A introduction to subsurface energy extraction and storage
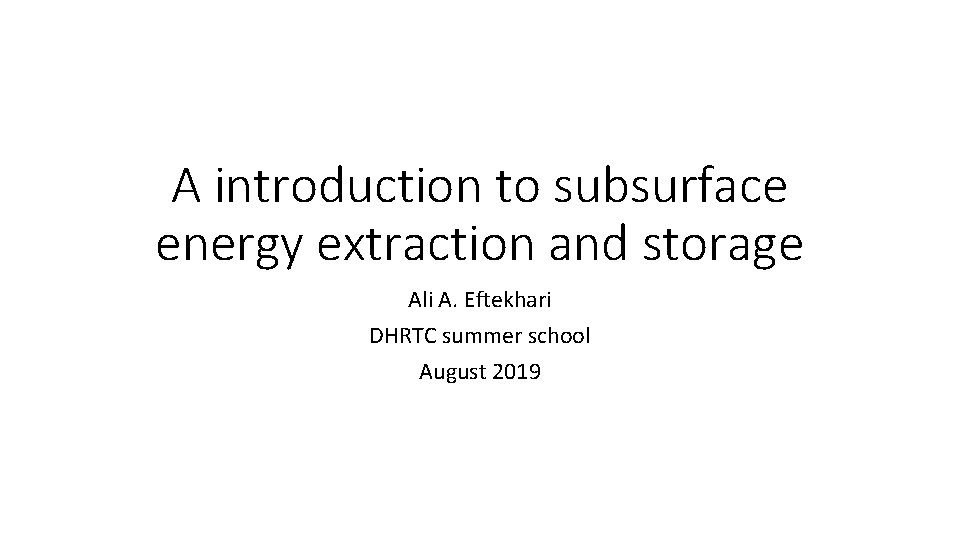
A introduction to subsurface energy extraction and storage Ali A. Eftekhari DHRTC summer school August 2019

Outline • Some thoughts on energy • A short history of thermodynamics • Case study: geothermal energy • Case study: water flooding • Case study: CO 2 storage Subsurface fluid and energy storage and extraction, 3 -week course, January 2020, Technical University of Denmark (5 credits)
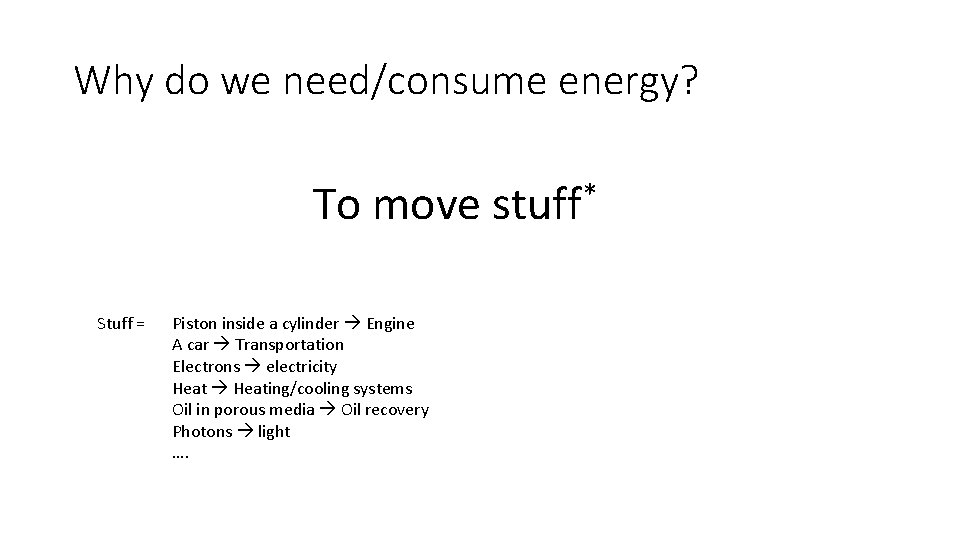
Why do we need/consume energy? To move stuff* Stuff = Piston inside a cylinder Engine A car Transportation Electrons electricity Heating/cooling systems Oil in porous media Oil recovery Photons light ….
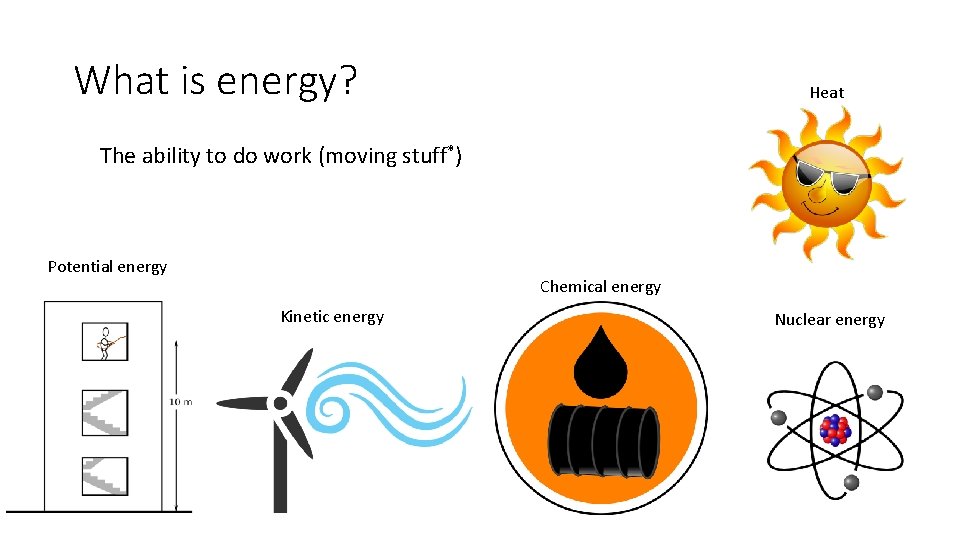
What is energy? Heat The ability to do work (moving stuff*) Potential energy Chemical energy Kinetic energy Nuclear energy
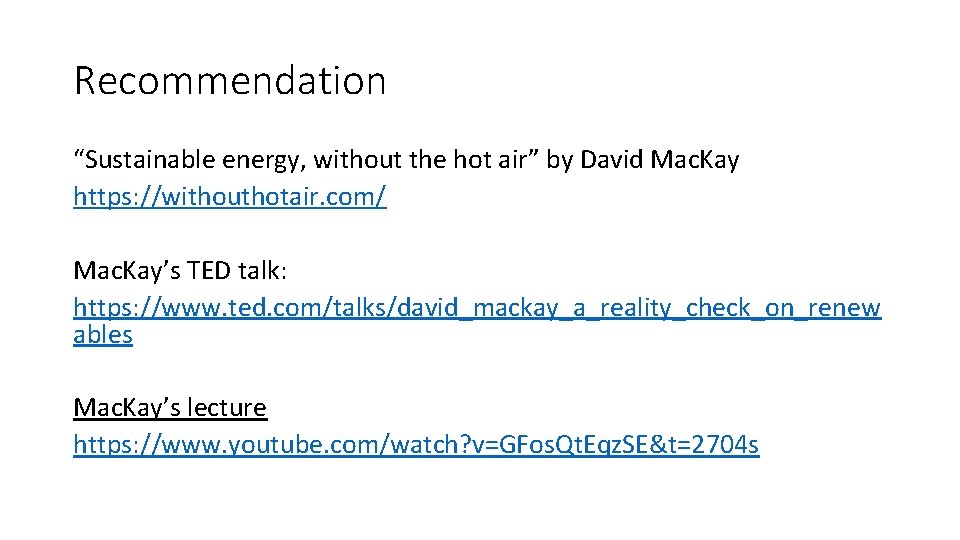
Recommendation “Sustainable energy, without the hot air” by David Mac. Kay https: //withouthotair. com/ Mac. Kay’s TED talk: https: //www. ted. com/talks/david_mackay_a_reality_check_on_renew ables Mac. Kay’s lecture https: //www. youtube. com/watch? v=GFos. Qt. Eqz. SE&t=2704 s

What is an energy resource? Anything that has a different pressure, temperature, velocity, altitude, and/or composition from its immediate surrounding Energy resource: Natural gas Composition: Methane: CH 4 Extracted energy Immediate surrounding: Atmosphere Composition: Nitrogen 78% Oxygen 21% Argon 0. 9% CO 2, CH 4, … <0. 1% Dead state
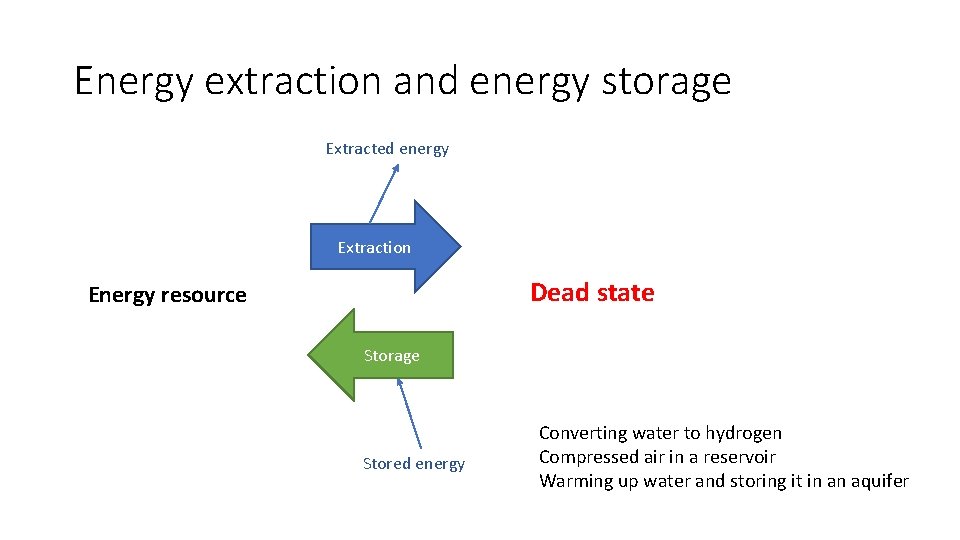
Energy extraction and energy storage Extracted energy Extraction Dead state Energy resource Storage Stored energy Converting water to hydrogen Compressed air in a reservoir Warming up water and storing it in an aquifer
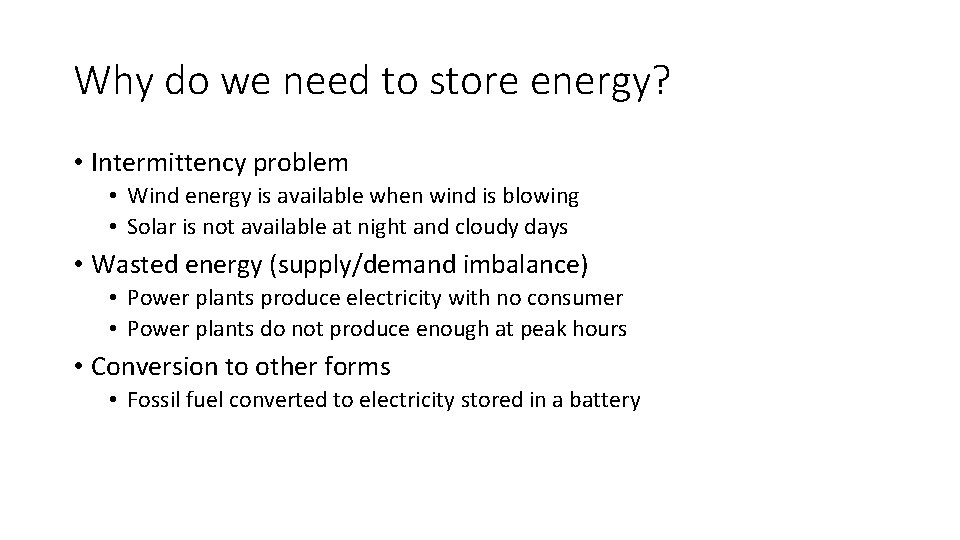
Why do we need to store energy? • Intermittency problem • Wind energy is available when wind is blowing • Solar is not available at night and cloudy days • Wasted energy (supply/demand imbalance) • Power plants produce electricity with no consumer • Power plants do not produce enough at peak hours • Conversion to other forms • Fossil fuel converted to electricity stored in a battery
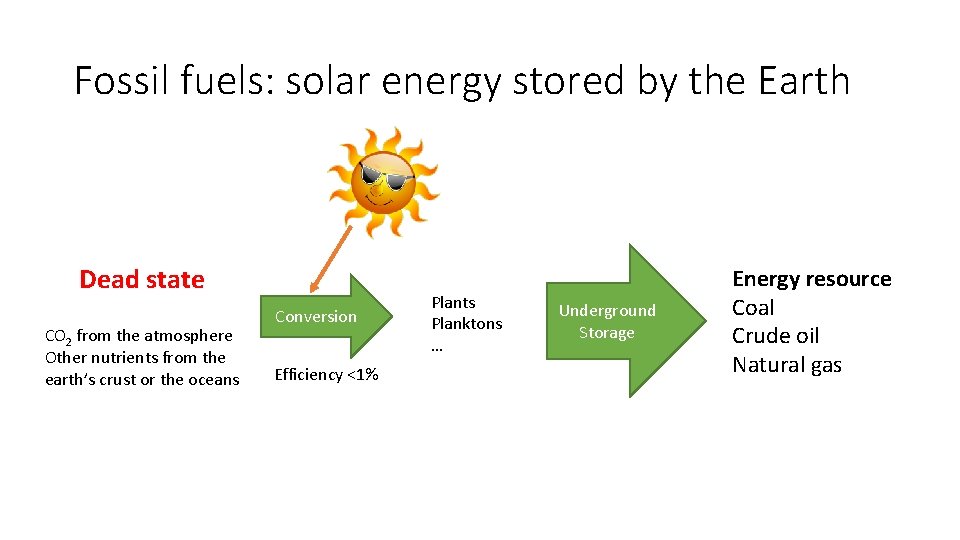
Fossil fuels: solar energy stored by the Earth Dead state CO 2 from the atmosphere Other nutrients from the earth’s crust or the oceans Conversion Efficiency <1% Plants Planktons … Underground Storage Energy resource Coal Crude oil Natural gas
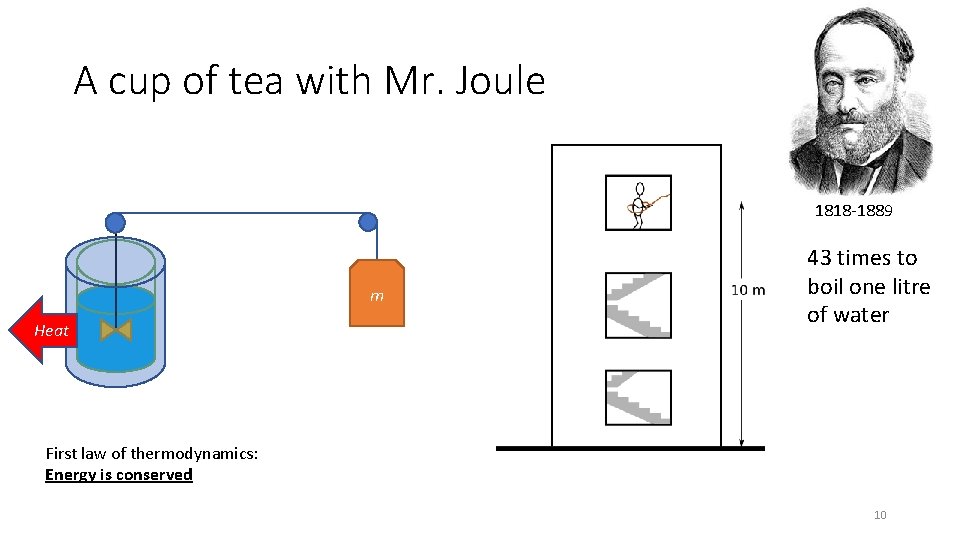
A cup of tea with Mr. Joule 1818 -1889 m Heat 43 times to boil one litre of water First law of thermodynamics: Energy is conserved 10
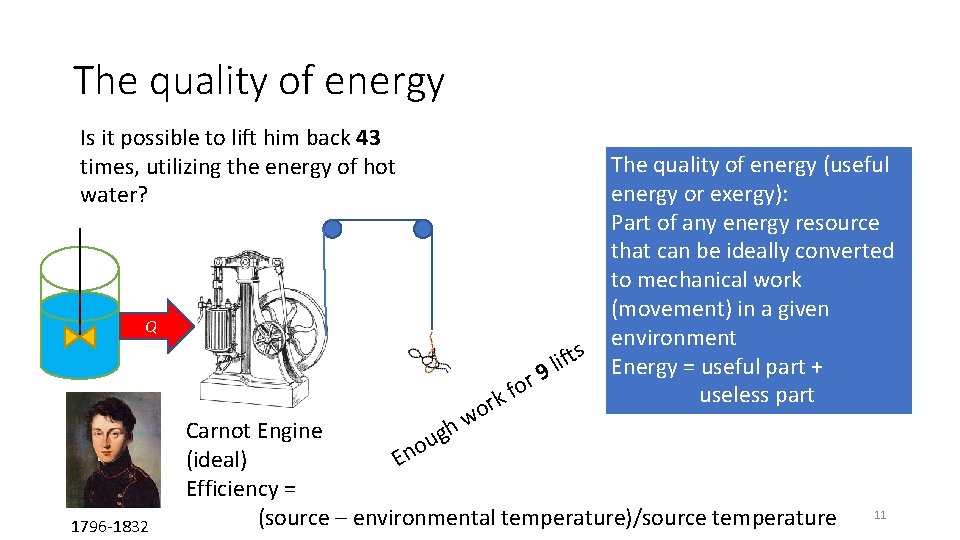
The quality of energy Is it possible to lift him back 43 times, utilizing the energy of hot water? Q m r o w r o f k 1796 -1832 s t f i 9 l The quality of energy (useful energy or exergy): Part of any energy resource that can be ideally converted to mechanical work (movement) in a given environment Energy = useful part + useless part Carnot Engine gh u o (ideal) En Efficiency = (source – environmental temperature)/source temperature 11
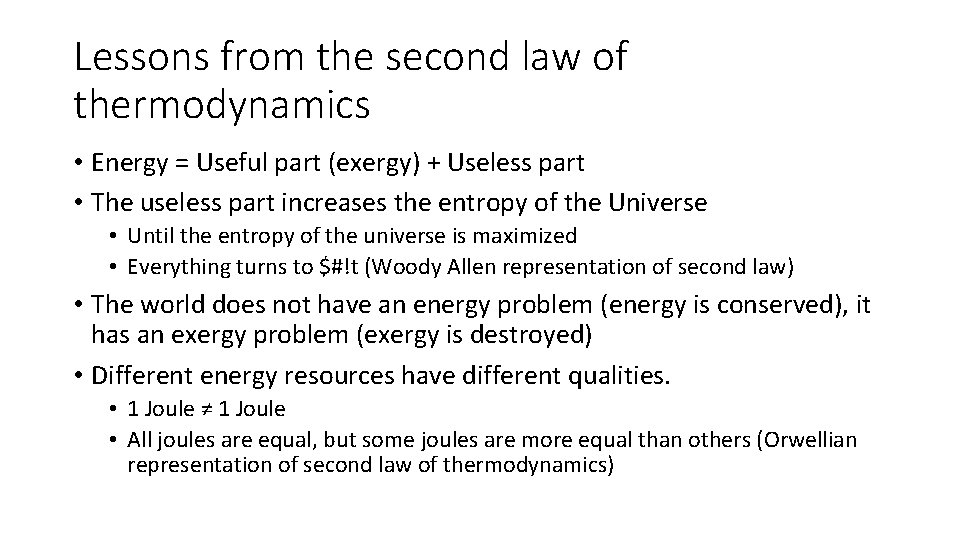
Lessons from the second law of thermodynamics • Energy = Useful part (exergy) + Useless part • The useless part increases the entropy of the Universe • Until the entropy of the universe is maximized • Everything turns to $#!t (Woody Allen representation of second law) • The world does not have an energy problem (energy is conserved), it has an exergy problem (exergy is destroyed) • Different energy resources have different qualities. • 1 Joule ≠ 1 Joule • All joules are equal, but some joules are more equal than others (Orwellian representation of second law of thermodynamics)
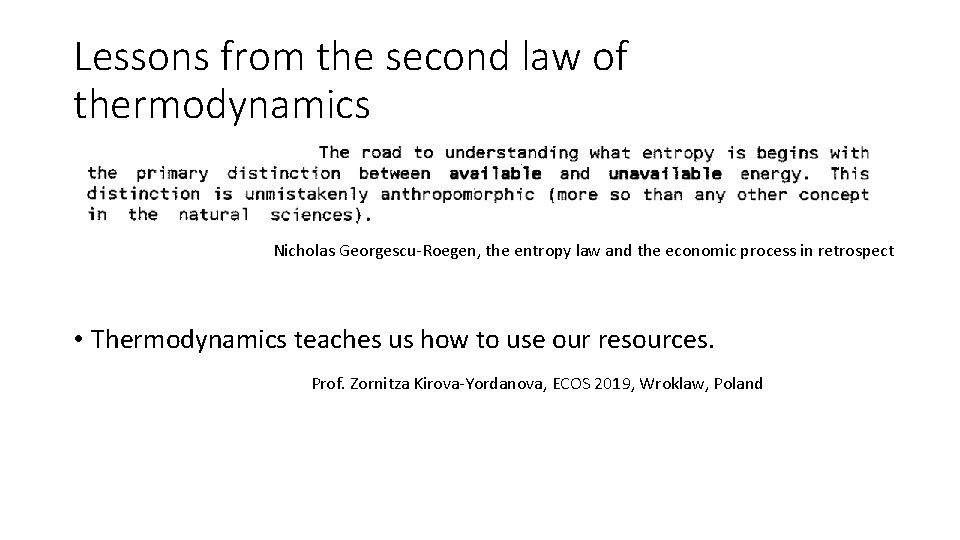
Lessons from the second law of thermodynamics Nicholas Georgescu-Roegen, the entropy law and the economic process in retrospect • Thermodynamics teaches us how to use our resources. Prof. Zornitza Kirova-Yordanova, ECOS 2019, Wroklaw, Poland

W • Maximum fraction of energy that can be converted to work Energy source • Practical quality • Maximum conversion with the Fossil fuels Hot water state of the art technology he re • Theoretical quality: W w e ca n ar e be Quality of energy: examples Theoretical quality Practical quality (second law of (state of the art thermodynamics) technology) ~ 100 % ~ 35 - 50 % 23 % ~ 4 % Steam 54 % ~ 10 % Wind 59. 3 % ~ 40 % Technological gap 14
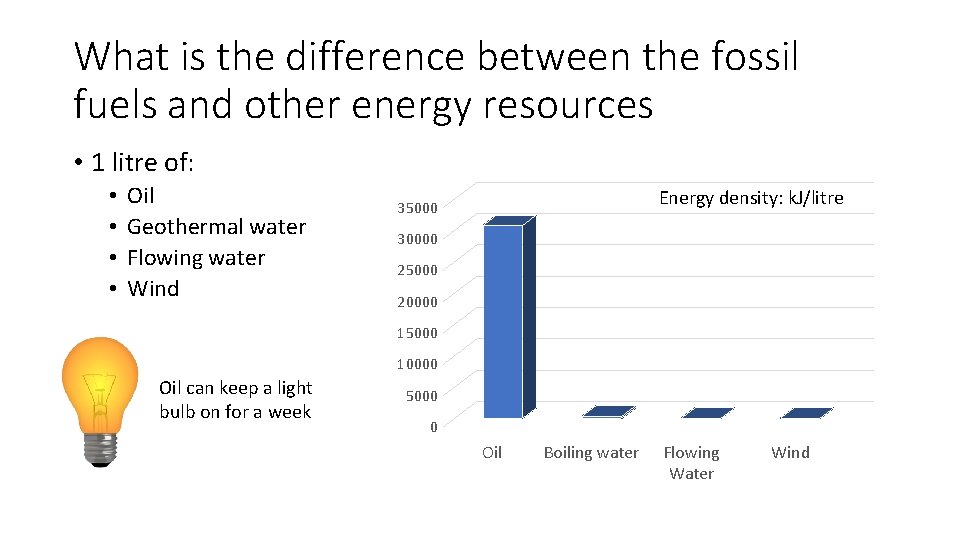
What is the difference between the fossil fuels and other energy resources • 1 litre of: • • Oil Geothermal water Flowing water Wind Energy density: k. J/litre 35000 30000 25000 20000 15000 10000 Oil can keep a light bulb on for a week 5000 0 Oil Boiling water Flowing Water Wind
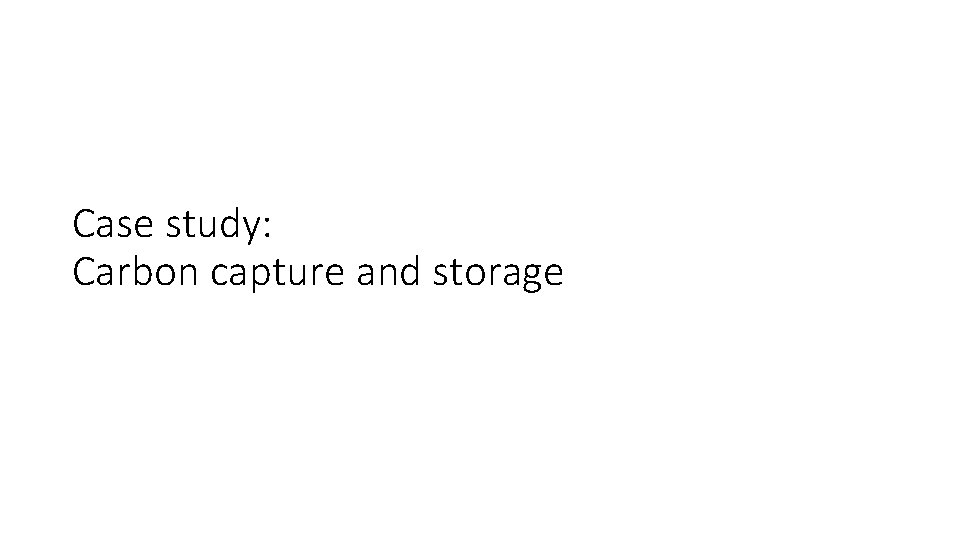
Case study: Carbon capture and storage
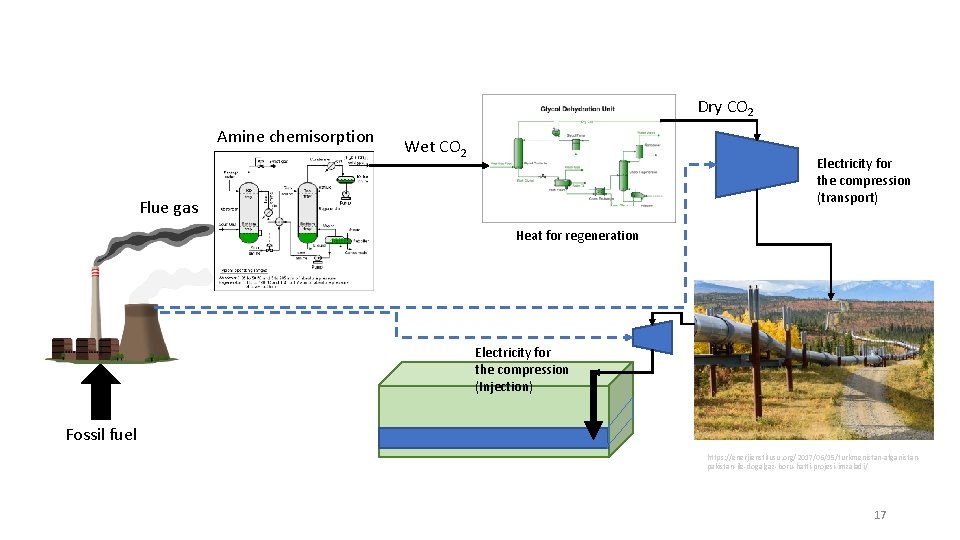
Dry CO 2 Amine chemisorption Wet CO 2 Electricity for the compression (transport) Flue gas Heat for regeneration Electricity for the compression (Injection) Fossil fuel https: //enerjienstitusu. org/2017/06/15/turkmenistan-afganistanpakistan-ile-dogalgaz-boru-hatti-projesi-imzaladi/ 17
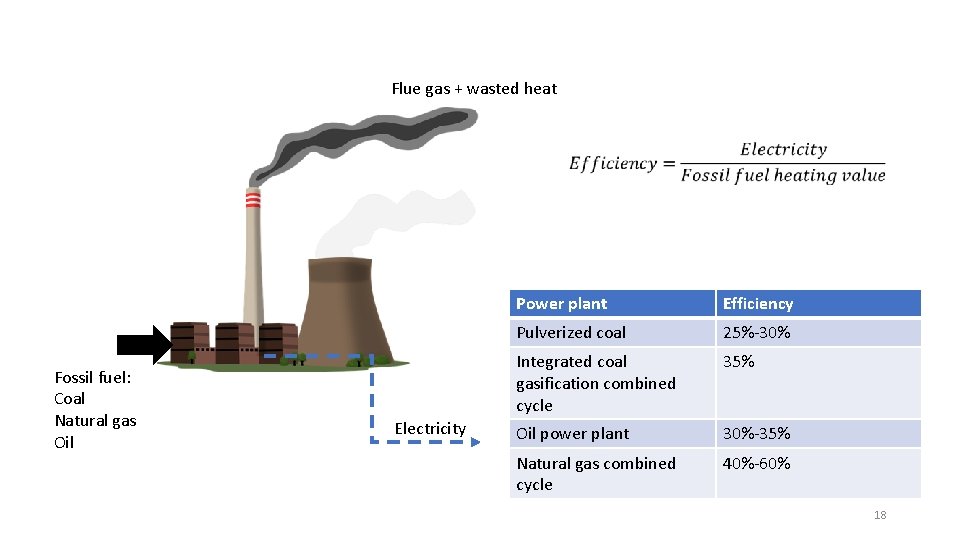
Flue gas + wasted heat Fossil fuel: Coal Natural gas Oil Electricity Power plant Efficiency Pulverized coal 25%-30% Integrated coal gasification combined cycle 35% Oil power plant 30%-35% Natural gas combined cycle 40%-60% 18
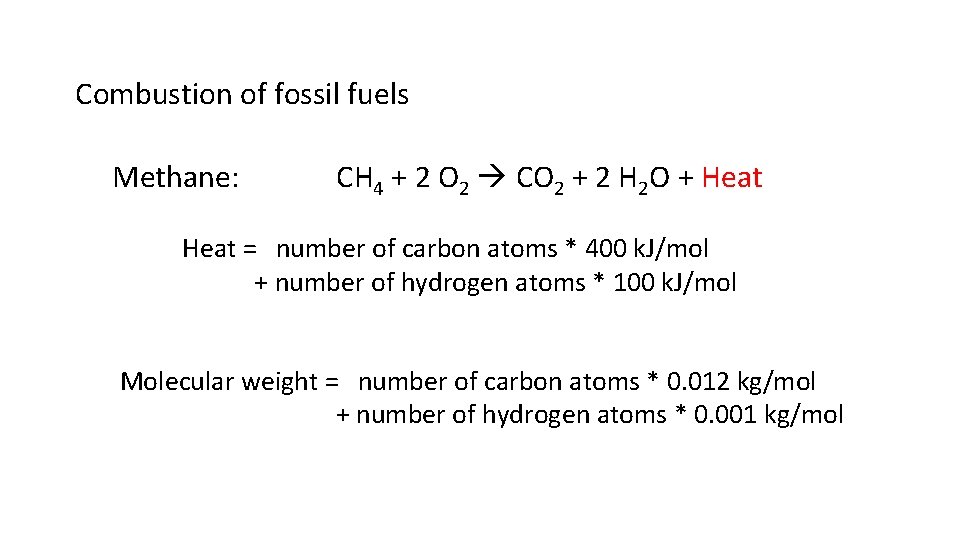
Combustion of fossil fuels Methane: CH 4 + 2 O 2 CO 2 + 2 H 2 O + Heat = number of carbon atoms * 400 k. J/mol + number of hydrogen atoms * 100 k. J/mol Molecular weight = number of carbon atoms * 0. 012 kg/mol + number of hydrogen atoms * 0. 001 kg/mol
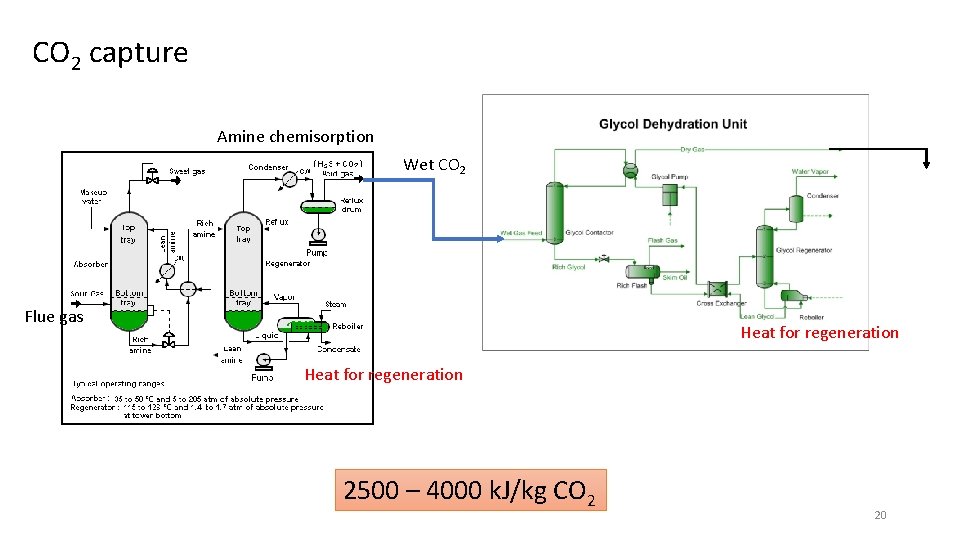
CO 2 capture Amine chemisorption Wet CO 2 Flue gas Heat for regeneration 2500 – 4000 k. J/kg CO 2 20
![Compressor Assuming isentropic compression: PVn=constant W = isentropic compression work [J/s] R = gas Compressor Assuming isentropic compression: PVn=constant W = isentropic compression work [J/s] R = gas](http://slidetodoc.com/presentation_image/1ff722cfb48342aa92ee2a44d900710e/image-21.jpg)
Compressor Assuming isentropic compression: PVn=constant W = isentropic compression work [J/s] R = gas constant 8. 314 J/(mol. K) T 1 = input temperature [K] n = cp/cv p 2 = output pressure [Pa] p 1 = input pressure [Pa] Z 1 = compressibility factor at input condition
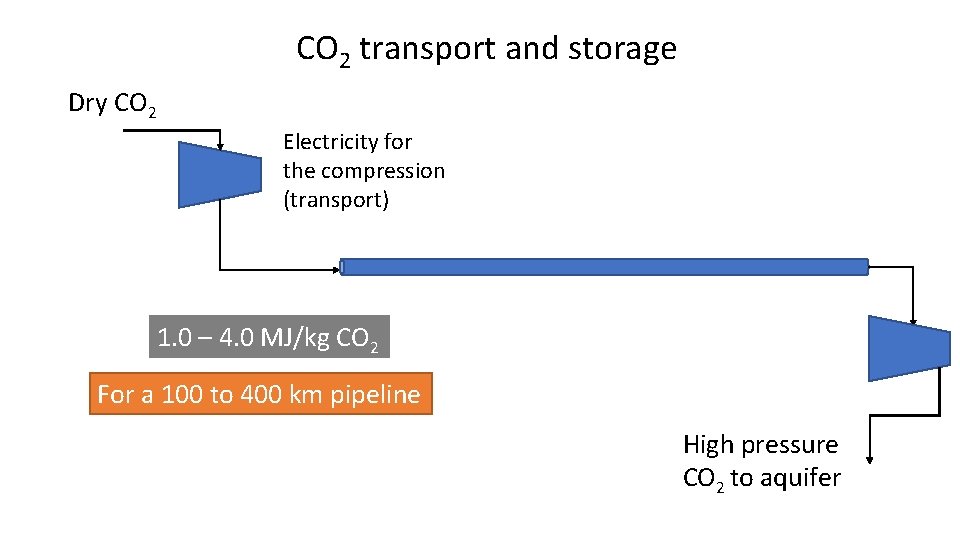
CO 2 transport and storage Dry CO 2 Electricity for the compression (transport) 1. 0 – 4. 0 MJ/kg CO 2 For a 100 to 400 km pipeline High pressure CO 2 to aquifer
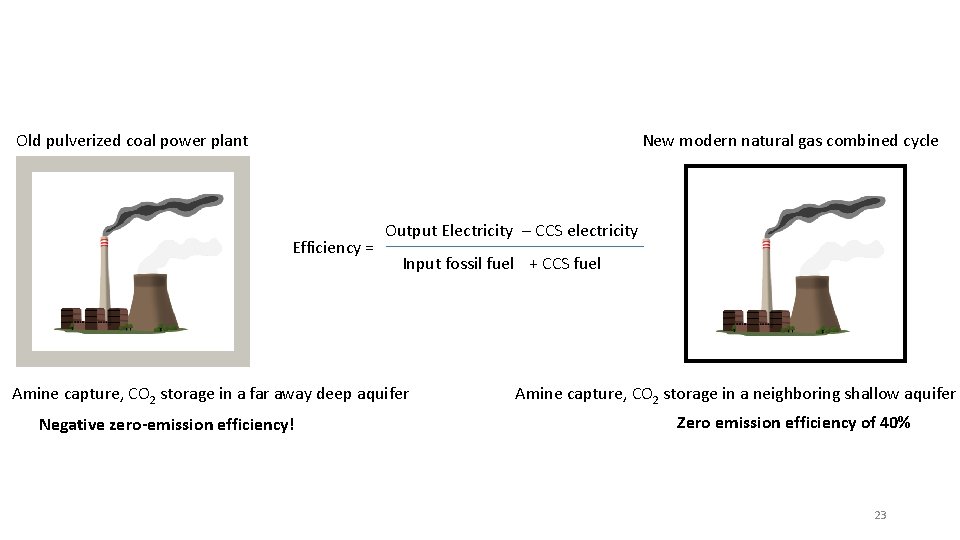
Old pulverized coal power plant New modern natural gas combined cycle Efficiency = Output Electricity – CCS electricity Input fossil fuel + CCS fuel Amine capture, CO 2 storage in a far away deep aquifer Negative zero-emission efficiency! Amine capture, CO 2 storage in a neighboring shallow aquifer Zero emission efficiency of 40% 23
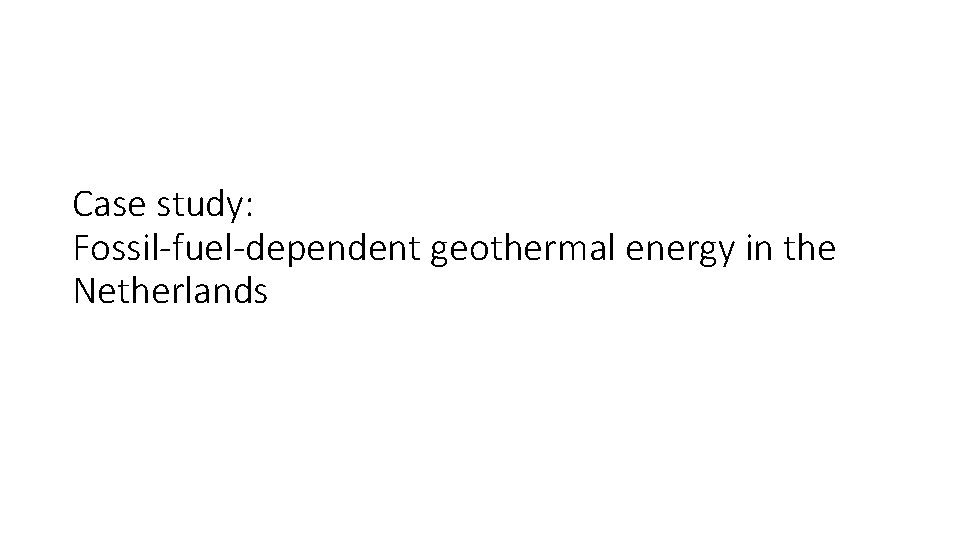
Case study: Fossil-fuel-dependent geothermal energy in the Netherlands
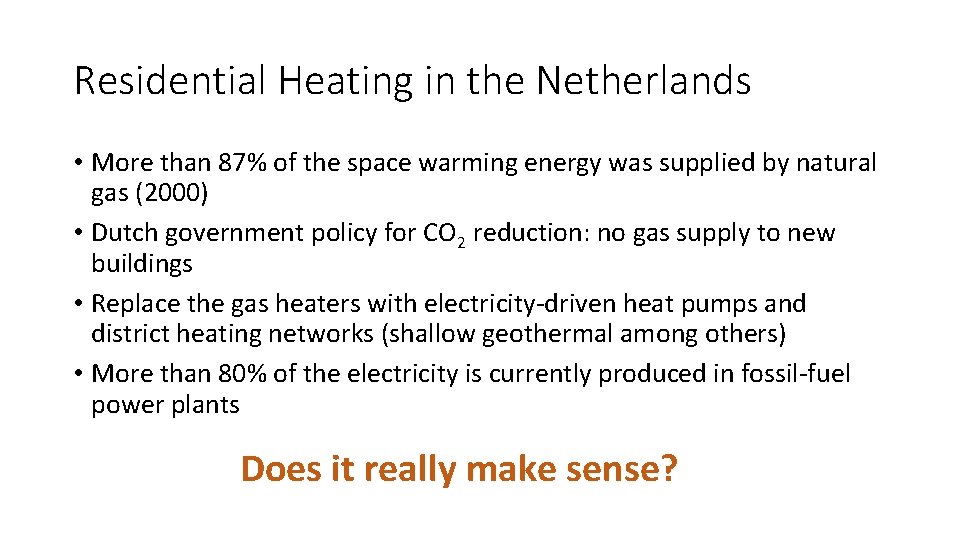
Residential Heating in the Netherlands • More than 87% of the space warming energy was supplied by natural gas (2000) • Dutch government policy for CO 2 reduction: no gas supply to new buildings • Replace the gas heaters with electricity-driven heat pumps and district heating networks (shallow geothermal among others) • More than 80% of the electricity is currently produced in fossil-fuel power plants Does it really make sense?
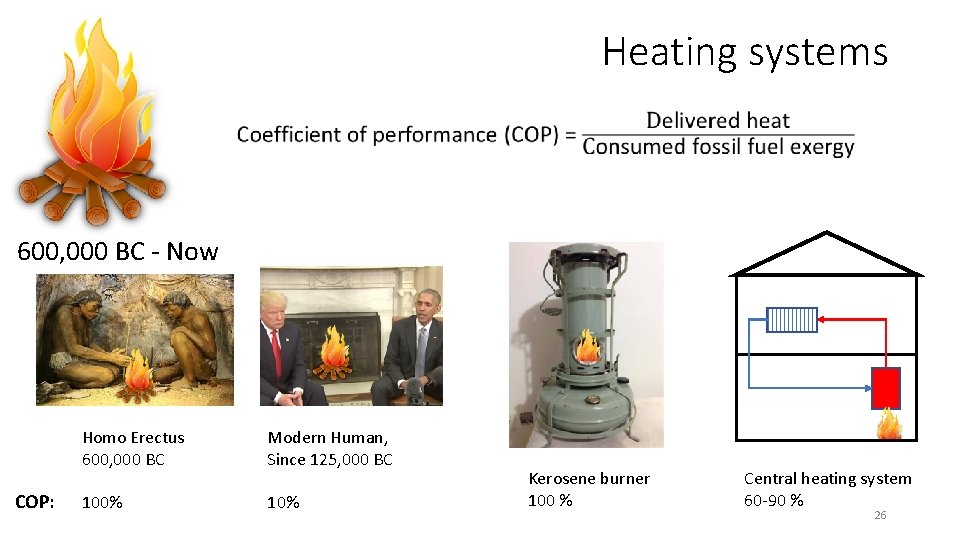
Heating systems 600, 000 BC - Now COP: Homo Erectus 600, 000 BC Modern Human, Since 125, 000 BC 100% 10% Kerosene burner 100 % Central heating system 60 -90 % 26
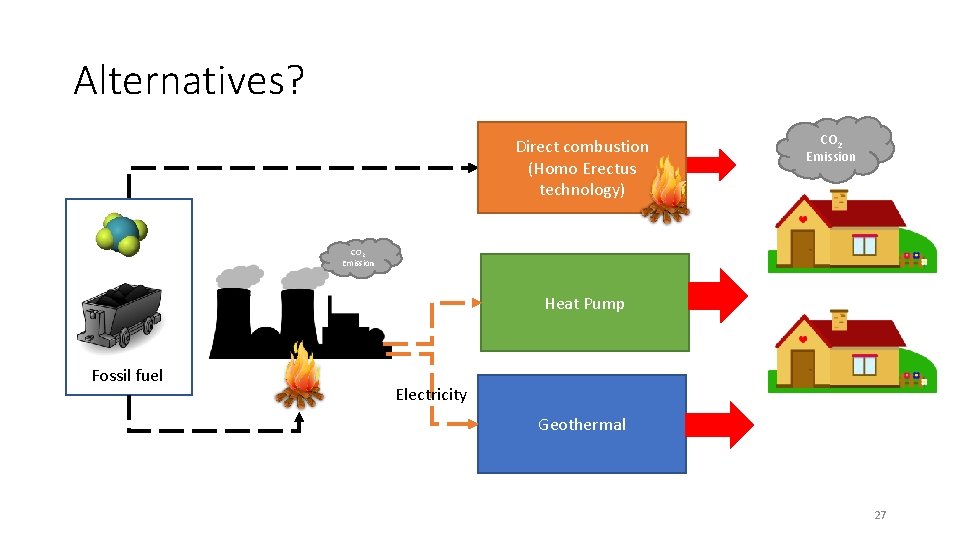
Alternatives? Direct combustion (Homo Erectus technology) CO 2 Emission Heat Pump Fossil fuel Electricity Geothermal 27
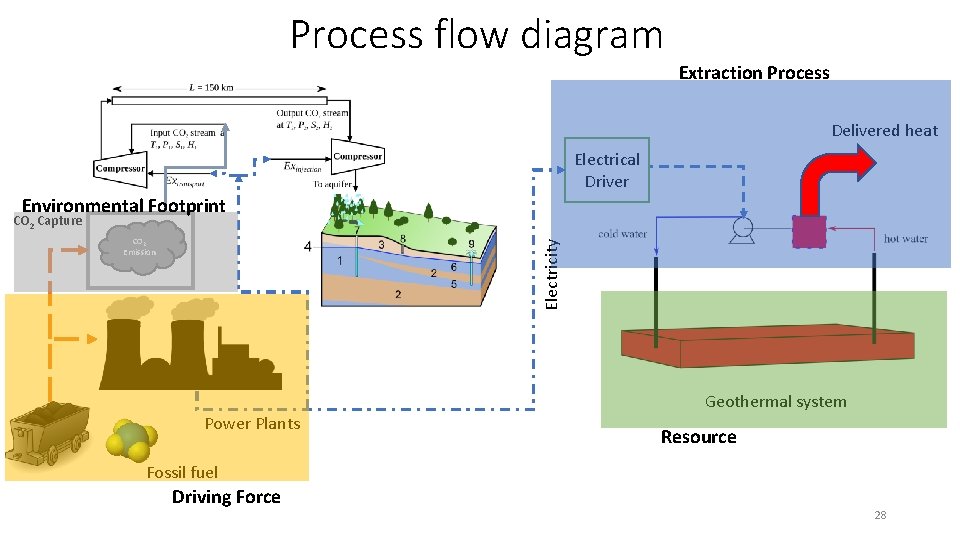
Process flow diagram Extraction Process Delivered heat Electrical Driver Environmental Footprint CO 2 Capture Electricity CO 2 Emission Geothermal system Power Plants Resource Fossil fuel Driving Force 28
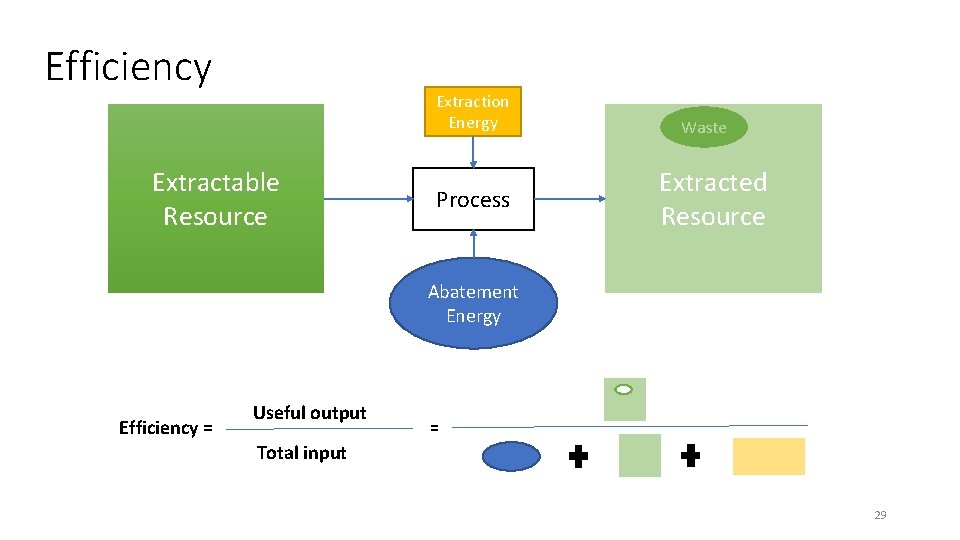
Efficiency Extraction Energy Extractable Resource Process Waste Extracted Resource Abatement Energy Efficiency = Useful output = Total input 29
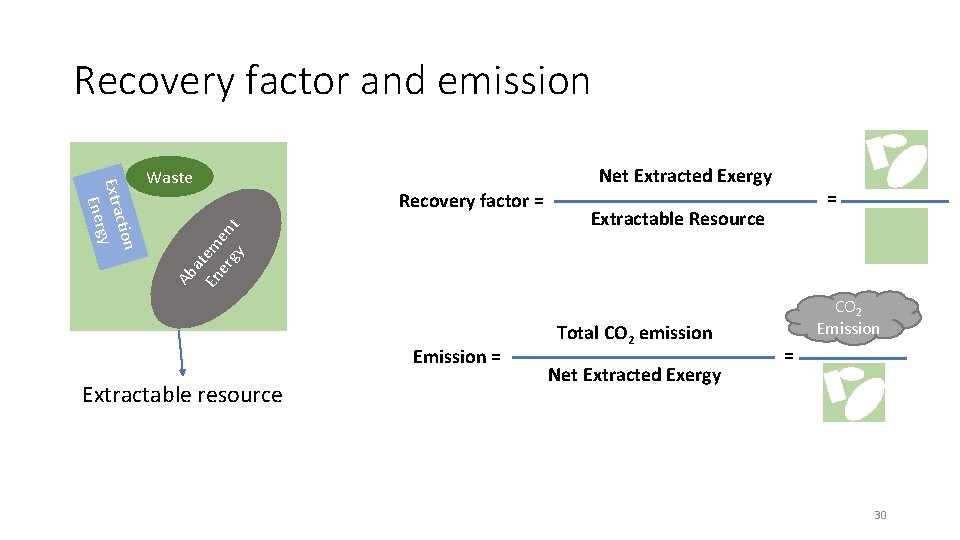
Recovery factor and emission Recovery factor = Ab at En em er en gy t ction Extra gy Ener Waste Emission = Extractable resource Net Extracted Exergy = Extractable Resource Total CO 2 emission Net Extracted Exergy CO 2 Emission = 30
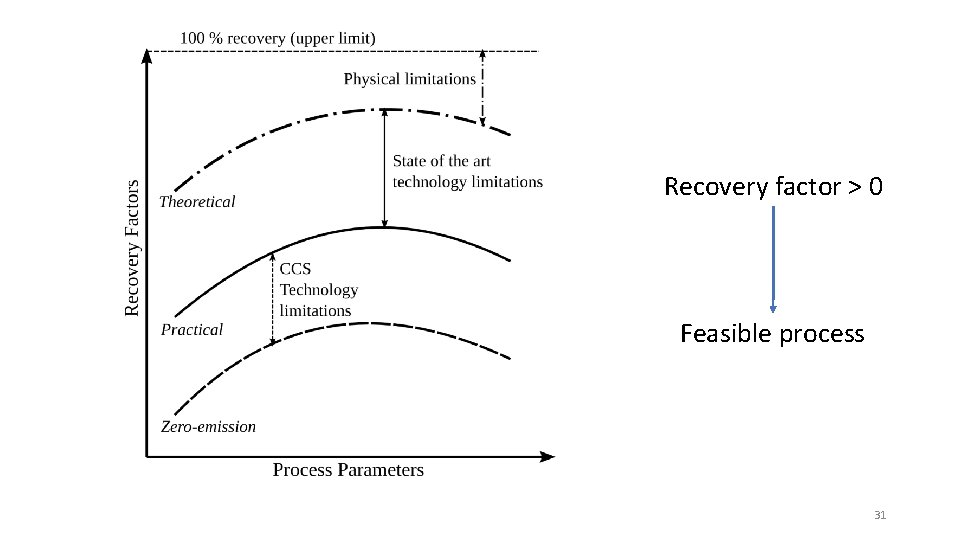
Recovery factor > 0 Feasible process 31
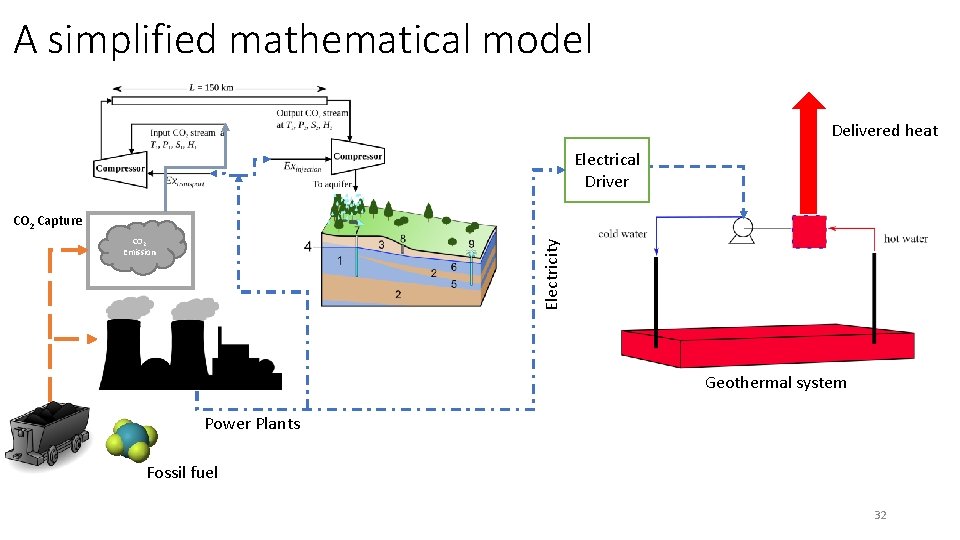
A simplified mathematical model Delivered heat Electrical Driver CO 2 Capture Electricity CO 2 Emission Geothermal system Power Plants Fossil fuel 32
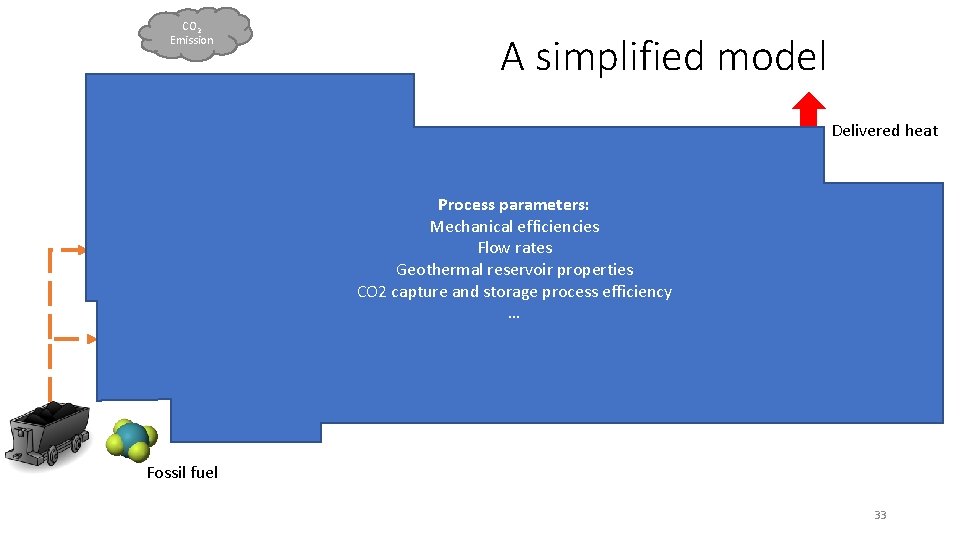
CO 2 Emission A simplified model Delivered heat Electrical Driver Process parameters: Mechanical efficiencies Flow rates Geothermal reservoir properties CO 2 capture and storage process efficiency … Electricity CO 2 Emission Geothermal system Power Plants Fossil fuel 33
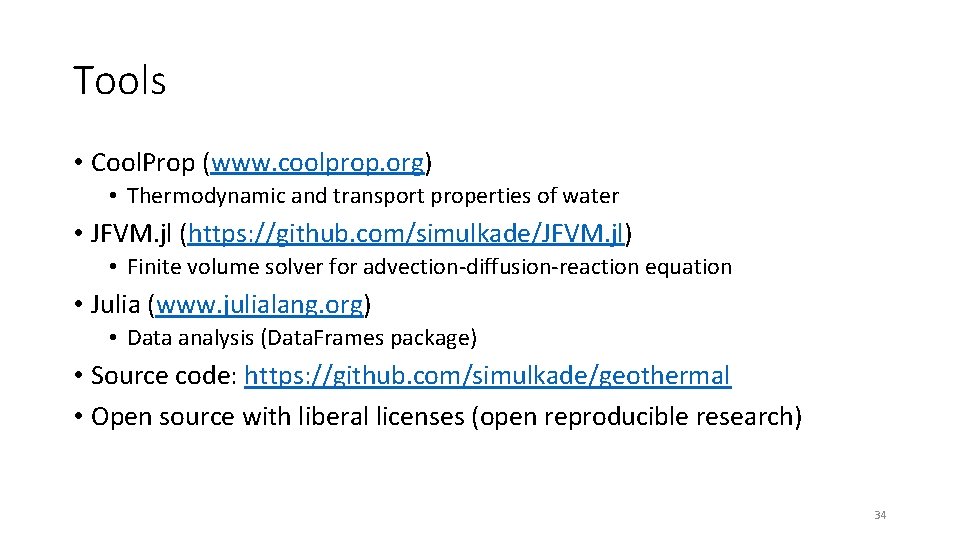
Tools • Cool. Prop (www. coolprop. org) • Thermodynamic and transport properties of water • JFVM. jl (https: //github. com/simulkade/JFVM. jl) • Finite volume solver for advection-diffusion-reaction equation • Julia (www. julialang. org) • Data analysis (Data. Frames package) • Source code: https: //github. com/simulkade/geothermal • Open source with liberal licenses (open reproducible research) 34
![Parameters Permeability range: 1 -1000 m. D Depth Reservoir temperature 25. 0 Porosity [%] Parameters Permeability range: 1 -1000 m. D Depth Reservoir temperature 25. 0 Porosity [%]](http://slidetodoc.com/presentation_image/1ff722cfb48342aa92ee2a44d900710e/image-35.jpg)
Parameters Permeability range: 1 -1000 m. D Depth Reservoir temperature 25. 0 Porosity [%] Source: http: //nlog. nl/reservoireigenschappen 30. 0 20. 0 15. 0 10. 0 5. 0 0. 00 1000. 00 2000. 00 3000. 00 Depth [m] 4000. 00 5000. 00 Bonte et al. , Netherlands Journal of Geosciences, 2012 35

Parameters 50 to 250 m 3/h Parameters • Reservoir permeability • Extraction rate • Well-spacing Well spacing 500 to 1000 m Permeability (acid stimulation, hydraulic fracturing) 0. 02 to 1. 0 Darcy 36
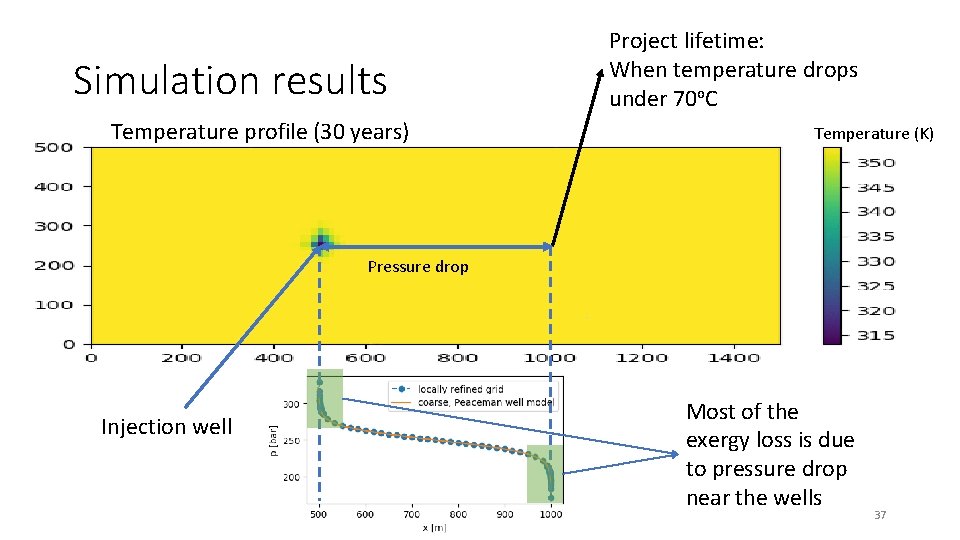
Simulation results Temperature profile (30 years) Project lifetime: When temperature drops under 70 o. C Temperature (K) Pressure drop Injection well Most of the exergy loss is due to pressure drop near the wells 37
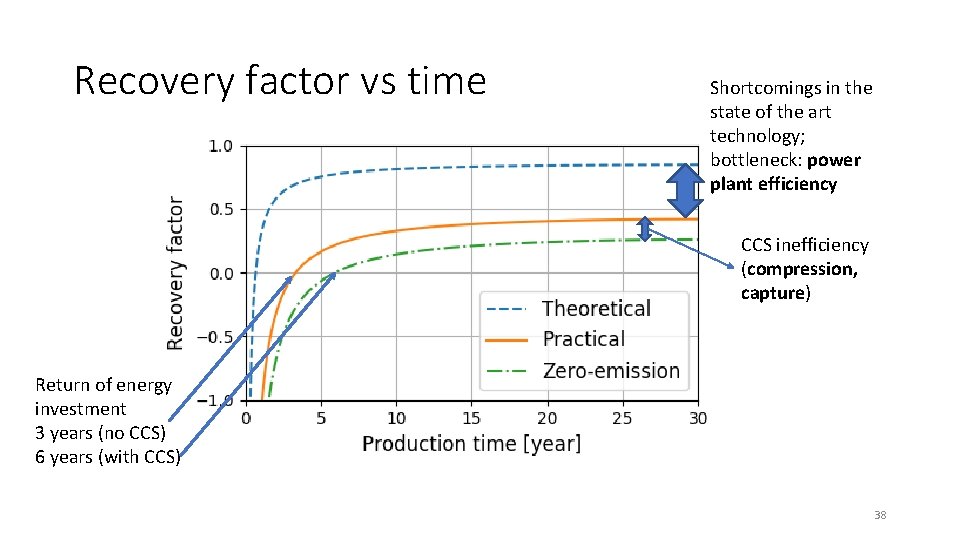
Recovery factor vs time Shortcomings in the state of the art technology; bottleneck: power plant efficiency CCS inefficiency (compression, capture) Return of energy investment 3 years (no CCS) 6 years (with CCS) 38

Choosing the optimum scenarios • Project life-time > 30 years • Zero-emission recovery factor > 0. 0 • Carbon emission < natural gas carbon emission • 30 cases out of 150 cases met the above criteria • High permeability • Low flow rate 39
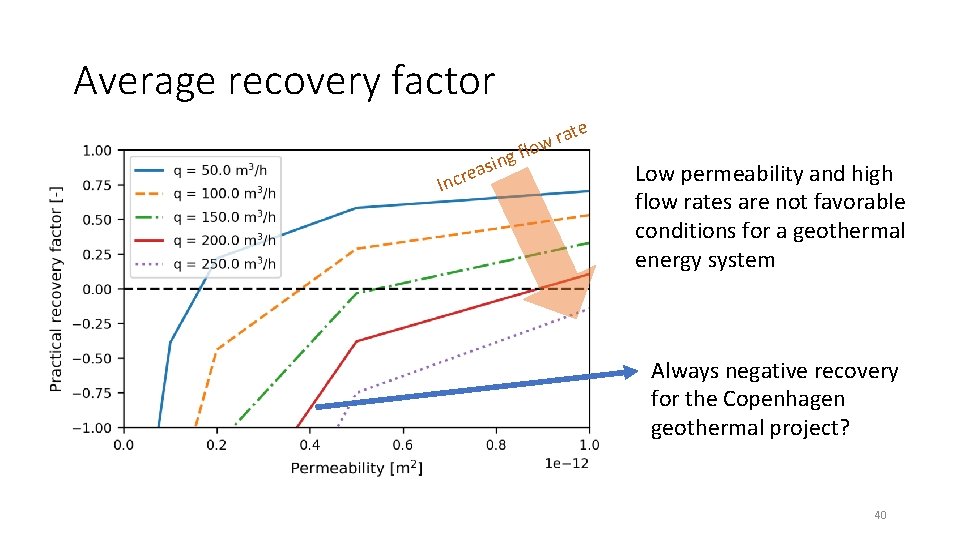
Average recovery factor fl I g n i s a ncre e rat w o Low permeability and high flow rates are not favorable conditions for a geothermal energy system Always negative recovery for the Copenhagen geothermal project? 40
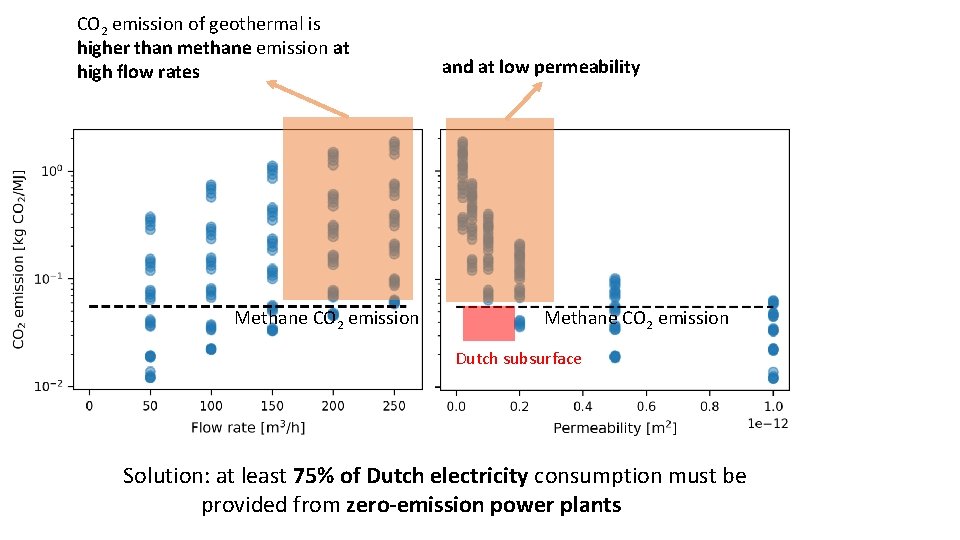
CO 2 emission of geothermal is higher than methane emission at high flow rates Methane CO 2 emission and at low permeability Methane CO 2 emission Dutch subsurface Solution: at least 75% of Dutch electricity consumption must be provided from zero-emission power plants
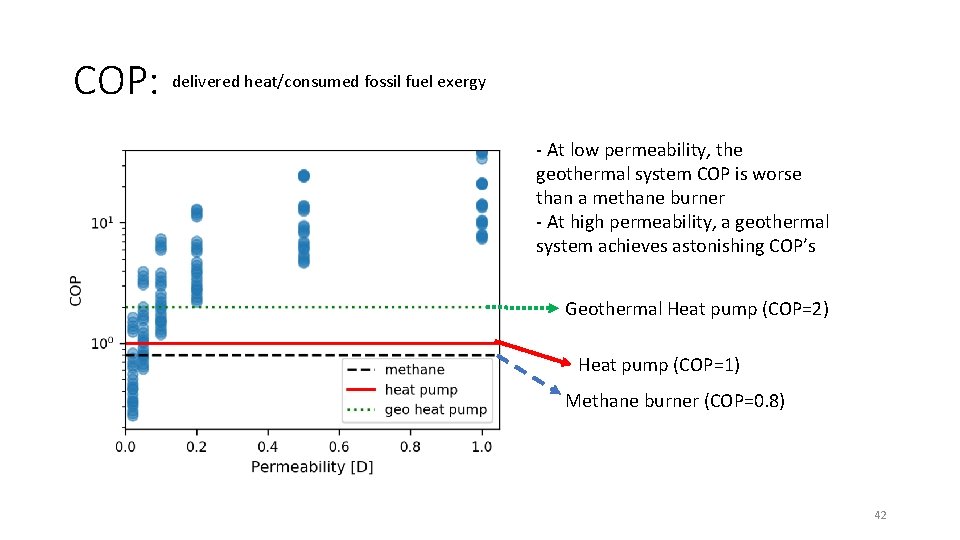
COP: delivered heat/consumed fossil fuel exergy - At low permeability, the geothermal system COP is worse than a methane burner - At high permeability, a geothermal system achieves astonishing COP’s Geothermal Heat pump (COP=2) Heat pump (COP=1) Methane burner (COP=0. 8) 42

Case study: water flooding Reference: Farajzedeh et al. , Journal of Cleaner Production 235 (2019) 812 e 821
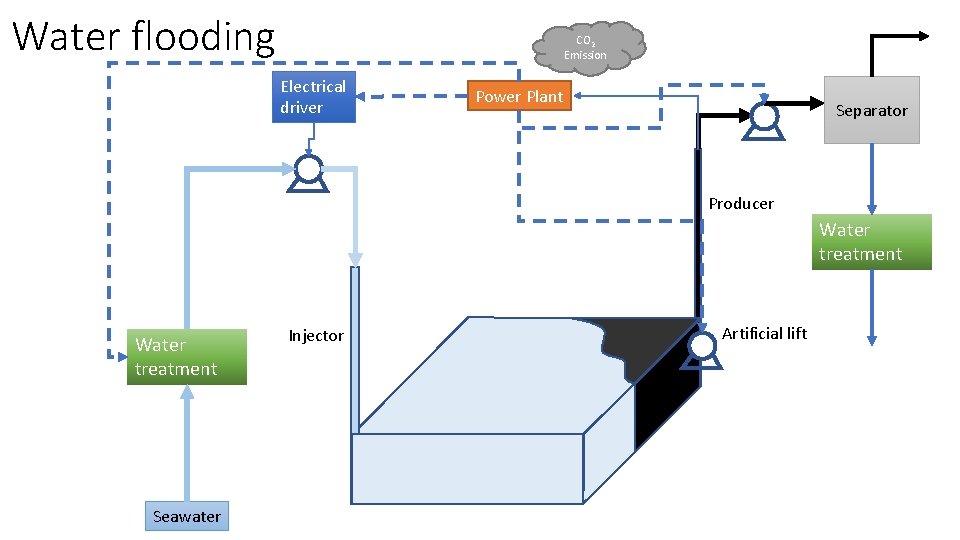
Water flooding CO 2 Emission Electrical driver Power Plant Separator Producer Water treatment Seawater Injector Artificial lift

Recovery factor Simplified for this case:
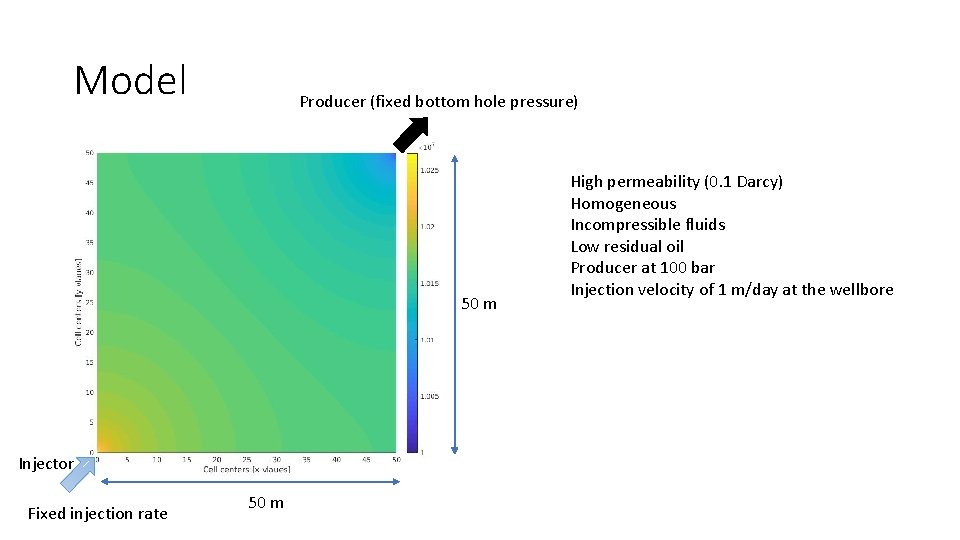
Model Producer (fixed bottom hole pressure) 50 m Injector Fixed injection rate 50 m High permeability (0. 1 Darcy) Homogeneous Incompressible fluids Low residual oil Producer at 100 bar Injection velocity of 1 m/day at the wellbore
![Pumping energy Pout: input pressure [Pa] Q: flow rate [m 3/s] Pin: input pressure Pumping energy Pout: input pressure [Pa] Q: flow rate [m 3/s] Pin: input pressure](http://slidetodoc.com/presentation_image/1ff722cfb48342aa92ee2a44d900710e/image-47.jpg)
Pumping energy Pout: input pressure [Pa] Q: flow rate [m 3/s] Pin: input pressure [Pa] 70 -80% 90% 25 -40%
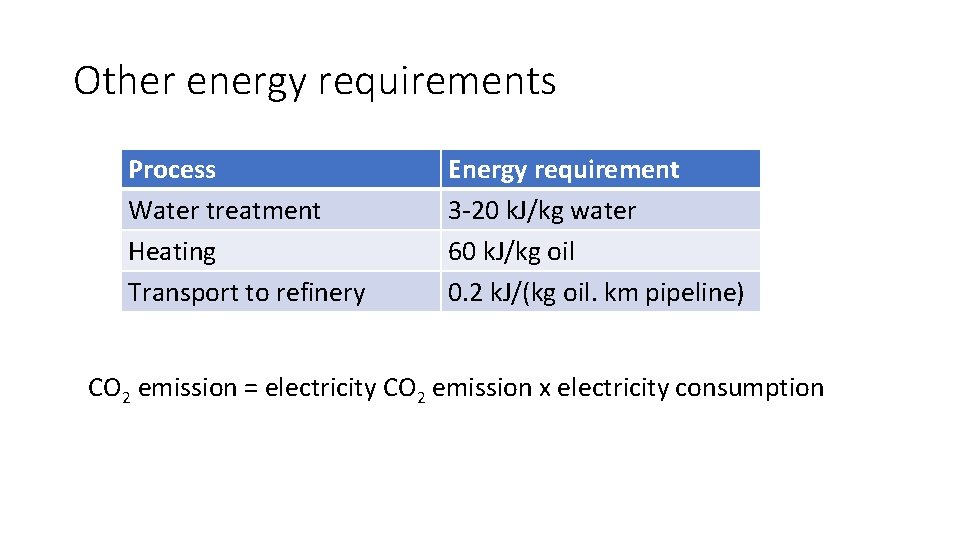
Other energy requirements Process Water treatment Heating Transport to refinery Energy requirement 3 -20 k. J/kg water 60 k. J/kg oil 0. 2 k. J/(kg oil. km pipeline) CO 2 emission = electricity CO 2 emission x electricity consumption
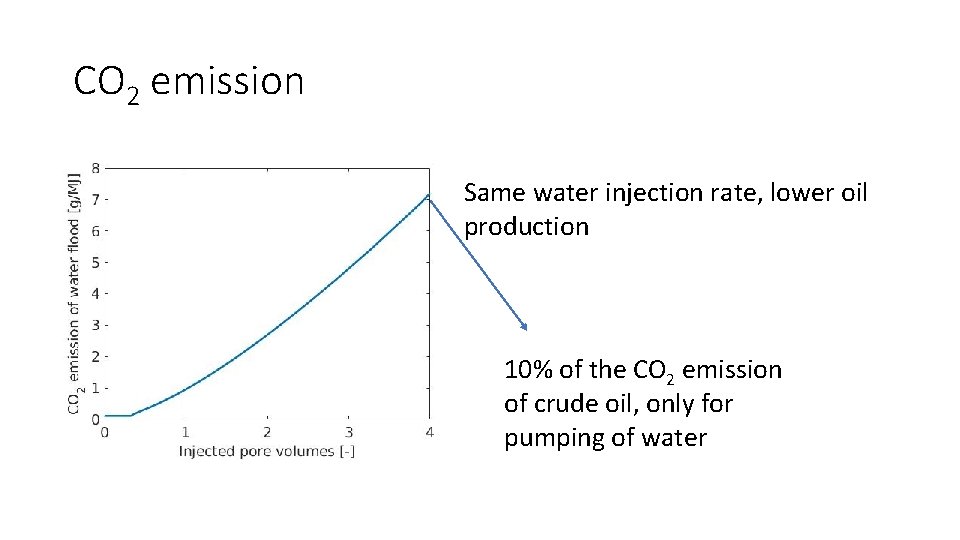
CO 2 emission Same water injection rate, lower oil production 10% of the CO 2 emission of crude oil, only for pumping of water

Exergetic recovery factor Technology limitations (room for improvement) CCS technology limitations
- Slides: 50