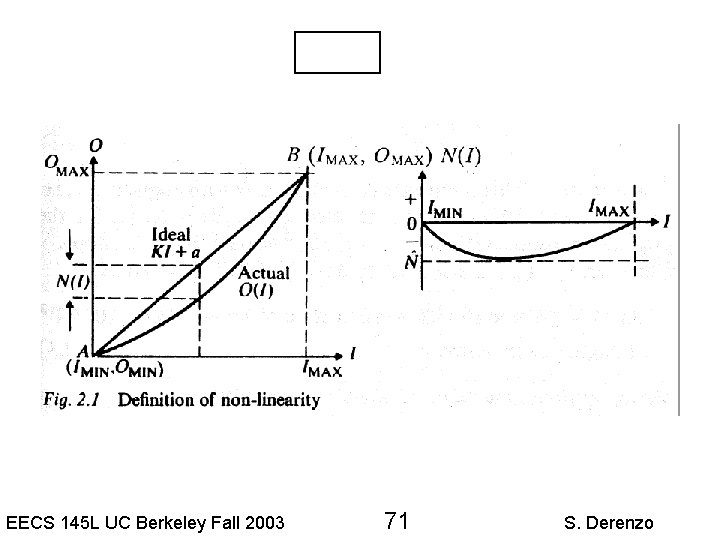
EECS 145 L UC Berkeley Fall 2003 71 S. Derenzo
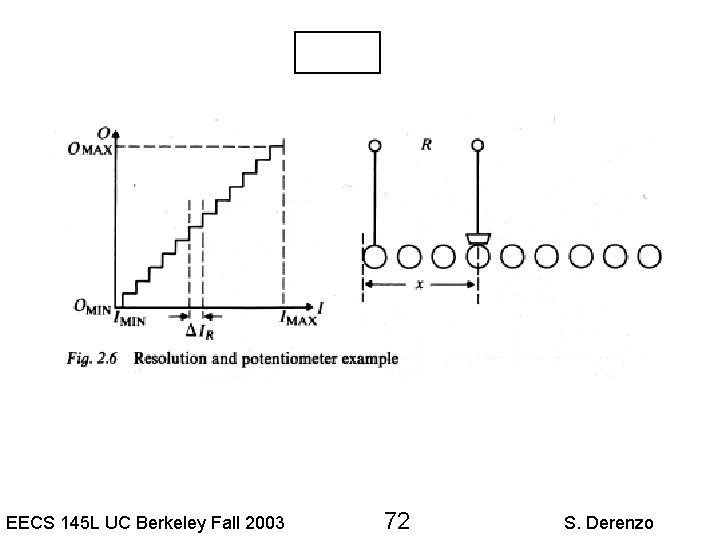
EECS 145 L UC Berkeley Fall 2003 72 S. Derenzo
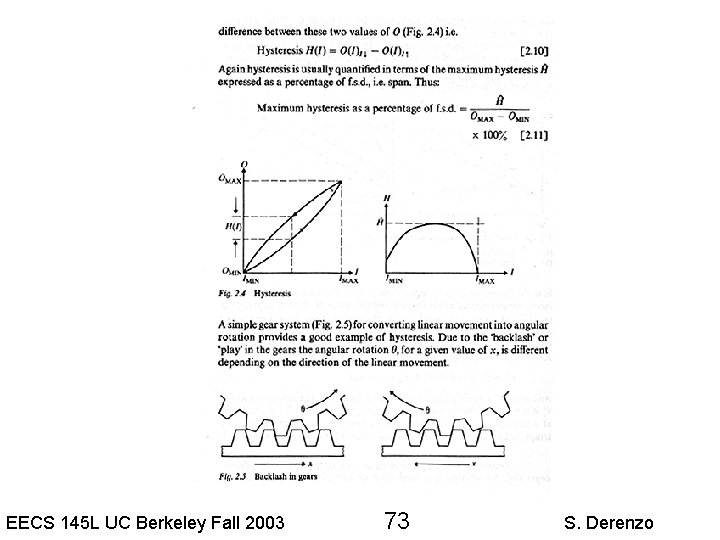
EECS 145 L UC Berkeley Fall 2003 73 S. Derenzo
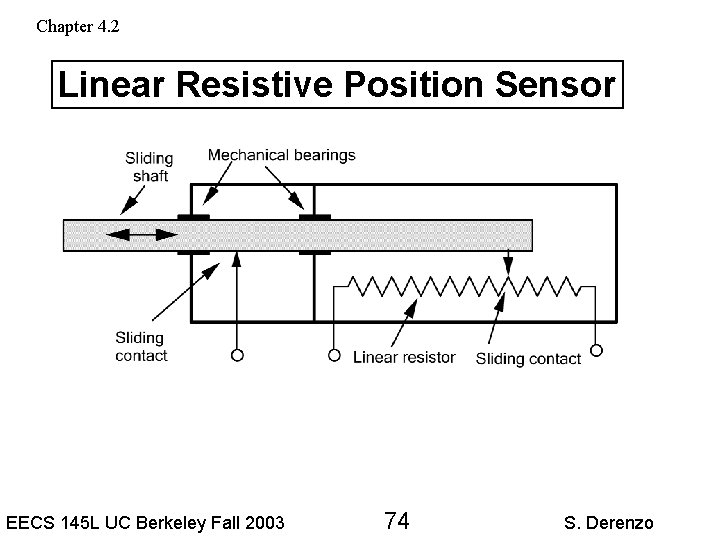
Chapter 4. 2 Linear Resistive Position Sensor EECS 145 L UC Berkeley Fall 2003 74 S. Derenzo
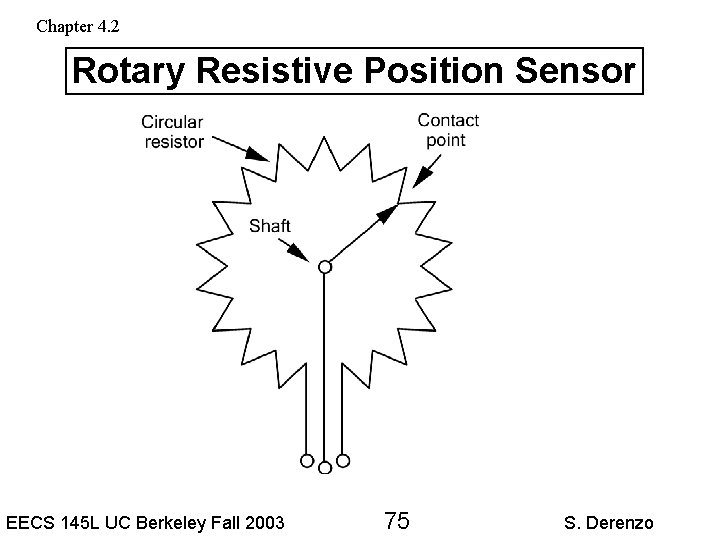
Chapter 4. 2 Rotary Resistive Position Sensor EECS 145 L UC Berkeley Fall 2003 75 S. Derenzo
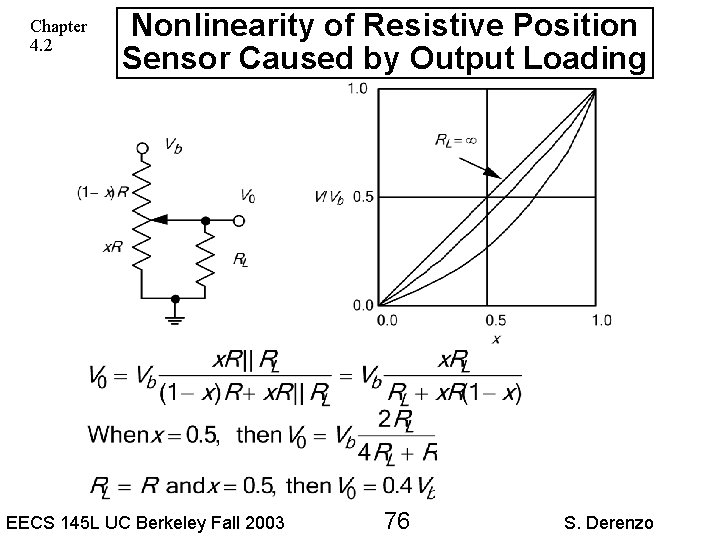
Chapter 4. 2 Nonlinearity of Resistive Position Sensor Caused by Output Loading EECS 145 L UC Berkeley Fall 2003 76 S. Derenzo
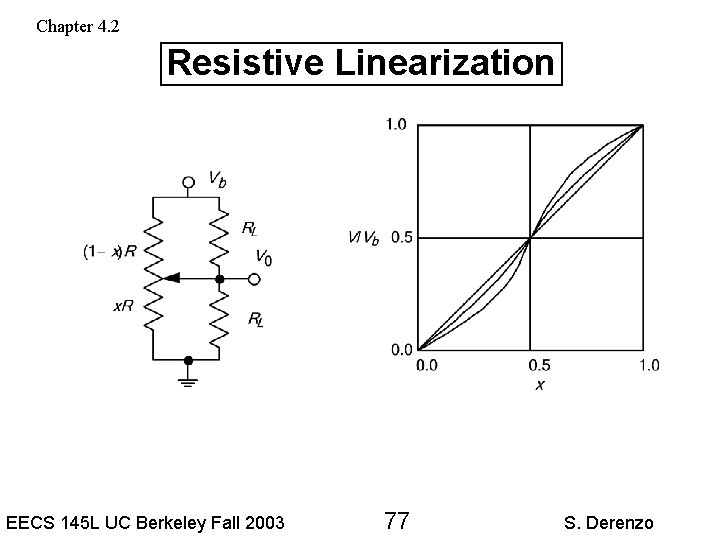
Chapter 4. 2 Resistive Linearization EECS 145 L UC Berkeley Fall 2003 77 S. Derenzo
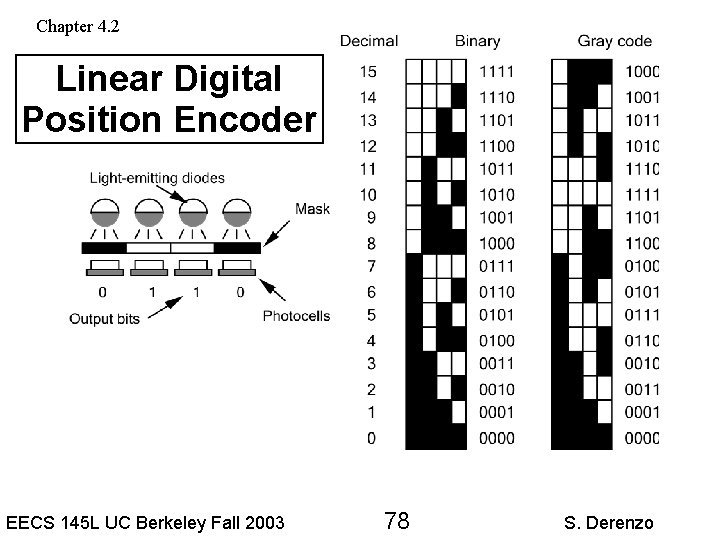
Chapter 4. 2 Linear Digital Position Encoder EECS 145 L UC Berkeley Fall 2003 78 S. Derenzo
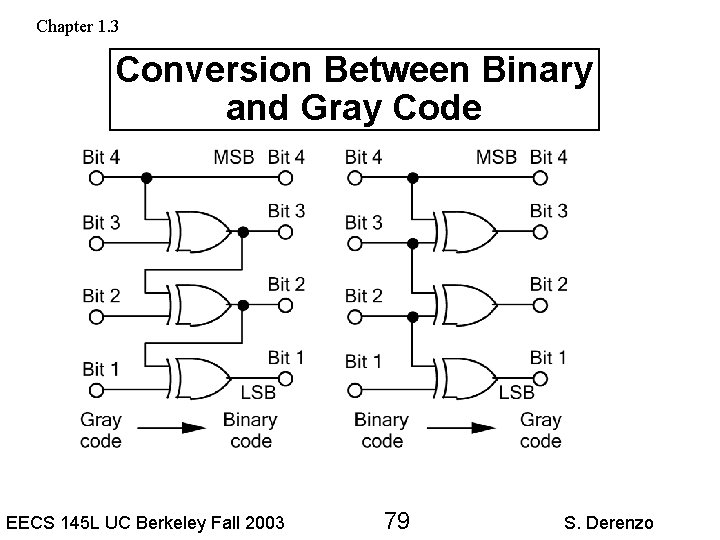
Chapter 1. 3 Conversion Between Binary and Gray Code EECS 145 L UC Berkeley Fall 2003 79 S. Derenzo
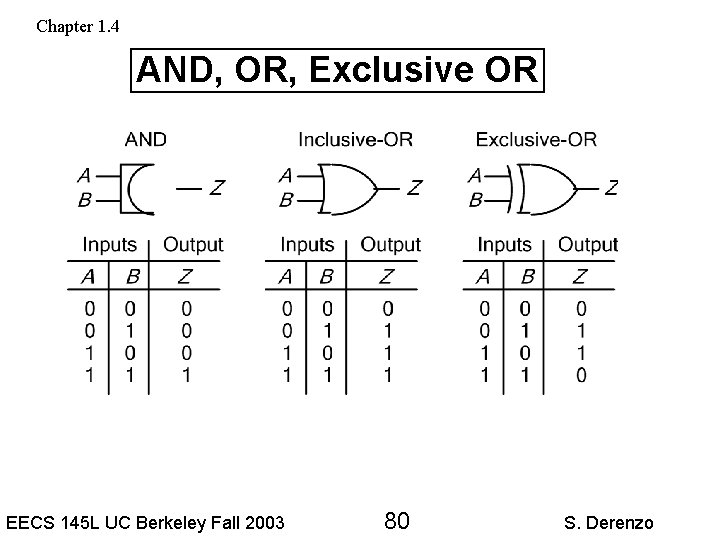
Chapter 1. 4 AND, OR, Exclusive OR EECS 145 L UC Berkeley Fall 2003 80 S. Derenzo
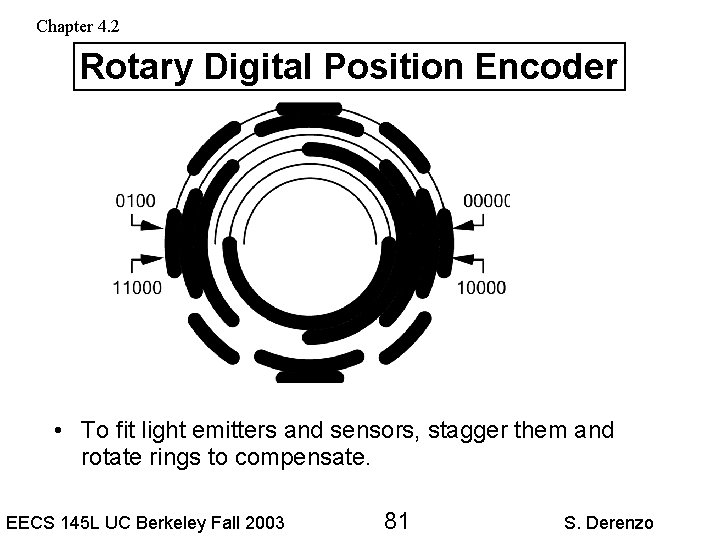
Chapter 4. 2 Rotary Digital Position Encoder • To fit light emitters and sensors, stagger them and rotate rings to compensate. EECS 145 L UC Berkeley Fall 2003 81 S. Derenzo
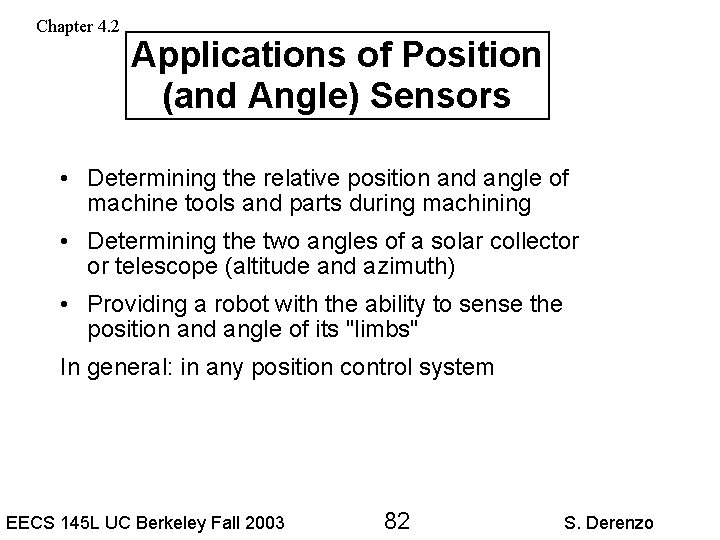
Chapter 4. 2 Applications of Position (and Angle) Sensors • Determining the relative position and angle of machine tools and parts during machining • Determining the two angles of a solar collector or telescope (altitude and azimuth) • Providing a robot with the ability to sense the position and angle of its "limbs" In general: in any position control system EECS 145 L UC Berkeley Fall 2003 82 S. Derenzo
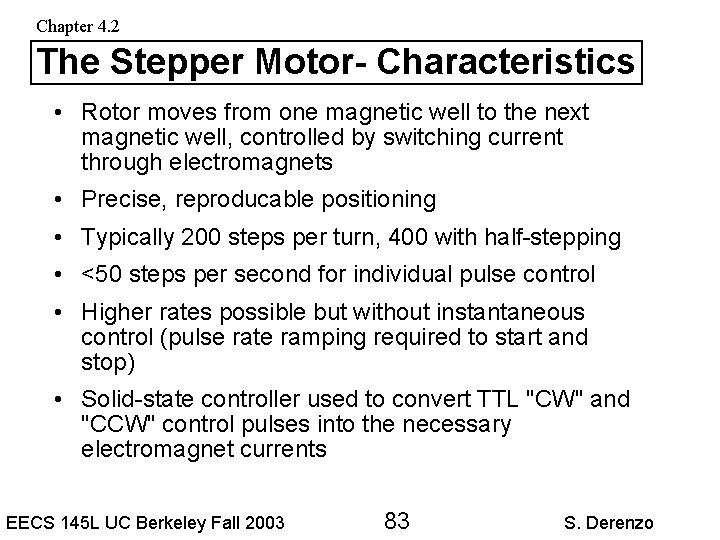
Chapter 4. 2 The Stepper Motor- Characteristics • Rotor moves from one magnetic well to the next magnetic well, controlled by switching current through electromagnets • Precise, reproducable positioning • Typically 200 steps per turn, 400 with half-stepping • <50 steps per second for individual pulse control • Higher rates possible but without instantaneous control (pulse rate ramping required to start and stop) • Solid-state controller used to convert TTL "CW" and "CCW" control pulses into the necessary electromagnet currents EECS 145 L UC Berkeley Fall 2003 83 S. Derenzo
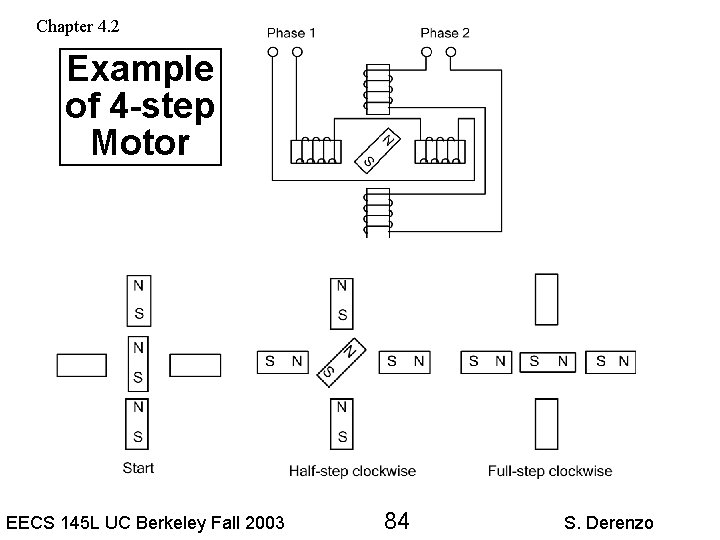
Chapter 4. 2 Example of 4 -step Motor EECS 145 L UC Berkeley Fall 2003 84 S. Derenzo
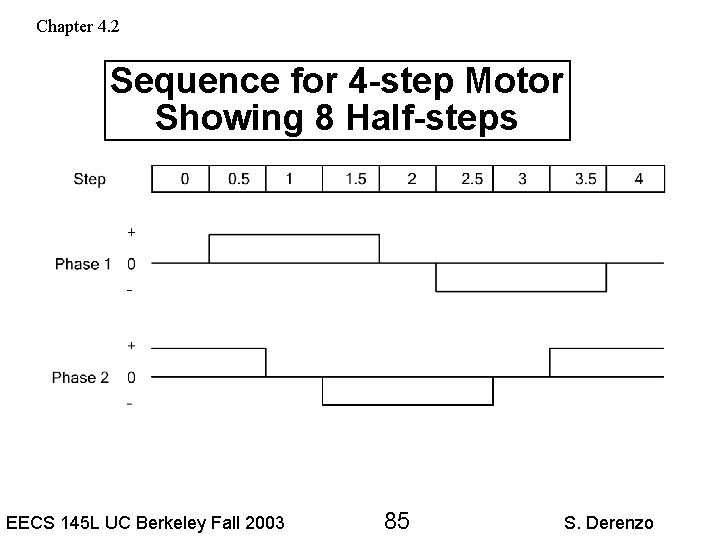
Chapter 4. 2 Sequence for 4 -step Motor Showing 8 Half-steps EECS 145 L UC Berkeley Fall 2003 85 S. Derenzo
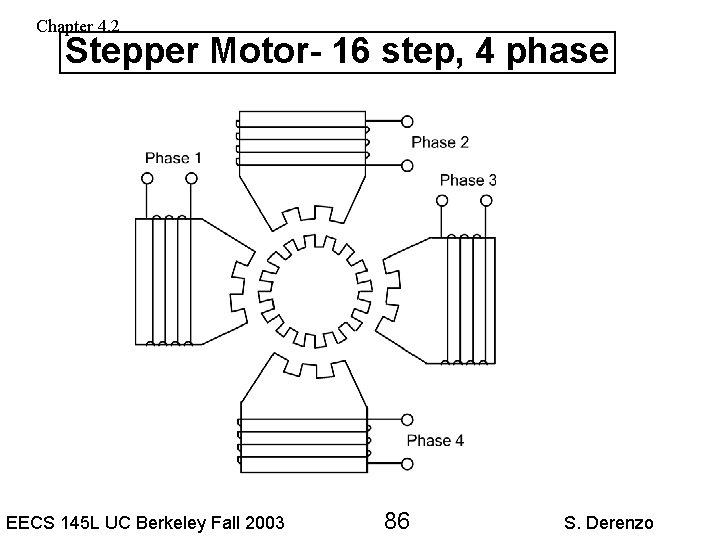
Chapter 4. 2 Stepper Motor- 16 step, 4 phase EECS 145 L UC Berkeley Fall 2003 86 S. Derenzo
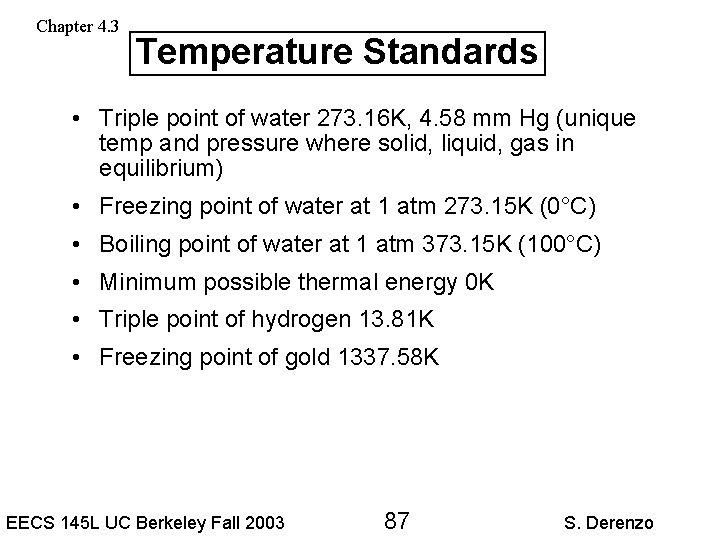
Chapter 4. 3 Temperature Standards • Triple point of water 273. 16 K, 4. 58 mm Hg (unique temp and pressure where solid, liquid, gas in equilibrium) • Freezing point of water at 1 atm 273. 15 K (0°C) • Boiling point of water at 1 atm 373. 15 K (100°C) • Minimum possible thermal energy 0 K • Triple point of hydrogen 13. 81 K • Freezing point of gold 1337. 58 K EECS 145 L UC Berkeley Fall 2003 87 S. Derenzo
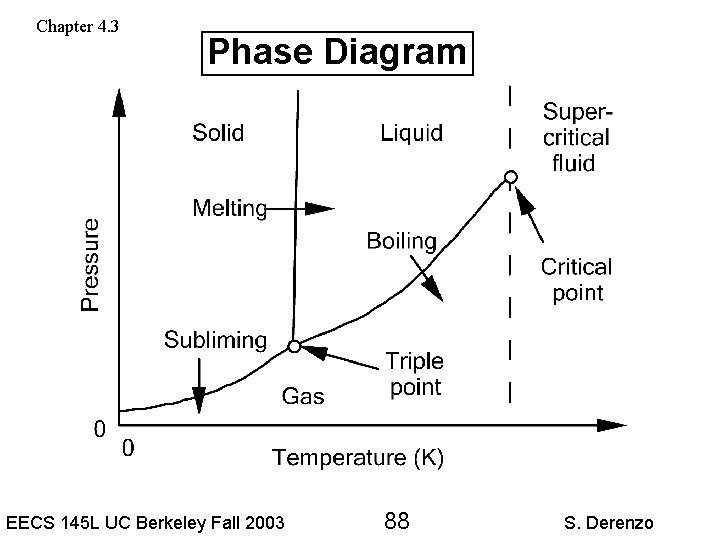
Chapter 4. 3 Phase Diagram EECS 145 L UC Berkeley Fall 2003 88 S. Derenzo
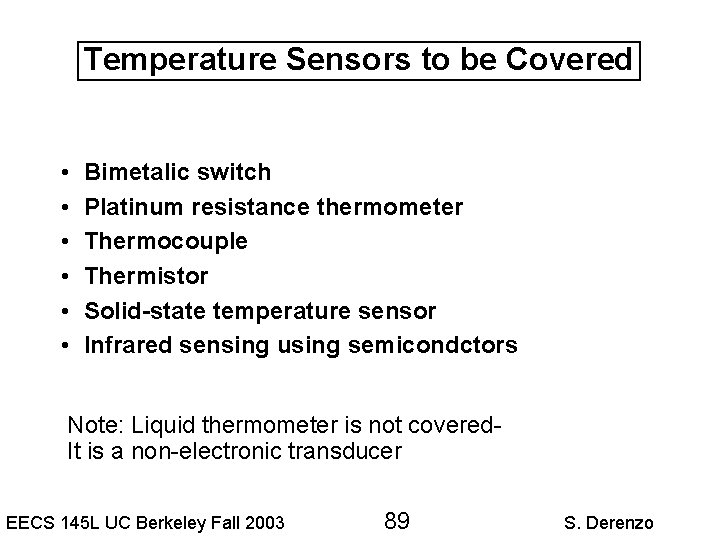
Temperature Sensors to be Covered • • • Bimetalic switch Platinum resistance thermometer Thermocouple Thermistor Solid-state temperature sensor Infrared sensing using semicondctors Note: Liquid thermometer is not covered. It is a non-electronic transducer EECS 145 L UC Berkeley Fall 2003 89 S. Derenzo
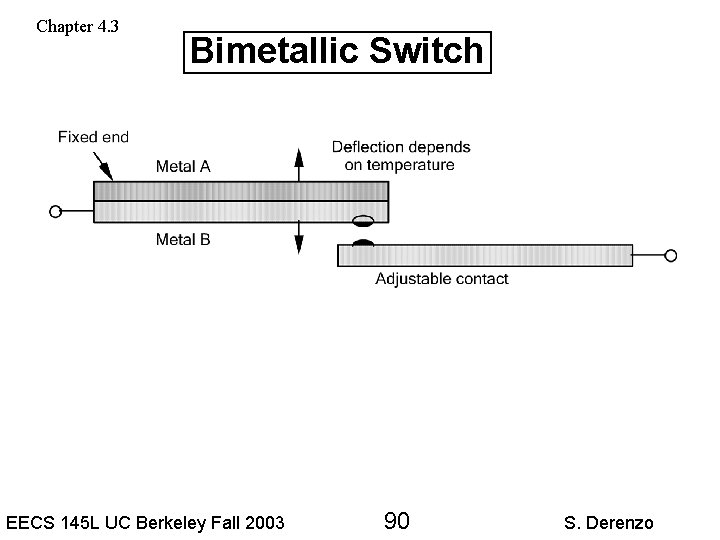
Chapter 4. 3 Bimetallic Switch EECS 145 L UC Berkeley Fall 2003 90 S. Derenzo
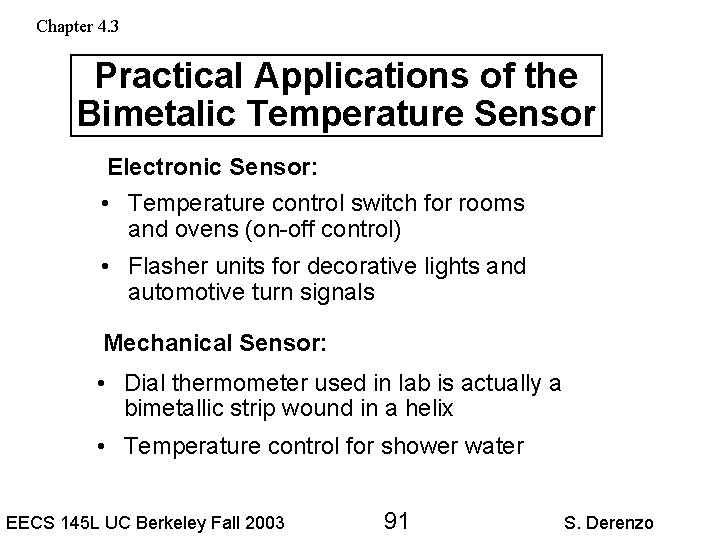
Chapter 4. 3 Practical Applications of the Bimetalic Temperature Sensor Electronic Sensor: • Temperature control switch for rooms and ovens (on-off control) • Flasher units for decorative lights and automotive turn signals Mechanical Sensor: • Dial thermometer used in lab is actually a bimetallic strip wound in a helix • Temperature control for shower water EECS 145 L UC Berkeley Fall 2003 91 S. Derenzo
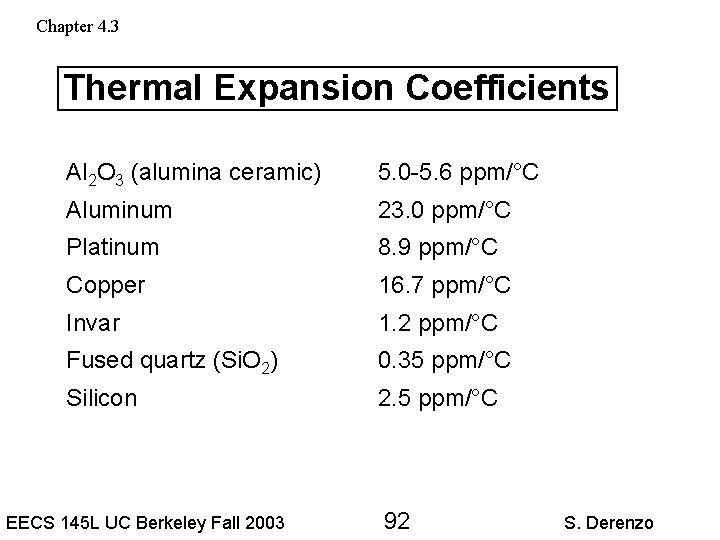
Chapter 4. 3 Thermal Expansion Coefficients Al 2 O 3 (alumina ceramic) 5. 0 -5. 6 ppm/°C Aluminum 23. 0 ppm/°C Platinum 8. 9 ppm/°C Copper 16. 7 ppm/°C Invar 1. 2 ppm/°C Fused quartz (Si. O 2) 0. 35 ppm/°C Silicon 2. 5 ppm/°C EECS 145 L UC Berkeley Fall 2003 92 S. Derenzo
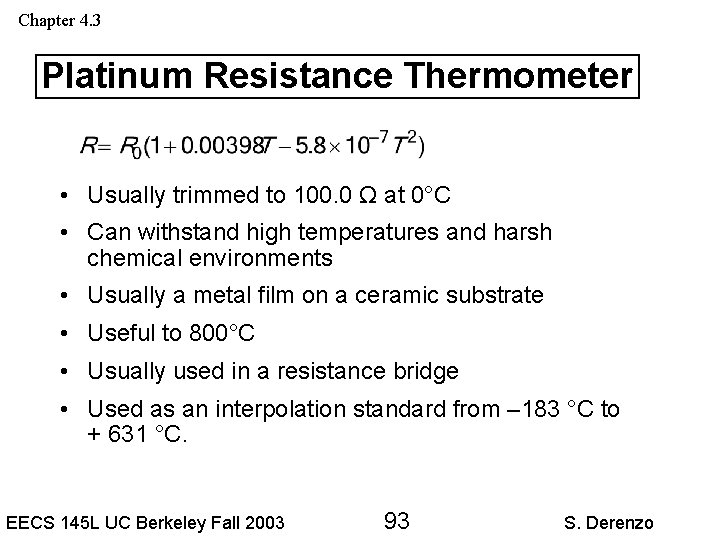
Chapter 4. 3 Platinum Resistance Thermometer • Usually trimmed to 100. 0 Ω at 0°C • Can withstand high temperatures and harsh chemical environments • Usually a metal film on a ceramic substrate • Useful to 800°C • Usually used in a resistance bridge • Used as an interpolation standard from – 183 °C to + 631 °C. EECS 145 L UC Berkeley Fall 2003 93 S. Derenzo
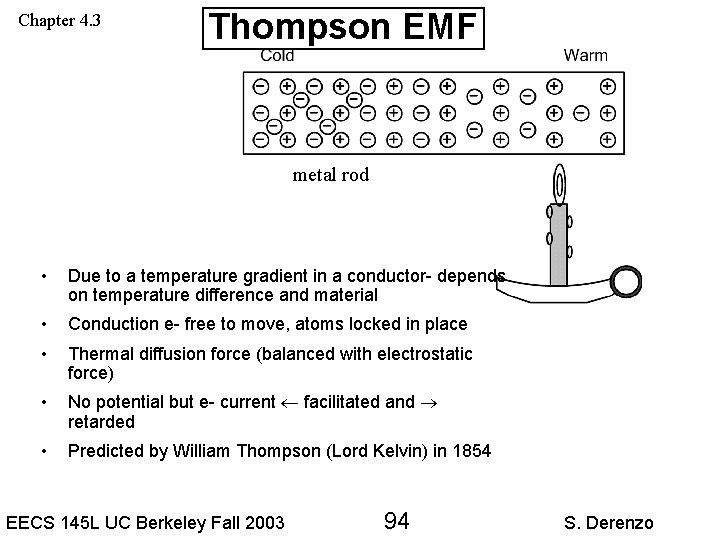
Chapter 4. 3 Thompson EMF metal rod • Due to a temperature gradient in a conductor- depends on temperature difference and material • Conduction e- free to move, atoms locked in place • Thermal diffusion force (balanced with electrostatic force) • No potential but e- current facilitated and retarded • Predicted by William Thompson (Lord Kelvin) in 1854 EECS 145 L UC Berkeley Fall 2003 94 S. Derenzo
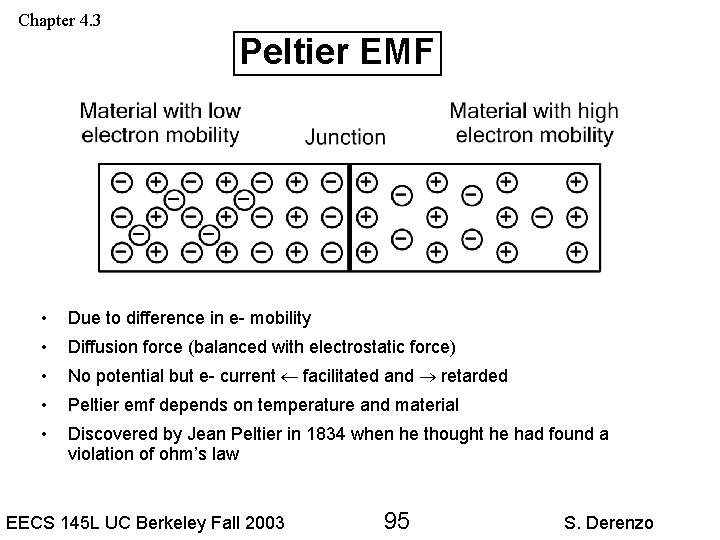
Chapter 4. 3 Peltier EMF • Due to difference in e- mobility • Diffusion force (balanced with electrostatic force) • No potential but e- current facilitated and retarded • Peltier emf depends on temperature and material • Discovered by Jean Peltier in 1834 when he thought he had found a violation of ohm’s law EECS 145 L UC Berkeley Fall 2003 95 S. Derenzo
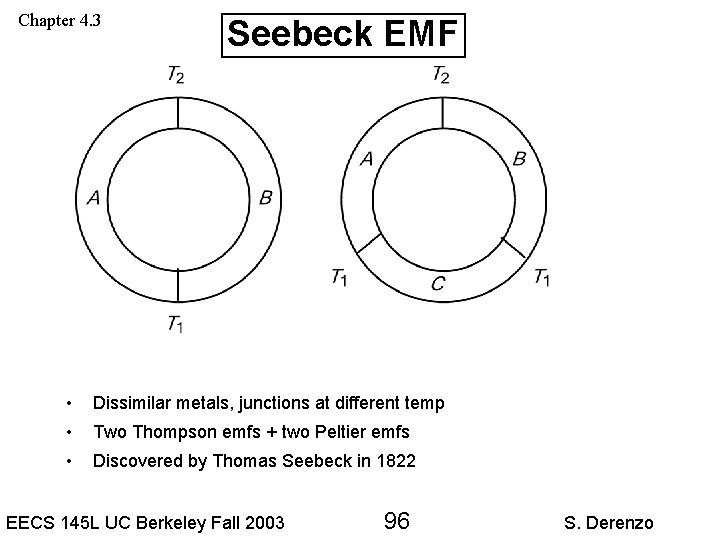
Chapter 4. 3 Seebeck EMF • Dissimilar metals, junctions at different temp • Two Thompson emfs + two Peltier emfs • Discovered by Thomas Seebeck in 1822 EECS 145 L UC Berkeley Fall 2003 96 S. Derenzo
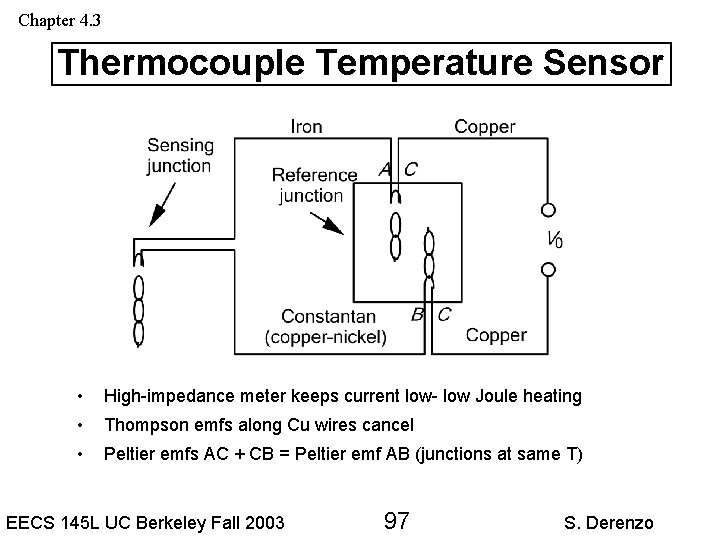
Chapter 4. 3 Thermocouple Temperature Sensor • High-impedance meter keeps current low- low Joule heating • Thompson emfs along Cu wires cancel • Peltier emfs AC + CB = Peltier emf AB (junctions at same T) EECS 145 L UC Berkeley Fall 2003 97 S. Derenzo
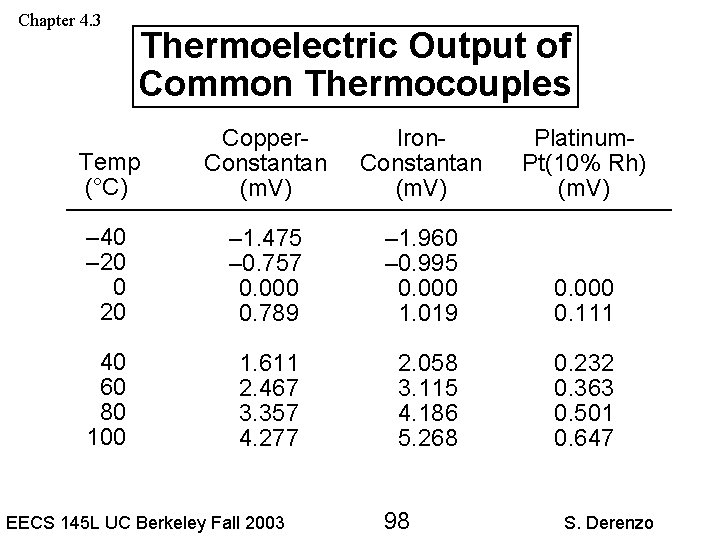
Chapter 4. 3 Thermoelectric Output of Common Thermocouples Temp (°C) Copper. Constantan (m. V) Iron. Constantan (m. V) Platinum. Pt(10% Rh) (m. V) – 40 – 20 0 20 – 1. 475 – 0. 757 0. 000 0. 789 – 1. 960 – 0. 995 0. 000 1. 019 0. 000 0. 111 40 60 80 100 1. 611 2. 467 3. 357 4. 277 2. 058 3. 115 4. 186 5. 268 0. 232 0. 363 0. 501 0. 647 EECS 145 L UC Berkeley Fall 2003 98 S. Derenzo
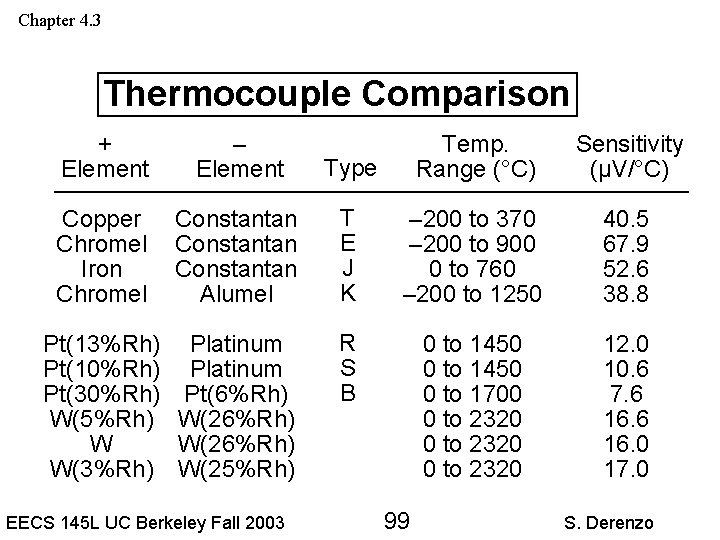
Chapter 4. 3 Thermocouple Comparison + Element – Element Type Temp. Range (°C) Sensitivity (µV/°C) Copper Chromel Iron Chromel Constantan Alumel T E J K – 200 to 370 – 200 to 900 0 to 760 – 200 to 1250 40. 5 67. 9 52. 6 38. 8 Pt(13%Rh) Pt(10%Rh) Pt(30%Rh) W(5%Rh) W W(3%Rh) Platinum Pt(6%Rh) W(26%Rh) W(25%Rh) R S B 0 to 1450 0 to 1700 0 to 2320 12. 0 10. 6 7. 6 16. 0 17. 0 EECS 145 L UC Berkeley Fall 2003 99 S. Derenzo
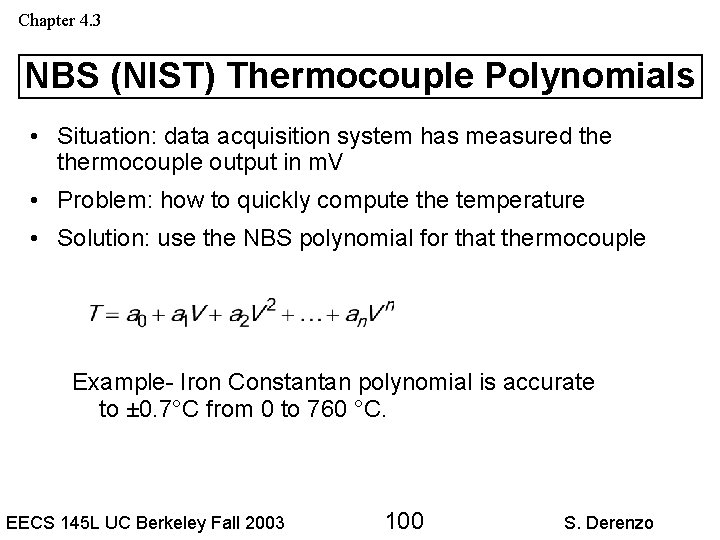
Chapter 4. 3 NBS (NIST) Thermocouple Polynomials • Situation: data acquisition system has measured thermocouple output in m. V • Problem: how to quickly compute the temperature • Solution: use the NBS polynomial for that thermocouple Example- Iron Constantan polynomial is accurate to ± 0. 7°C from 0 to 760 °C. EECS 145 L UC Berkeley Fall 2003 100 S. Derenzo
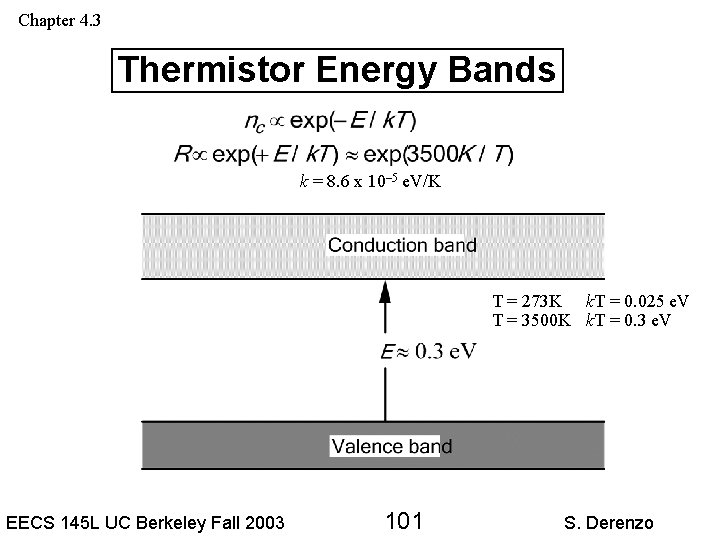
Chapter 4. 3 Thermistor Energy Bands k = 8. 6 x 10– 5 e. V/K T = 273 K k. T = 0. 025 e. V T = 3500 K k. T = 0. 3 e. V EECS 145 L UC Berkeley Fall 2003 101 S. Derenzo
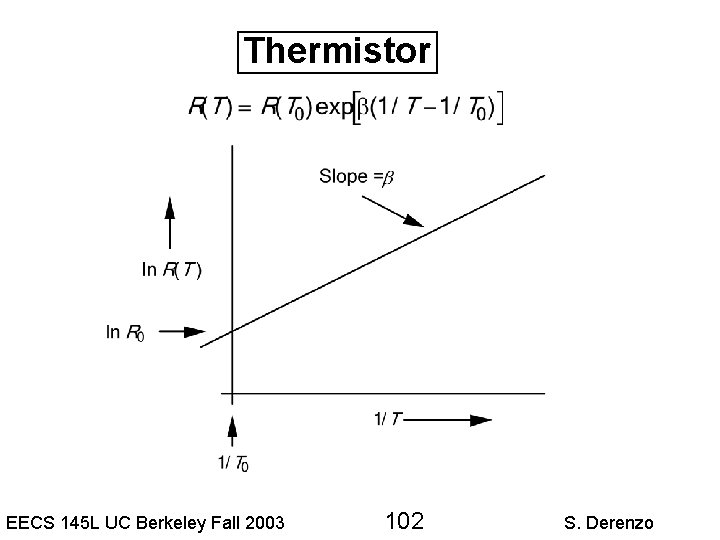
Thermistor EECS 145 L UC Berkeley Fall 2003 102 S. Derenzo
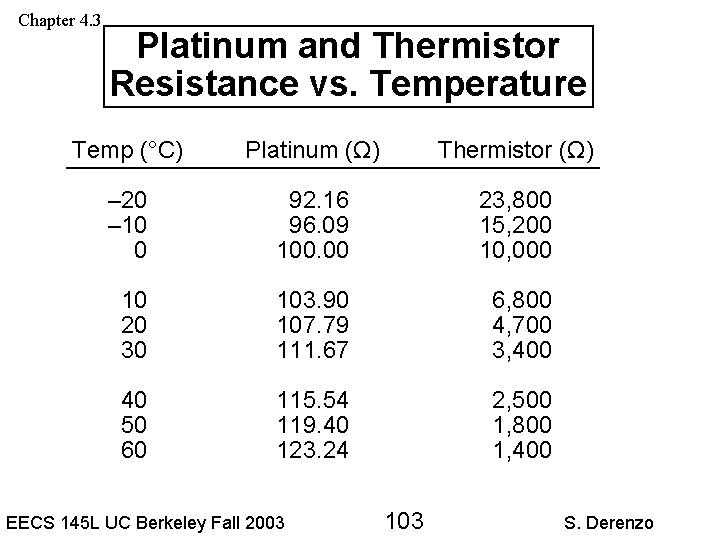
Chapter 4. 3 Platinum and Thermistor Resistance vs. Temperature Temp (°C) Platinum (Ω) Thermistor (Ω) – 20 – 10 0 92. 16 96. 09 100. 00 23, 800 15, 200 10, 000 10 20 30 103. 90 107. 79 111. 67 6, 800 4, 700 3, 400 40 50 60 115. 54 119. 40 123. 24 2, 500 1, 800 1, 400 EECS 145 L UC Berkeley Fall 2003 103 S. Derenzo
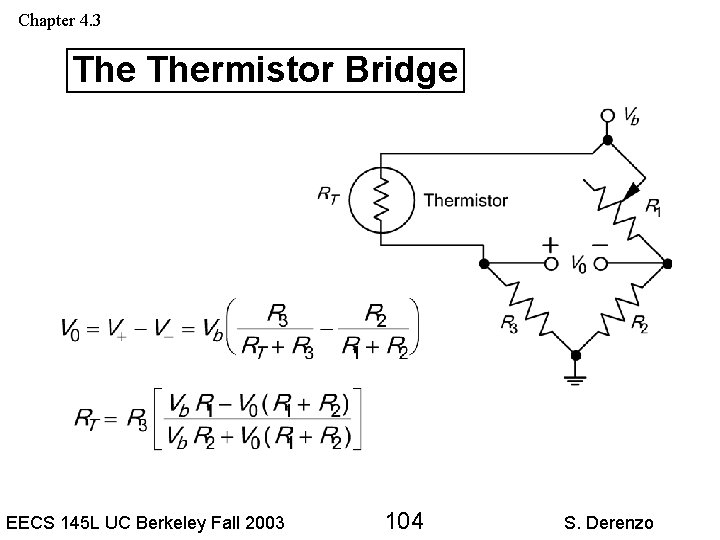
Chapter 4. 3 Thermistor Bridge EECS 145 L UC Berkeley Fall 2003 104 S. Derenzo
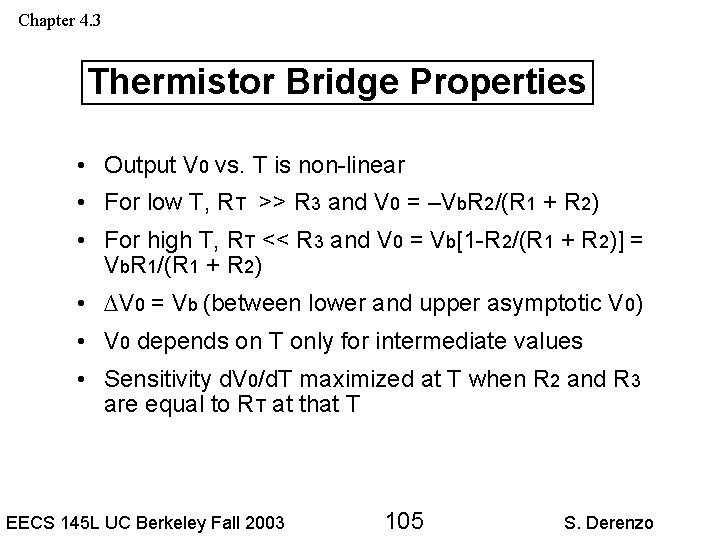
Chapter 4. 3 Thermistor Bridge Properties • Output V 0 vs. T is non-linear • For low T, RT >> R 3 and V 0 = –Vb. R 2/(R 1 + R 2) • For high T, RT << R 3 and V 0 = Vb[1 -R 2/(R 1 + R 2)] = Vb. R 1/(R 1 + R 2) • ∆V 0 = Vb (between lower and upper asymptotic V 0) • V 0 depends on T only for intermediate values • Sensitivity d. V 0/d. T maximized at T when R 2 and R 3 are equal to RT at that T EECS 145 L UC Berkeley Fall 2003 105 S. Derenzo
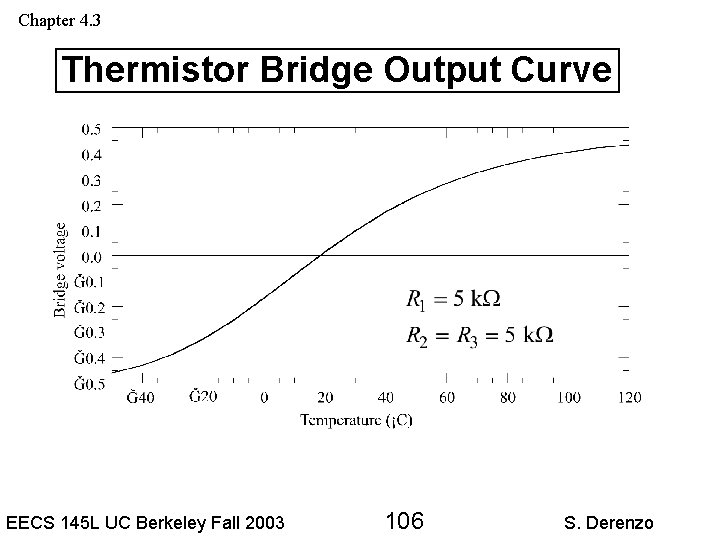
Chapter 4. 3 Thermistor Bridge Output Curve EECS 145 L UC Berkeley Fall 2003 106 S. Derenzo
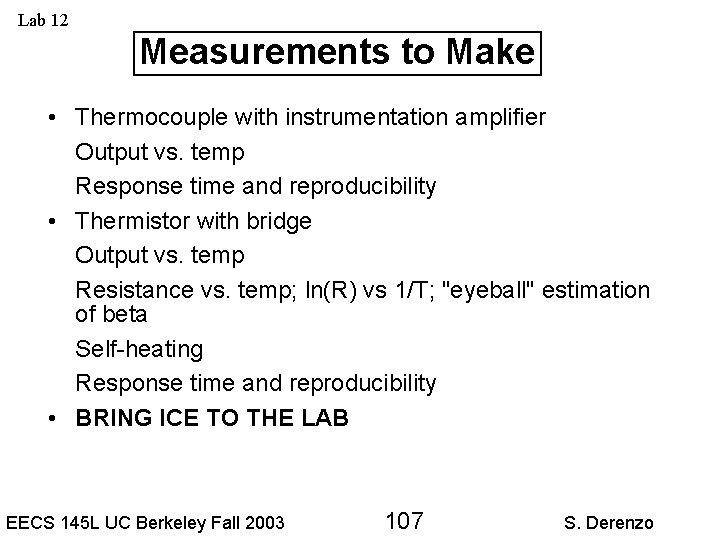
Lab 12 Measurements to Make • Thermocouple with instrumentation amplifier Output vs. temp Response time and reproducibility • Thermistor with bridge Output vs. temp Resistance vs. temp; ln(R) vs 1/T; "eyeball" estimation of beta Self-heating Response time and reproducibility • BRING ICE TO THE LAB EECS 145 L UC Berkeley Fall 2003 107 S. Derenzo
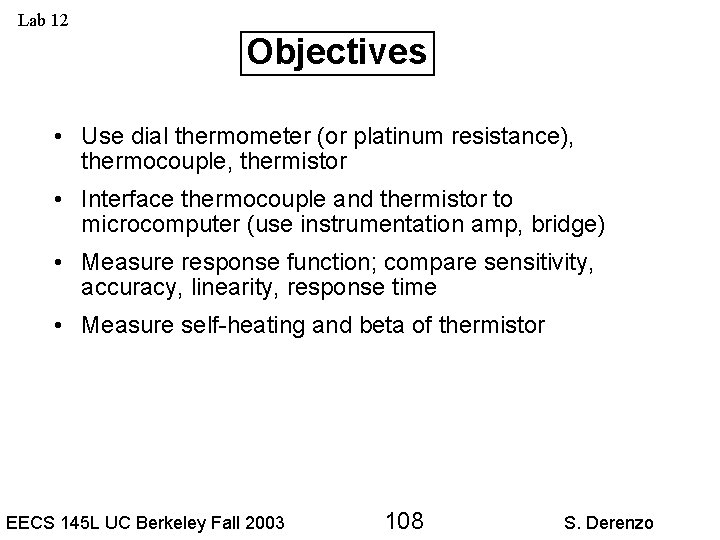
Lab 12 Objectives • Use dial thermometer (or platinum resistance), thermocouple, thermistor • Interface thermocouple and thermistor to microcomputer (use instrumentation amp, bridge) • Measure response function; compare sensitivity, accuracy, linearity, response time • Measure self-heating and beta of thermistor EECS 145 L UC Berkeley Fall 2003 108 S. Derenzo
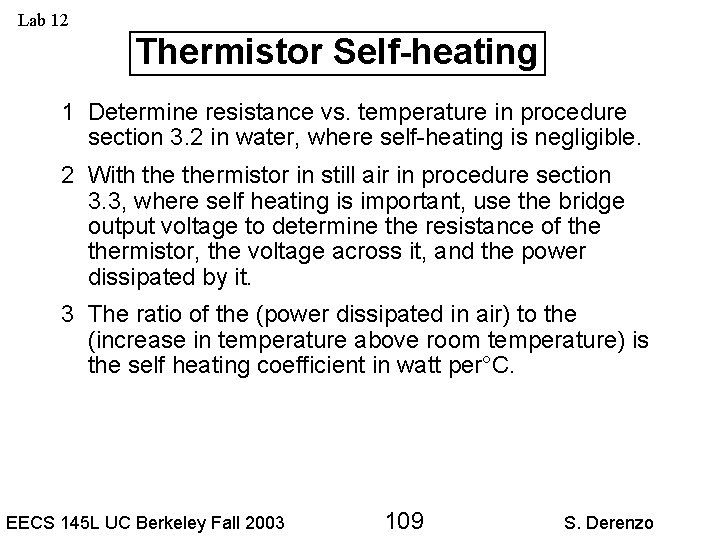
Lab 12 Thermistor Self-heating 1 Determine resistance vs. temperature in procedure section 3. 2 in water, where self-heating is negligible. 2 With thermistor in still air in procedure section 3. 3, where self heating is important, use the bridge output voltage to determine the resistance of thermistor, the voltage across it, and the power dissipated by it. 3 The ratio of the (power dissipated in air) to the (increase in temperature above room temperature) is the self heating coefficient in watt per°C. EECS 145 L UC Berkeley Fall 2003 109 S. Derenzo
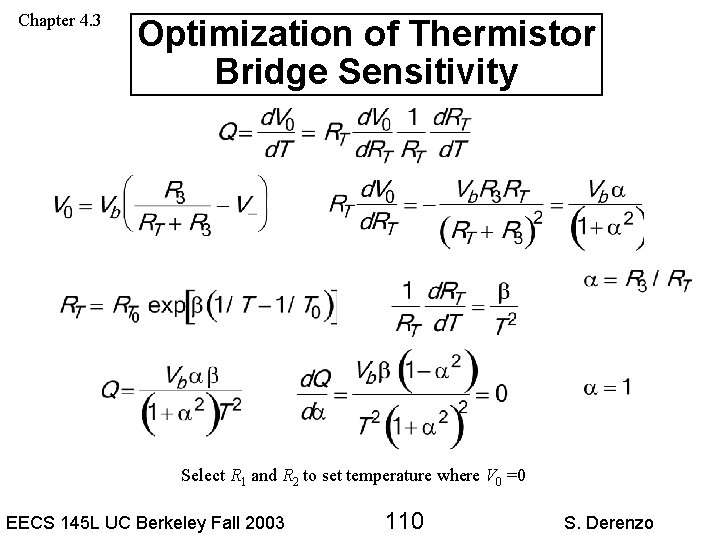
Chapter 4. 3 Optimization of Thermistor Bridge Sensitivity Select R 1 and R 2 to set temperature where V 0 =0 EECS 145 L UC Berkeley Fall 2003 110 S. Derenzo
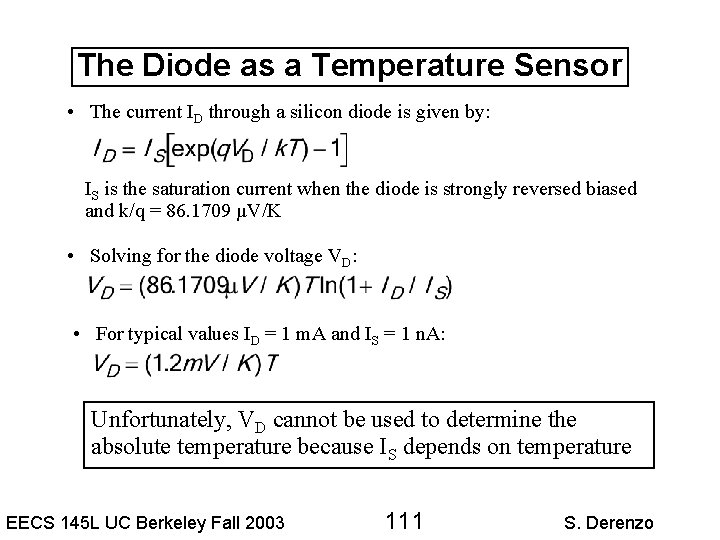
The Diode as a Temperature Sensor • The current ID through a silicon diode is given by: IS is the saturation current when the diode is strongly reversed biased and k/q = 86. 1709 µV/K • Solving for the diode voltage VD: • For typical values ID = 1 m. A and IS = 1 n. A: Unfortunately, VD cannot be used to determine the absolute temperature because IS depends on temperature EECS 145 L UC Berkeley Fall 2003 111 S. Derenzo
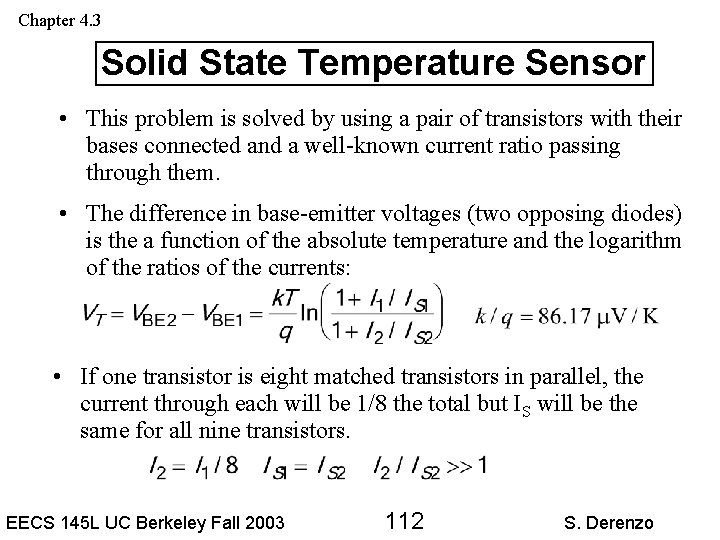
Chapter 4. 3 Solid State Temperature Sensor • This problem is solved by using a pair of transistors with their bases connected and a well-known current ratio passing through them. • The difference in base-emitter voltages (two opposing diodes) is the a function of the absolute temperature and the logarithm of the ratios of the currents: • If one transistor is eight matched transistors in parallel, the current through each will be 1/8 the total but IS will be the same for all nine transistors. EECS 145 L UC Berkeley Fall 2003 112 S. Derenzo
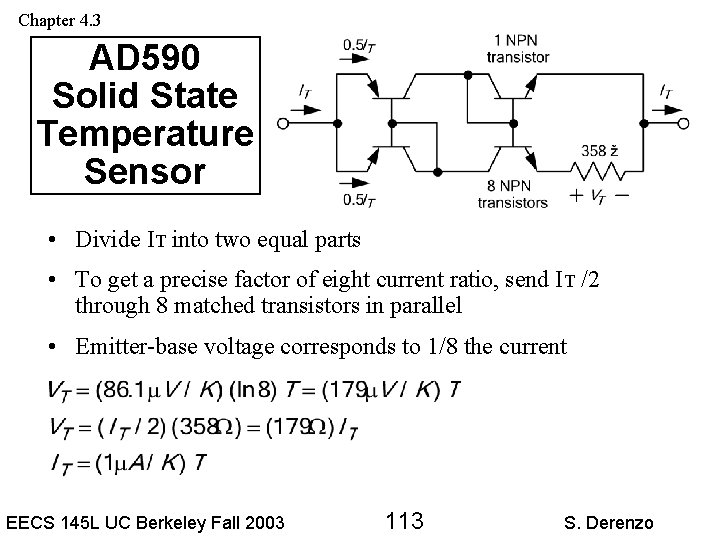
Chapter 4. 3 AD 590 Solid State Temperature Sensor • Divide IT into two equal parts • To get a precise factor of eight current ratio, send IT /2 through 8 matched transistors in parallel • Emitter-base voltage corresponds to 1/8 the current EECS 145 L UC Berkeley Fall 2003 113 S. Derenzo
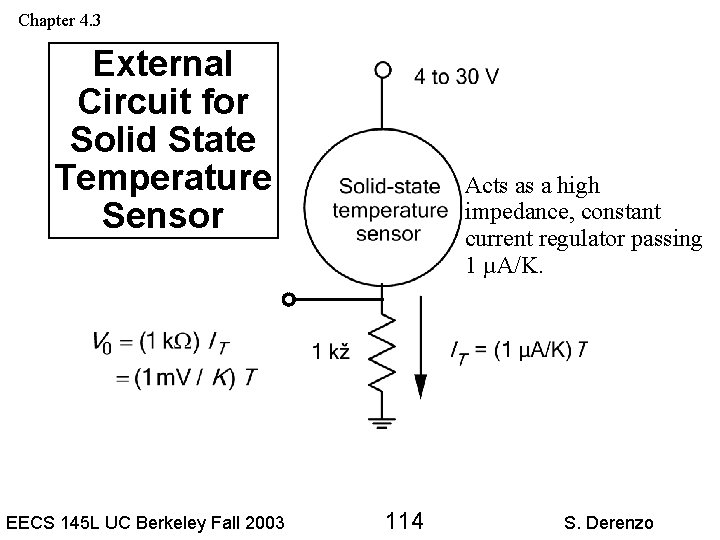
Chapter 4. 3 External Circuit for Solid State Temperature Sensor EECS 145 L UC Berkeley Fall 2003 Acts as a high impedance, constant current regulator passing 1 µA/K. 114 S. Derenzo